Cardiac Muscle: The Heart as a Pump and Cardiac Function PDF
Document Details
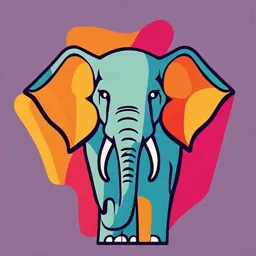
Uploaded by DelightfulNashville
LECOM-Bradenton Master of Medical Sciences
Santiago Lorenzo, Ph.D.
Tags
Summary
This document is a set of lecture notes on cardiac muscle, the heart as a pump, and cardiac function. It includes diagrams and definitions related to the heart and its functionality.
Full Transcript
Cardiac Muscle; The Heart as a Pump and Cardiac Function LECOM-Bradenton Masters of Medical Sciences Medical Physiology Santiago Lorenzo, Ph.D. Santiago Lorenzo, Ph.D. Email: [email protected] Phone #: 941-782-0690 Office location: #381 (COM) Appointm...
Cardiac Muscle; The Heart as a Pump and Cardiac Function LECOM-Bradenton Masters of Medical Sciences Medical Physiology Santiago Lorenzo, Ph.D. Santiago Lorenzo, Ph.D. Email: [email protected] Phone #: 941-782-0690 Office location: #381 (COM) Appointments: Email me! Heart location 3 Heart Location 4 Associated structures 5 The Pericardium Pericardium—double-walled sac that encloses the heart (isolates heart from other organs) Allows heart to beat without friction, provides room to expand, yet resists excessive expansion Anchored to diaphragm inferiorly and sternum anteriorly 6 The Pericardium Parietal pericardium (pericardial sac)—outer wall of sac Superficial fibrous layer of dense irregular connective tissue Deep, thin serous layer Visceral pericardium (epicardium)—heart covering Serous lining of sac turns inward at base of heart to cover the heart surface Pericardial cavity—space inside the pericardial sac filled with 5 to 30 mL of pericardial fluid 7 Pericardial cavity Pericardial sac: Fibrous layer Serous layer Epicardium 8 Pericarditis Inflammation of pericardium Causes Infection (viral/bacterial) Heart attack Trauma to chest Swelling/inflammation of heart muscle Radiation to chest Painful Treatment: NSAIDs (ibuprofen to ↓swelling) Antibiotics Pericardiocentesis 9 What’s wrong with these pictures? Cardiac Tamponade Pericardiocentesis 10 Pericardiocentesis: Treatment for Cardiac Tamponade 11 Layers of the Heart Wall 12 Structure of Cardiac Muscle Cardiac muscle cell - Cardiac fiber - Myocardial cell - Myocardial fiber Specialization of Cardiac Muscle Cells: 1. The cardiac fibers are joined together in a branching network with a single nucleus per cell 2. Cardiac cells are smaller than skeletal muscle fibers and contain an abundance of mitochondria and myoglobin 3. Adjacent cells are joined end to end by “intercalated discs” Desmosomes: mechanically holds cells together. Gap Junctions: areas of low electrical resistance that allow the spread of action potentials. 4. The heart has a specialized “conduction system” that allows an impulse spontaneously generated in one part of the heart to spread throughout the heart 13 Function of Cardiac Muscle Specialization of Cardiac Muscle Cells: 1. Heart operates on its own 2. Each contraction is “all or none” - all the cardiac muscle cells will contract during each heart beat as the electrical impulse spreads to all cells that are joined by gap junctions - it is a “functional syncytium” 3. The atria and ventricles each form a functional syncytium and thus contract as separate units 4. Fibrous insulator exists between atrium and ventricle (why?) 14 Action Potentials in Cardiac Muscle Resting membrane potential of cardiac muscle is -85 to -95 millivolts Action potential is 105 millivolts Plateau lasts ~0.2-0.3 sec in ventricular muscle (much longer than skeletal muscle) 15 Ventricular Muscle Action Potential ++ K+ Channels Ca Channels K+ Channels Open Open More Open More Slow Ca++ Channels Open 1 +20 Membrane Potential 2 0 -20 3 (mV) -40 0 -60 4 -80 -100 Fast Na+ Channels Open 0 1 2 3 4 phase 0- Fast Na+ channels open then slow Ca ++ channels phase 1- K+ channels open Seconds ++ phase 2- Ca channels open more phase 3- K+ channels open more phase 4- Resting membrane potential 16 Short Discussion The refractory period in cardiac cells is about 250ms. In other cells ( like muscular system) is just 2-3ms During this time cardiac muscle cannot be re-excited Why is this important in cardiac contractile cells? Importance of Refractory Period 18 Excitation-Contraction Coupling in Cardiac Muscle * 19 Without “tetani” or fiber recruitment, how can the strength of contraction be graded or controlled? 20 Phospholamban regulates Ca2+-ATPase activity * PHOSPHOLAMBAN How would phospholamban affect cardiac contractions? 21 Structure-Function of Cardiac Muscle Like Skeletal Muscle 1. Cardiac muscle is striated (same arrangement of actin and myosin) 2. Actin contains troponin and tropomyosin, where Ca2+ acts to turn on cross-bridge activity Like Smooth Muscle 1. Ca2+ enters the cytosol from both the ECF and the sarcoplasmic reticulum 2. The Ca2+ entry from the ECF triggers Ca2+ release from the sarcoplasmic reticulum (Ca2+-induced Ca2+ release) 3. Cardiac cells are interconnected by “gap junctions” allowing the spread of action potentials through the heart 4. Some cardiac cells display pacemaker activity (i.e., exhibit their own action potentials without external influence) 22 Purple Foxglove Digitalis purpurea d 23 Digitoxin inhibits Na + transport DIGITOXIN * How would digitoxin affect cardiac contractions? 24 Group Discussion - Digitoxin What are the two sources of Ca+2 for cardiac contraction? Which is most important in affecting contractility? Where does the Ca+2 go after a contraction? What affect should digitoxin have on Ca+2 cycling and Ca+2 stores in cardiac muscle? How would digitoxin affect cardiac contractions? 25 Cardiac Function – What is measured? Stroke Volume: Volume of blood pumped out of the ventricle during a single systole Stroke volume = End-Diastolic Volume – End-Systolic Volume expressed as ml of blood Cardiac Output (Qc): Volume of blood pumped from the ventricle in one minute Qc= Heart Rate x Stroke Volume expressed as liters of blood per minute (so need to convert some units) Ejection Fraction (EF) = Stroke Volume/End-Diastolic Volume expressed as % (so multiple by 100) 26 Example End- - = End-Diastolic Volume Systolic Stroke Volume Volume 140 ml 70 ml 70 ml If the heart does this 70 times per minute… Qc Heart Rate 70 bpm x Stroke Volume 70 ml = 4900 ml/min or 4.9 l/min Ejection Fraction? 50% 27 Ejection Fraction End diastolic volume = 120 ml End systolic volume = 50 ml Ejection volume (stroke volume) = 70 ml Ejection fraction = 70 ml/120 ml = 58% (normally 60%) If heart rate (HR) is 70 beats/minute, what is cardiac output? Cardiac output = HR * stroke volume = 70/min. * 70 ml = 4900 ml/min. 28 Autonomic Effects on Heart Sympathetic stimulation causes increased HR + increased contractility with HR = 180-200 bpm and C.O. = 15-20 L/min. Very fast heart rates (HR>200bpm) can decrease Qc because there is not enough time for heart to fill during diastole. Parasympathetic stimulation decreases HR markedly and decreases cardiac contractility slightly. Vagal fibers go mainly to atria. 29 Effect of Sympathetic and Parasympathetic Stimulation on Cardiac Output Maximum 25 sympathetic stimulation 20 Cardiac Output (L/min) Normal 15 sympathetic stimulation Zero sympathetic stimulation 10 (Parasympathetic stimulation) 5 0 -4 0 +4 +8 Right Atrial Pressure (mmHg) 30 Cardiac Electrophysiology LECOM-Bradenton Masters of Medical Sciences Medical Physiology Santiago Lorenzo, Ph.D. Conducting System of the Heart Bundle of His 32 Cardiac Electrical Pathway Begins in the sinoatrial (S-A) node Internodal pathway to atrioventricular (A-V) node Impulse delayed in A-V node and bundle (allows atria to contract before ventricles) A-V bundle takes impulse into ventricles Left and right bundles of Purkinje fibers take impulses to all parts of ventricles 33 Electrical Activity of the Heart 99% of cardiac cells are “contractile cells” that propagate action potentials and contract/generate force The remainder of the cells are “autorhythmic cells” Some have minimal or absent sarcomeres – primary role is NOT force generation Propagate action potentials Spontaneously initiate action potentials - display “pacemaker activity” Sinoatrial Node: Pacemaker in right atrium Atrioventricular Node: At junction of atria and ventricles Bundle of His: Has 2 branches in the interventricular septum 34 Autorhythmic cells (Sinoatrial Node) Sinoatrial (SA) node acts as pacemaker. Membrane leaks Na+ and membrane potential is -55 to - 60mV When membrane potential reaches -40 mV, slow Ca++ channels open causing action potential After 100-150 msec Ca++ channels close and K+ channels open more thus returning membrane potential to - 55mV 35 Rhythmical Discharge of Sinus Nodal Fiber Sinus Nodal Slow Ca++ Fiber Channels Open K+ Channels Membrane Potential (mV) +20 Open more 0 Threshold -20 -40 -60 -80 Na+ Leak -100 0 1 2 3 4 Seconds 36 Membrane Potentials: Contractile cells Autorhythmic cells 0 3 4 0 Phase 0, Rapid Depolarization, is the upstroke of the action potential 1 Phase 1, Early Repolarization, is early brief repolarization before plateau * 2 Phase 2, Plateau, is sustained depolarization * 3 Phase 3, Final Repolarization, is a return to the resting membrane potential 4 Phase 4, Resting/Pacemaker Membrane Potential 37 Membrane Potentials in Autorhythmic cells Lack a constant resting membrane potential Rate of spontaneous pacemakers Sinoatrial (SA) Node 70-80 Atrioventricular (AV) Node 40-60 Bundle of His 20-40 Action potentials per minute 38 Pacemaker Potential: Autorhythmic cells IK 0 Membrane 3 Potential IF (Na+) 4 Following an action potential, K+ channels slowly close, decreasing the outward flow of K+ (IK is a delayed outward rectifier K + channel) There is a constant inward leak of Na+ through IF channels. IF or “funny” channels belong to the HCN (hyperpolarization-activated, cyclic nucleotide- gated) family of channels. Open during hyperpolarization. Thus, the balance flow of ions (Na+ inward and K+ outward) is disrupted, causing the membrane to slowly depolarize during Phase 4 39 Action Potential: Autorhythmic Cells 0 Membrane ICa, L 3 Potential IK 4 ICa, T IF (Na+) Transient “T-type” Ca2+ channels open, bringing the membrane to threshold (voltage-gated, but only open briefly) to start Phase 0. Once threshold is reached, longer lasting “L-type” Ca2+ channels open and there is a large influx of Ca2+ generating the peak of the action potential The delayed outward rectifier K+ channels open and K + leaves, returning the membrane to below threshold during Phase 3 40 Action Potential: Cardiac Contractile Muscle The membrane potential of cardiac contractile cells remains at a resting potential of –90mV until excited Inward rectifier K+ channel is open 0 (IK1) Rapid depolarization (Phase 0) is 4 triggered by arrival of a propagated action potential Opening of voltage-gated “fast” Na+ channels (INa) tetrodotoxin sensitive(cardiac contractile cells only) INa Inward rectifier K+ channel begins IK1 to close with depolarization, but is slow 41 Action Potential: Cardiac Contractile Muscle Early repolarization (Phase 1) is 1 due to the fast Na+ channels 2 starting to close 0 Plateau (Phase 2) is caused by opening of Ca2+ channels (ICa, L) and the closing of the inward rectifier K+ channels …causing 4 ICa, L a plateau at a positive membrane potential Both T-Type and L-type Ca2+ channels are present, but L-type are the predominant channels INa present in contractile cells IK1 42 Action Potential: Cardiac Contractile Muscle 1 Final repolarization (Phase 3) is 2 due to the closing of Ca+2 channels and opening of the delayed outward rectifier K + channels (IK) 0 3 Returns the membrane to resting 4 4 ICa, L Resting membrane potential (Phase 4) IK Inward rectifier K+ channel reopens (IK1), stabilizing IK1 resting potential at –90mV 43 Name that Channel! What channel is primarily responsible for each phase of the action potential? Phase 0 Phase 2 Phase 3 Phase 4 Contractile INA Ica,L Ik Ik1 Delayed Inward outward Fast Na+ channel L-type Ca2+ channel recitifier K+ recitifier K+ channel channel abesen Autorhythmic Ica, L Ik If t Delayed outward Funny L-type Ca+ channel rectifier K+ Channel channel 44 Gap junctions mean first action potential “wins” Cell A Cell B 45 Bundle of His 30 mph Bundle of His 30 mph Bundle of His 30 mph Bundle of His 140 mph 46 Conducting System of the Heart 1. SA Node spontaneously depolarizes more often than other autorhythmic cells (membrane potential of -55mV) Rate = 70-80 bpm Natural Pacemaker of Heart 2. Spreads rapidly throughout atria via interatrial pathways Fibrous “skeleton” isolates atria from ventricles 3. SA node to AV node takes ~100 msec via internodal pathway 47 Conducting System of the Heart 4. AV node transmission takes ~100 msec (fewer gap junctions). Delayed propagation allows atria to complete contraction prior to ventricular contraction 48 Conducting System of the Heart 5. Bundles of His electrically connects atria and ventricles Left bundle branch is larger than the right bundle branch Conduct excitation to the Purkinje fibers (near the apex) and to the papillary muscles Papillary muscles contract early to brace AV valve leaflets 49 Conducting System of the Heart 6. Excitation reaches the Purkinje fibers and causes ventricular myocyte depolarization Fast conduction; many gap junctions at intercalated disks Contraction of the ventricles thus occurs from apex From SA depolarization to ventricular contraction takes ~220msec at rest. 50 Extrinsic Innervation of the Heart Vagus Nerve Atria receive parasympathetic & sympathetic fibers Ventricles receive sympathetic fibers Sympathetic nerves release norepinephrine, causing tachycardia (heart rate increases) Parasympathetic nerves release acetylcholine, causing bradycardia (heart rate slows) 51 Sympathetic Activity = HR Catecholamines from sympathetic nerves (norepinephrine/epinephrine) or epinephrine from adrenal medulla bind to 1-adrenergic receptors on autorhythmic cells 1-receptor Gs ↑Adenylate cyclase ↑cAMP ↑IF & ICa,T Opens more If and ICa,T channels, so more rapid rate of rise to threshold potential 52 Sympathetic Effects on Heart rate Releases norepinephrine at sympathetic ending Causes increased sinus node discharge Increases rate of conduction of impulse Increases force of contraction in atria and ventricles 53 Parasympathetic Activity = HR Acetylcholine from parasympathetic (vagal) nerves binds to muscarinic- cholinergic receptors on autorhythmic cells M2-receptor Gi - ↑IK,Ach ↓Adenylate cyclase ↓cAMP ↓IF & ICa,T Closes some If and ICa,T channels, so less rapid rate of rise Increases K+ permeability – hyperpolarizes cell 54 Parasympathetic Effects on Heart Rate Parasympathetic (vagal) nerves, which release acetylcholine at their endings, innervate S-A node and A-V junctional fibers proximal to A-V node. Causes hyperpolarization because of increased K+ permeability in response to acetylcholine. This causes decreased transmission of impulses maybe temporarily stopping heart rate. 55 The Electrocardiogram LECOM-Bradenton Masters of Medical Sciences Medical Physiology Santiago Lorenzo, Ph.D. The Electrocardiogram 0.8 second Sum of electrical activity in myocardium cells R R +1 – recorded by surface PQ ST electrodes on arms, segment segment Millivolts P wave T wave legs and chest 0 PR Q Key diagnostic for interval S QT interval QRS interval heart abnormalities –1 The Electrocardiogram P wave Atrial depolarization SA node fires, atria depolarize and contract Atrial systole begins ~100 ms after SA signal QRS complex Ventricular depolarization Complex shape of spike due to different thickness and shape of the two ventricles T wave Ventricular repolarization Ventricles relax a few milliseconds after onset of T wave Standardized EKG’s EKG Concepts The P wave immediately precedes atrial contraction. The QRS complex immediately precedes ventricular contraction. Ventricles remain contracted until after repolarization has occurred (end of T wave). The atria remain contracted until the atria are repolarized, but an atrial repolarization wave cannot be seen on the electrocardiogram (why?) EKG Concepts (cont’d) P-R (or P-Q) interval: time between the beginning of atrial depolarization and the beginning of ventricular depolarization. Also, represents the A-V nodal delay. ST segment: ventricular systole (plateau in myocardial action potential) Q-T interval: approximates the time of ventricular contraction. R-R Interval: used to estimate heart rate. Standardized EKG’s Time and voltage calibrations are standardized as shown here. Heart Rate Calculation 0.83seconds R-R interval = 0.83 sec What is the heart rate? The Electrocardiogram Wave of depolarization 1. Atrial depolarization Wave of repolarization begins R 2. Atrial depolarization P P complete (atria Q contracted) S 1 4 Atria begin depolarizing. Ventricular depolarization complete. 3. Ventricles begin to depolarize at apex; atria R repolarize (atria relaxed) P P T 4. Ventricular depolarization Q S complete (ventricles contracted) 2 Atrial depolarization complete. 5 Ventricular repolarization begins at apex and progresses superiorly. 5. Ventricles begin to R R repolarize at apex P P T Q Q S 6. Ventricular repolarization complete (ventricles 3 Ventricular depolarization begins at apex 6 Ventricular repolarization complete; heart relaxed) and progresses superiorly as atria repolarize. is ready for the next cycle. Flow of Electrical Currents in the Chest Around the Heart Ventricular depolarization starts at the ventricular septum and the endocardial surfaces of the heart. The average current flows positively from the base of the heart to the apex. At the very end of depolarization the current reverses and flows toward the outer walls of the ventricles near the base (S wave). 3-Lead and 12-Lead ECG The Standard Leads Electrodes placed on the skin surface to generate different views of the electrical activity of the heart Different configurations of electrodes are referred to as leads Bipolar Limb Leads Lead I: The negative terminal of the electrocardiogram is connected to the right arm, and the positive terminal is connected to the left arm. Lead II: - connected to the right arm, + to the left leg. Lead III: - connected to the left arm, + to the left leg. Bipolar Limb Leads (cont’d) Einthoven’s Law states that the sum of the potentials recorded in leads I and III will equal the potential in lead II (+ and - signs of leads must be observed). If lead I = 1.0 mV, Lead III = 0.5 mV, then Lead II = 1.0 + 0.5 = 1.5 mV. Bipolar Limb Leads (cont’d) 0.5 mV 0.7 mV How does the depolarization vector affect the wave deflection on an ECG tracing? Other EKG Leads Chest Leads (Precordial Leads) know as V1-V6 are very sensitive to electrical potential changes underneath the electrode. Other EKG Leads (cont’d) Augmented Unipolar Limb Leads aVR + electrode is the right arm aVL + electrode is the left arm aVF, + electrode is the left foot Diagnostic Value of ECG Conduction abnormalities & arrhythmias Rx: anti-arrhythmic drugs Myocardial infarction (MI) - abnormal ST segment & T waves Heart enlargement – enlarged QRS Rx: Antihypertensives (ACE inhibitors, diuretics, vasodilators) Electrolyte imbalance (Na+, K+, Ca2+) Rx: supplements or antidiuretics Hormone imbalance Rx: Replacement therapy SINUS TACHYCARDIA Tachycardia: “fast heart rate’ (HR>100bpm) Causes Hyperthermia: 10 beats per 1°F increase in body temp. Sympathetic stimulation: hypovolemia, hypotension, weakening of myocardium Ventricular Tachycardia Serious condition: caused by significant ischemic damage in ventricles, and can lead to v-fib. Digitalis: causes irritable foci that can lead to v-tach Amiodarone and lidocaine can treat v-tach Amiodarone: prolong refractory period, slows A-V conduction Lidocaine: depresses the sodium permeability, blocks impulse Atrial Fibrillation ‘Noisy’ Sawtooth pattern Most common arrhythmia – ~ 2.2 million Americans – Atrial enlargement (heart valve lesions, ventricular failure) is a typical cause – Efficiency only decreased 20-30% – Increased stroke risk – 15% of strokes Normal heart rhythm ~75 bpm Premature Ventricular Contraction (PVC) – causes: hypoxia, electrolyte imbalance, stimulants, stress, emotional irritability, excessive coffee intake – aka extrasystole, premature beat, ectopic beat – Prolonged, high-voltage QRS – Usually benign, but often PVCs can lead to V-fib PVC Atrioventricular Heart Block Causes: ischemia, compression, inflammation, extreme vagal stimulation 1st degree: prolonged P-R interval (constant delay) Also known as “incomplete block” 2nd degree: P-R interval increases until “dropped beat” P wave w/o QRS complex Type 1: progressive prolongation of P-R interval (A-V node (slows down electrical signal slightly that is coming from the atrium to the ventricles, the delay gives time for the ventricles to fill with blood ) abnormality) Type 2: fixed # of non conducted P waves for every QRS complex such as 2:1, 3:1, or 3:2 (His-Purkinje abnormality) 3rd degree: Complete A-V block. P waves dissociated from QRS complex Atrioventricular Heart Block 1st Degree Dropped beat 2nd Degree 3rd Degree Ventricular Fibrillation Most serious cardiac arrhythmia No organized pattern: twitching of entire ventricular myocardium Blood flow stops Loss of consciousness after 4-5 sec Causes: sudden electrical shock to the heart, or ischemia of the heart muscle, or both. Acute MI – ST segment elevation Post-MI (chronic) – enlarged Q waves – ST segment depression 83 Hyperkalemia Suppression of impulse generation by the SA node and reduced conduction by the AV node and His-Purkinje system, resulting in bradycardia and conduction blocks and ultimately cardiac arrest. Peaked T waves P wave widens and flattens, eventually disappears PR segment lengthens Hypokalemia Decrease in the T-wave amplitude As potassium levels decline further, ST-segment depression and T-wave inversions are seen Presence of U wave Cardiac Cycle and Wiggers Diagram LECOM-Bradenton Masters of Medical Sciences Wiggers diagram… Medical Physiology Santiago Lorenzo, Ph.D. Heart Valves Function to prevent back-flow A-V valves: from ventricles into atria Semilunar valves: from arteries into ventricles Chordae tendineae are attached to A-V valves Papillary muscle, attached to chordae tendineae, contract during systole and help prevent excessive bulging of valve and back-flow. Because of smaller opening, velocity through aortic and pulmonary valves exceed that through the A-V valves. 87 Heart Valves Right AV Left AV Posterior Left AV (bicuspid) valve Right AV (tricuspid) valve Openings to coronary arteries Aortic valve Pulmonary valve Chordae Tendinae Anterior 88 Pulmonary The Valves: In situ Chordae tendinae Papillary muscle View within the right ventricle Endoscopic view of aortic (tricuspid valve) valve viewed from above 89 Understanding the Cardiac Cycle 90 Event Recap Systole Isovolumic contraction A-V valves close (ventricular press. > atrial press.) Aortic valve opens Ejection phase Aortic valve closes 91 Event Recap Diastole Isovolumic relaxation A-V valves open Rapid inflow Diastasis - slow flow into ventricle Atrial systole - extra blood in and this just follows P wave. Accounts for ~20-30% of filling 92 Electrocardiography – Electrophysiology Echocardiography – Cardiac Muscle Function 93 Events of the cardiac cycle Wiggers Diagram Electrical ECG Mechanical Pressure & Volume Acoustic Heart Sounds 94 Cardiac Cycle Electrical events (EKG) P - atrial depolarization QRS - ventricular depolarization T - ventricular repolarization Mechanical events Systole – ventricular muscle stimulated by action potential and contracting Diastole – ventricular muscle reestablishing Na+/K+/Ca++ gradient and is relaxing 95 Systole: Contraction and Emptying Diastole: Relaxation and Filling One Cardiac Cycle Ventricular Ventricular diastole systole Atrial Atrial systole Atrial diastole systole Atrial systole immediately precedes ventricular systole Parts of atrial and ventricular diastole overlap 96 Ventricular systole End-Diastolic Volume B A End-Systolic Volume Ventricular Ventricular diastole systole Ventricular systole has two phases A. Isovolumic Contraction: AV, semilunar valves closed B. Ejection : AV closed, semilunar opened 97 Stroke Volume Volume of blood pumped out of the ventricle during a single systole Stroke volume = End-Diastolic Volume – End-Systolic Volume expressed as ml of blood 98 Ventricular diastole End-Diastolic Volume A B End-Systolic Volume Ventricular Ventricular diastole systole Why the bumps in filling? Ventricular diastole has two phases A. Isovolumic Relaxation: AV, semilunar valves closed B. Ventricular Filling Phase: AV opened, semilunar closed 99 Ventricular diastole Ventricular Filling Rapid Atrial Filling systole Reduced Filling Ventricular diastole Ventricular filling phase Rapid Filling Passive filling during atrial and ventricular diastole. As the atria fill, blood passively Reduced Filling flows into the ventricles. Atrial contraction Atrial Contraction then “tops off” the ventricles. 100 Heart Sounds 1st Heart Sound 2nd Heart Sound Follows closing of AV valves Follows closing of Semilunar valves Beginning of Isovolumic Contraction Beginning of Isovolumic Relaxation 101 Pressures determine flow & open-close valves 102 Aortic, Ventricular, and Atrial Pressures Systolic Pressure Diastolic Pressure Ventricular systole Ventricular diastole When is systolic pressure?120mmHG (the highest) When is diastolic pressure?Lowest pressure 103 When is Ventricular Pressure > Aortic Pressure?(A) Ejection Isovolumic Contraction Ventricular systole Ventricular diastole Aortic valve Aortic valve Opens Closes 104 When is Atrial Pressure > Ventricular Pressure? Ventricular Filling Isovolumic Relaxation Rapid Atrial Filling systole Reduced Filling Ventricular systole Ventricular diastole AV valve AV valve Closes Opens 105 Atrial pressure waves a wave - atrial contraction c wave - ventricular contraction (A-V valves bulge) v wave - flow of blood into atria v wave a wave c wave 106 Cardiac Cycle (cont’d) 107 Frank-Starling Mechanism LECOM-Bradenton Masters of Medical Sciences Medical Physiology Santiago Lorenzo, Ph.D. Frank-Starling Mechanism Within physiological limits the heart pumps all the blood that comes to it without excessive damming in the veins. Extra stretch on cardiac myocytes makes actin and myosin filaments interdigitate to a more optimal degree for force generation. 109 Preload and the Frank-Starling Principle Preload is the degree of stretching of the ventricle experienced during diastole - directly proportional to end-diastolic volume Myocyte stretch determines number of sarcomere cross-bridge links, according to the length-tension curve The relationship between myocyte length and tension development is known as the Frank-Starling Principle Often explored by looking at Pressure-Volume Loops Preload and Cardiac Function Developed Ventricular Pressure Ventricular Initial end-diastolic Fiber Length volume (Preload) Preload and the Frank-Starling Principle While looking at Pressure-Volume Loops gives a more detailed understanding, the Frank-Starling Principle is often summarized by the following graph: Stroke Volume vs End-Diastolic Volume “Frank Starling Curve” Preload Frank–Starling Law of the heart: SV EDV Stroke volume is proportional to the EDV Ventricles eject as much blood as they receive The more they are stretched, the harder they contract 113 Preload Preload—the amount of tension in ventricular myocardium immediately before it begins to contract (i.e. end diastolic volume) Increased preload causes increased force of contraction Exercise increases venous return and stretches myocardium Cardiocytes generate more tension during contraction Increased cardiac output matches increased venous return 114 Contractility Contractility refers to how hard the myocardium contracts for a given preload Positive inotropic agents increase contractility Hypercalcemia can cause strong, prolonged contractions and even cardiac arrest in systole Catecholamines increase calcium levels Glucagon stimulates cAMP production Digitcontractionalis raises intracellular calcium levels and strength 115 Contractility Negative inotropic agents reduce contractility Hypocalcemia can cause weak, irregular heartbeat and cardiac arrest in diastole Hyperkalemia reduces strength of myocardial action potentials and the release of Ca2+ into the sarcoplasm Vagus nerves have effect on atria but too few nerves to ventricles for a significant effect 116 Work Output of the Heart External Work 117 Work Output of the Heart 200 End Systolic Volume Intraventricular Pressure 150 Period of Isovolumic Ejection Relaxation (mmHg) 100 Isovolumic Contraction 50 End Diastolic Volume 0 50 100 150 200 Period of Filling Left Ventricular Volume (ml) 118 1 Pressure Volume Loop Pressure Volume Loops shows 1 Cardiac Cycle Left Ventricular Pressure (mmHg) C Aortic Systolic Pressure Aortic Diastolic Pressure A Filling B isovolumic B contraction D Stroke Volume C ejection D Isovolumic Relaxation A Left Ventricular Volume (ml) 119 Pressure Volume Loops Left Ventricular Pressure (mmHg) What has happened to: EDV ESV SV Stroke Volume Left Ventricular Volume (ml) 120 Pressure Volume Loops – Left Ventricular Pressure (mmHg) What has happened to: EDV down ESV equal SV down Stroke Volume Left Ventricular Volume (ml) 121 Left Ventricular Pressure What has happened to: 3 EDV 2 ESV SV 1 4 Left Ventricular Volume 122 B Left Ventricular Pressure What has happened to: 3 EDV 2 ESV SV 1 4 Left Ventricular Volume 123 Overview of the Circulation; Pressure, Flow, and Resistance LECOM-Bradenton Masters of Medical Sciences Medical Physiology Santiago Lorenzo, Ph.D. Major functions of circulatory system Transporting nutrients to the tissues. Transporting waste products away from the tissues Transporting hormones 125 Components of the circulatory system Blood flows in a circuit at rate of 5 L/min. 126 Function of the aorta and large arteries (Highway and Side Roads). 127 Function of arterioles (Traffic and Navigation). 128 Function of capillaries (roundabouts). 129 Function of large veins and venules. 130 Function of the pulmonary circulation. 131 Cross-sectional area in the vascular tree cm2 Aorta 2.5 Small Arterioles CSA of capillaries 20 Arterioles is 1000 times 40 higher than aorta Capillaries 2500 Venules 250 Small Veins 80 Venae Cavae 8 132 Which component of the circulation has the highest velocity of blood flow? Velocity of blood flow is the speed at which blood flows in the circulation (mm/sec) Velocity of Blood Flow = Blood Flow Cross sectional area Aorta >Arterioles > Small veins >Capillaries 133 Pumps and Tubes When using the term “blood flow”, this implies a volume of blood per unit of time, e.g. milliliters of blood per min, or ml/min Velocity, on the other hand, implies a distance traveled per unit of time, e.g., cm per s Flow = Velocity x cross-sectional area 134 Blood Pressure Profile in the Circulatory System High pressures in the arterial tree Low pressures in the venous side of the circulation Large pressure drop across the arteriolar-capillary junction 135 Basic Theory of Circulatory Function Blood flow to tissues is controlled in relation to tissue needs Cardiac output is mainly controlled by local tissue flow Arterial pressure is controlled independent of either local blood flow control or cardiac output control 136 What is blood flow? Blood flow is the quantity of blood that passes a given point in the circulation in a given period of time Unit of blood flow is usually expressed as milliliters (ml) or Liters (L) per minute Overall flow in the circulation of an adult is 5 liters/min which is the cardiac output 137 There are dramatic variations in tissue blood flow in the human body ml/min/ Percent ml/min 100 gm Brain 14 700 50 Heart 4 200 70 Bronchi 2 100 25 Kidneys 22 1100 360 Liver 27 1350 95 Portal (21) (1050) Arterial (6) (300) Muscle (inactive state) 15 750 4 Bone 5 250 3 Skin (cool weather) 6 300 3 Thyroid gland 1 50 160 Adrenal glands 0.5 25 300 Other tissues 3.5 175 1.3 Total 100.0 5000 --- 138 Overview of the Circulation; Pressure, Flow, and Resistance LECOM-Bradenton Masters of Medical Sciences Medical Physiology Santiago Lorenzo, Ph.D. BLOOD FLOW AND CONTROL OF BLOOD PRESSURE Hemodynamics (Fluid Dynamics of Blood) What is blood pressure? Blood pressure is the force exerted by the blood against any unit area of vessel wall Measured in millimeters of mercury (mmHg). A pressure of 100 mmHg means the force of blood was sufficient to push a column of mercury 100mm high Low pressures are sometimes reported in units of mm of water. 1mmHg = 13.6 mm of water 141 Mean Pressure and Pulse Pressure Pulse Pressure = Systolic Pressure - Diastolic Pressure Mean Arterial Pressure = Diastolic Pressure + 1/3 Pulse Pressure Alternative: (2 x Diastolic Pressure + Systolic Pressure)/3 142 Function of Elastic Arteries: Aortic Recoil Elastic arteries prevent pressure from rising too high during systole, and convert intermittent outflow into smooth (but still pulsatile) regular flow across the cardiac cycle 143 Pumps and Tubes Pressure 1 Pressure 2 Pressure = Pressure1-Pressure2 Blood Flow = Pressure pressure gradient, the flow Resistance resistance, the flow 144 What are the major determinants of blood flow? Qc= P/R Flow (Qc) through a blood vessel is determined by: 1) The pressure difference ( P or P1-P2) between the two ends of the vessel 2) Resistance (R) of the vessel P1 P2 Pressure at Pressure at arterial end venous end 145 What is meant by resistance of blood vessels? Resistance is the impediment to blood flow in a vessel Resistance can be calculated by dividing the pressure difference between two points in a vessel by the vessel blood flow R = P = mmHg Q ml/min 146 Pumps and Tubes P1 P2 P = P1 - P2 Flow = P/R Poiseuille’s Law defines resistance as: R= 8Lƞ r4 What can we L, length of the tube change? Ƞ, “eta”, viscosity of the fluid , 3.14… r4, radius of the tube to the 4th power 147 What is the effect of changing vessel diameter on blood flow? Conductance is a measure of the blood flow through a vessel for a given pressure difference Conductance is very sensitive to change in diameter of vessel. The conductance of a vessel increases in proportion to the fourth power of the radius Q = Pr4 8l 148 Characteristics of blood flow in a vessel Blood usually flows in streamlines with each layer of blood remaining the same distance from the wall, this type of flow is called laminar flow When laminar flow occurs, the velocity of blood in the center of the vessel is greater than that toward the outer edge creating a parabolic profile Laminar flow Turbulent flow Blood Vessel 149 What are some causes of turbulent blood flow? Causes of turbulent blood flow High velocities Sharp turns in the circulation Rough surfaces in the circulation Rapid narrowing of blood vessels Turbulent flow Laminar flow is silent, whereas turbulent flow tend to cause murmurs Murmurs or bruits are important in diagnosing vessel stenosis, vessel shunts, and cardiac valvular lesions 150 Remember this… Flow= ΔP/R 1. Blood flows if a pressure gradient is (ΔP) present 2. Blood flows from areas of higher pressure to areas of lower pressure 3. Blood flow is opposed by the resistance (R) of the system 4. Three factors affecting resistance are: a) Radius of the blood vessels b) Viscosity of the blood c) Length of the system 5. Flow is usually expressed in either liters or milliliters per minute (L/min or ml/min) 6. Velocity of flow is usually expressed in either centimeters per minute or millimeters per minute (cm/min or mm/sec) 7. The primary determinant of velocity of flow (when the flow rate is constant) is the total cross sectional area of the vessel(s) Vasoconstriction Vascular smooth muscle tone Vasodilation 151 Vascular Tone: Balance of Influences SYMPATHETIC VASODILATORS VASOCONSTRICTOR EPINEPHRINE, NITRIC OXIDE, NERVE PROSTAGLANDINS, ADENOSINE, HISTAMINE, ATP, K+, H+, CO2, O2, OSMOLARITY Competition between local vasodilator mechanisms which attempt to secure SKELETAL MUSCLE adequate blood flow for RESISTANCE metabolic demand and VESSEL (ARTERIOLE) NO neural vasoconstrictor reflexes attempting to maintain blood pressure VASCULAR SMOOTH MUSCLE ENDOTHELIUM “Balance” Vasoconstrictor Influence Vasodilator Influence 152 Vascular Smooth Muscle: Local Control 153 Vascular Smooth Muscle: Local Control Arterial smooth muscle responds when stretched Stretch causes Ca+2 channels to open in the muscle “Myogenic response” increases its tone Vascular Smooth Muscle: Local Control Autoregulatory Zone Blood Flow An Extremely Important Mechanism for Regulation of Cerebral Blood Flow Arterial Pressure Pressure Autoregulation: The myogenic response in local arterioles will keep tissue blood flow fairly constant in spite of wide deviations in mean arterial pressure 155 Vascular Smooth Muscle: Autonomic Control 156 (Alpha) (Beta) 1 receptors 1 receptors Arteriolar smooth muscle Autorhythmic and contractile cells larger “conduit” vessels Heart Norepinephrine Norepinephrine Sympathetic nerves Sympathetic nerves Epinephrine Epinephrine Adrenal medulla Adrenal medulla Vasoconstriction Increase heart rate and contractility 2 receptors 2 receptors Arteriolar smooth muscle Arteriolar smooth muscle Smaller, more distal vessels Heart and skeletal muscle Norepinephrine Epinephrine Sympathetic nerves Adrenal medulla Epinephrine Adrenal medulla 157 Vasoconstriction Vasodilation BLOOD FLOW AND CONTROL OF BLOOD PRESSURE The Microcirculation Capillaries -The “Sites for Exchange” Capillaries arise from terminal arterioles and dump into post- capillary venules Some contain pre- capillary sphincters Arteriovenous anastamoses directly link arterioles and venules 159 Capillaries -The “Sites for Exchange” Pre-capillary sphincters controlled by local gas concentrations (O2, CO2), local metabolite release (K+, lactic acid), local release of vasoactive substances (nitric oxide, prostaglandins) Blood flow through the capillary bed is determined by pre-capillary sphincter status and arteriolar flow If pre-capillary sphincters are closed, blood flows through “metarterioles” 160 Bulk Flow Across Capillary Wall – Starling Forces Forces causing flow out of the capillary (filtration) 1) Capillary Blood Pressure (Pcap): The hydrostatic pressure exerted on the inside of the capillary walls by the blood (average = 37mmHg) 2) Interstitial Fluid-Colloid Osmotic pressure (IF): The small amount of proteins in the interstitial fluid exert an osmotic effect that “sucks” fluid from the capillaries (average = 0 to 2 mmHg) Pcap IF 161 Bulk Flow Across Capillary Wall – Starling Forces Forces causing flow into the capillary (reabsorption) 1) Interstitial Fluid Hydrostatic Pressure (PIF): The fluid pressure exerted on the outside of the capillary wall by the interstitial fluid (average = 1mmHg; but very difficult to determine) 2) Plasma-Colloid Osmotic pressure (cap): The osmotic effect caused by the large number of proteins in the plasma (average = 25mmHg) which “pulls” water into the capillary (aka oncotic pressure) PIF cap 162 Bulk Flow Across Capillary Wall – Starling Forces Net Exchange Pressures = Outward Pressures versus Inward Pressures If outward pressures are greater than inward pressures, then there is net filtration; if inward pressure are greater than outward pressures, then there is net reabsorption In most tissues in the body, net ultrafiltration is favored (gut is one exception) 35 25 3 2 Out In 35 3 Pcap 2 25 PIF IF cap 37 28 Out > In 7200 L/day 7197 L/day By 9 Net filtration 163 3 L/day Group Discussion - Capillaries 1. Although along capillaries there is a transition from net filtration (arterial end) to net absorption (venous end) find one example where you might find continuous filtration throughout the entire capillary and one example where you will find continuous absorption. 2. If most capillaries have a net filtration, where does the excess fluid from the interstitial space go and how does it get back to the circulatory system? It will be collected by the lymphatic system and then returned back to the circulatory system 3. Histamine increases the intercellular clefts during tissue injury, and more plasma proteins enter the interstitium. How does that change the hydrostatic and osmotic pressures? What would be the end result? This will result in fluid accumulation, and will disrupt the fluid accumulation 164 Cardiac Output, Venous Return, and Their Regulation LECOM-Bradenton Masters of Medical Sciences Medical Physiology Santiago Lorenzo, Ph.D. Cardiac Output Amount ejected by ventricle in 1 minute Cardiac output = HR (heart rate) x SV(stroke volume) About 4 to 6 L/min at rest A red blood cell leaving the left ventricle will arrive back at the left ventricle in about 1 minute Vigorous exercise increases cardiac output to 21 L/min for a fit person and up to 35 L/min for a world-class athlete Cardiac reserve—the difference between a person’s maximum and resting cardiac output ↑with fitness, ↓with disease 166 Cardiac Output Cardiac Output is the sum of all tissue flows and is affected by their regulation CO = 5L/min CO is proportional to tissue O 2 use CO is proportional to 1/TPR (total peripheral resistance) when blood pressure is constant CO = MAP / TPR CO = HR * SV 167 Control of Cardiac Function Intrinsic Afterload Intrinsic Extrinsic Vascular Extrinsic Function Extrinsic – From without Intrinsic - Inherent, essential to the organ 168 Systemic Hemodynamics Flow = P/R Applies to each region Applies to the whole system Flow = Cardiac Output (Qc) Equal to VR/sum of all tissue blood flow Determined by tissues metabolic needs P = Mean Arterial Pressure (MAP) R = “Total Peripheral Resistance” Qc = MAP TPR MAP = QC x TPR 169 Qc = MAP TPR 170 The heart has limits for cardiac output Nervous stimulation Hypertrophy Increase Afterload ANS CHF Valvular deficit CAD Hypoxin 171 Role of nervous system on cardiac output 172 Heart Rate Pulse—surge of pressure in artery Infants have HR of 120-150bpm Young adult females average 72 to 80 bpm Young adult males average 64 to 72 bpm Heart rate rises again in the elderly (Why?) Tachycardia—resting adult HR>100 bpm Stress, anxiety, drugs, heart disease, or fever Bradycardia—resting adult HR of 2 Inflate difficulty 1 < 2 (until equal in size) Pc: pressure collapsing the alveolus THE RESPIRATORY MEMBRANE Made of six layers:- Surfactant fluid layer Alveolar epithelium Alveolar basement membrane Interstitial fluid layer Capillary basement membrane Capillary endothelium THE RESPIRATORY MEMBRANE Total surface area of about 70 square meters and a blood supply of about 60 – 140 milliliters Separate the lung capillary blood from the alveolar air. Allow oxygen diffusion from the alveolar air into the lung capillary blood Allow carbon dioxide diffusion from the lung capillary blood into the alveolar air The Respiratory Membrane Gas Diffusion through the Respiratory Membrane Factors that affect the rate of gas diffusion through the respiratory membrane:- Thickness of the membrane. Surface area of the membrane. Diffusion coefficient of the gas. Partial pressure difference of the gas. Diseases that affect the Respiratory Membrane RESPIRATORY SYSTEM # 2 PULMONARY VENTILATION Processes: A. Pulmonary ventilation: (Air) exchanged by breathing. B. External Respiration: Pulmonary exchange of gases between the lungs and blood. C. Internal Respiration: (Tissue) exchange of gasses between the blood and tissues. Pulmonary Ventilation Basic concepts and definitions - Ventilation called negative draft ventilation (because the air pulled into the lungs by creating a lower pressure inside the chest compared to the outside air) - Breathing in called inspiration or inhalation - Breathing out called expiration or exhalation - Alveolar pressure:- Pressure within the lung (pressure inside the tiny air sacs in your lungs. Changes as you breathe in and out) - Pleural pressure:- Pressure within the pleural cavity - Transpulmonary pressure:- Difference between alveolar and pleural pressures. (this is what keeps the lungs open and inflated. When the pressure difference is higher then the lungs are more expanded and when lower the lungs are less expanded) Standard Notations Symbol Definition Example Px Partial pressure of x PCO2: partial pressure of CO2 Fx Fractional volume or pressure FN2: nitrogen fraction of pressure Sx Saturation, decimal fraction SO2: % saturation of hemoglobin with or % oxygen Cx Concentration (content) CO2: total oxygen content Locations a Arterial blood PaCO2: carbon dioxide partial pressure of arterial blood v Mixed venous PvCO2: carbon dioxide partial pressure of venous blood c Pulmonary capillary blood PcO2: oxygen partial pressure of arterial blood A Alveolar gas PAO2: alveolar oxygen partial pressure I Inspired gas FIO2: oxygen fraction of inspired gas E Mixed expired gas PECO2: CO2 partial pressure in expired air Respiratory Muscles Muscles of Inspiration Diaphragm:- Contract downwards External intercostal:- Raise the rib cage upwards. Scaleni:- Lift the first two ribs upwards. Anterior Serrati:- Lift many of the ribs upwards. Sternocleidomastoid:- Lift the sternum upwards. Muscles of Expiration Diaphragm:- relax upwards. Internal intercostal:- Pull the rib cage downwards. Abdominal Recti:- Pull lower ribs downwards. Gas Exchange 1. Gas behavior: Gas laws. a. Charle's law: As a gas is heated it will expand. b. Dalton's law: Each gas in a mixture will exert Its own pressure proportional to its % concentration. In air: pO2= 20% of 760 mmHg or 160 mmHg pCO2= 0.04 % of 760 mmHg or 0.3 mmHg. c. Henry's law: The amount of a gas that will dissolve in a liquid (Blood or plasma) is proportionally related to its partial pressure and solubility coefficient. Dalton's Law In a gas mixture the pressure exerted by each individual gas in a space is independent of the pressure exerted by other gases. Patm = PH2O+PO2+PN2 Pgas = % total gases * Ptotal Sea Level: dry gas Patm=760mmHg-47mmHg=713mmHg PO2=0.2093*713mmHg BOYLE’S LAW As the size of a closed container decreases, pressure inside the container is increased As the size of a closed container increases, pressure inside the container is decreased Pulmonary Ventilation Quiet Resting Ventilation Air moves into the lungs when alveolar pressure inside lungs is less than atmospheric pressure: Contraction of the diaphragm pulls the lower surface of the lungs downwards, enlarges the chest cavity and reduces alveolar pressure to about -1 cmH2O (below than atmospheric pressure). Air drafts into the lungs as a negative pressure draft. Inspiration or Inhalation is done. Pulmonary Ventilation Quiet Resting Ventilation Air moves out of the lungs when atmospheric pressure is less than the alveolar pressure inside the lungs: - Relaxation of the diaphragm pushes the lower surface of the lungs upwards and reduces the chest cavity. - Elastic recoil of the alveoli, chest wall and the abdominal muscles compress the lungs and creates an alveolar pressure greater than atmospheric pressure. - Air is pushed out of the lungs. - Expiration or exhalation is done. Ventilation Cycle Forced Ventilation During Exercise Forced inspiration Requires a larger decrease in alveolar pressures than resting inspiration:- Diaphragm, External Intercostal, Scaleni, Anterior Serrati and Sternocleidomastoid muscles contract more forcefully making the chest wider. Decreased alveolar pressure to lower values results in deeper breaths Forced Ventilation During Exercise Forced Expiration Requires a larger increase in alveolar pressures than resting expiration:- Diaphragm and External Intercostal muscles relax Accessory muscles: Internal Intercostal muscles contract compressing rib cage making the chest smaller. Abdominal Recti contract compressing abdominal organs and forcing the diaphragm to move up further Increased alveolar pressure force more air out Pulmonary Ventilation Dead space air Air that fills the conducting airways or pathologically damaged alveoli and is not involved in the gas exchange process. Anatomic dead space The conducting division of airways Physiologic dead space sum of anatomic dead space and any pathological alveolar dead space with no gas exchange Alveolar ventilation rate air that ventilates alveoli X respiratory rate directly relevant to ability for gas exchange External respiration O2 enters and CO2 exits the blood from the alveoli. Several factors contribute: a. Partial pressure differences: pO 2 in lung > pO2 in blood. Direct proportionality (160 vs 100 mmHg). b. Surface area: Lung beds (alveoli) have 750 sq ft of exchange. Direct proportionality. c. Diffusion distance: Alveolar thickness which the gases must diffuse across. Indirect proportionality. d. Breathing rate: Increases gas exposure and exchange. Direct proportionality if TV (tidal Volume) is constant. Which way is the concentration gradient oriented for O2? and CO2? Lung Volumes Lung Volumes and Capacities Volume is one measure of quantity of air Capacity is sum of two or more volumes Spirometer or respirometer device for measuring volumes and capacities recorded as a Spirogram Lung Volumes and Capacities Lung volumes (1) Tidal Volume (Vt) Volume of air in one breath (2) Inspiratory Reserve Volume (IRV) Volume of air inspired in addition to VT (3) Expiratory Reserve Volume (ERV) Volume of air expired in addition to VT (4) Residual Volume (RV) Volume of air that cannot be expired even with maximum forced expiration. Lung Volumes and Capacities Lung Capacities: (1) Inspiratory Capacity (IC) = Vt + IRV (2) Functional Residual Capacity: (FRC) = RV + ERV (3) Vital Capacity (VC) = Vt + IRV + ERV (4) Total Lung Capacity (TLC) = Vt + IRV + ERV + RV The Spirogram LUNG VOLUMES AND CAPACITIES FEV1 stands for Forced Expiratory Volume in one second Represent the percentage of vital capacity that is forcedly expired in one second Used in clinical settings to evaluate lung function Should be 75% or higher in healthy adults Lower values than 75% indicate airways obstruction, lung diseases or weakness of the respiratory muscles Flow-volume Curve Max effort Submax effort Flow How does F/V loop look in obstructive and restrictive disease? 6 1 Volume (liters) Airway Resistance during Forced Exhalation Increased Airway Resistance with Forced Exhalation +.25 +5 -8 -8 +25 +25 +.5 +15 -8 -8 +25 +25 +1 +25 +2 +35 -8 -8 +25 +25 Passive Expiration Forced Expiration PULMONARY BLOOD FLOW Blood Volume Approximately 500 ml Can shift to systemic circulation Measurement of Flow MEASUREMENT OF PULMONARY BLOOD FLOW (i.e. Cardiac Output) Fick Principle VO2=Q(CaO2-CvO2) VO2 = Oxygen Consumption CaO2 = Arterial Content Q = Blood flow CvO2 = Venous Content MEASUREMENT OF PULMONARY BLOOD FLOW VO2=Q(CaO2-CvO2) CaO2 = 20 ml O2/100 ml blood VO2 = 250 ml/min CvO2 = 15 ml O2/100 ml blood Q = 250 ml O2/min = 250 ml O2 * 100 ml blood (20-15) ml O2/100 ml blood min 5 ml O2 Q = 5000 ml blood /min DISTRIBUTION OF BLOOD FLOW Blood Flow Bottom Top Distance up Lung Hydrostatic Effects on Blood Flow No zone 1 in a healthy lung Pa =arterial PA = alveolar Pv =venous Ppc=pulmonary capillary The aterial pressure is higher than the alveolar Distance We have a pressure that is higher Flow DISTRIBUTION OF BLOOD FLOW Once you start exercising then the blood flow Will go up everywhere Blood Flow Exercise Rest Bottom Top Distance up Lung In the lungs when there is hypoxic then the the vessels constrict, why?So, it can allow the Air to go to places that are well ventenilated O2= 150 CO2 =0 O2=100 ↓O2 ↓O2 CO2 =40 ↑CO2 ↑CO2 O2= 40 O2=100 O2= 40 ↓O2(40) ↓O2 CO2 =45 CO2 =40 CO2 =45 ↑CO2(45) ↑CO2 A) normal B) hypoxic C) hypoxic vasoconstriction O2=100 CO2 =40 Pulmonary Capillary Dynamics Outward Forces Pulmonary capillary pressure 7 mmHg Interstitial osmotic pressure 14 mmHg Negative interstitial pressure 8 mmHg Total 29 mmHg Inward Forces Plasma osmotic pressure 28 mmHg Net filtration pressure 1 mmHg Negative interstitial pressure keeps alveoli dry Pulmonary Capillary Dynamics Forces Outward Pcap + πint – Pint 7 + 14 + 8= 29 Forces Inward 28 Net movement +1 outward Outward fluid movement is transferred to lymphatics….similar to systemic circulation. RESPIRATORY SYSTEM # 3 GAS EXCHANGE AND GAS TRANSPORT Physical Principles of Gas Exchange Diffusion in response to concentration gradient Pressure proportional to concentration Gas contributes to total pressure in direct proportion to concentration CO2 20 times as soluble as O2 Air is humidified yielding a vapor pressure of 47 mmHg Determinants of Diffusion Ficks Law Diffusion = (P1-P2 ) * Area * Solubility Distance * MW Pressure Gradient Area Distance Solubility and MW are fixed Gas Exchange In lungs – Alveolar Gas Exchange Diffusion of O2 from alveolar air into blood Diffusion of CO2 from the blood into alveolar air In tissues – Systemic Gas Exchange Diffusion of O2 from blood into tissues Diffusion of CO2 from tissues into blood Diffusion across the extremely thin respiratory membrane or the capillary wall from higher to lower concentrations of gases ALVEOLAR GAS EXCHANGE ALVEOLAR GAS EXCHANGE SYSTEMIC GAS EXCHANGE High metabolic activity will make more CO2 SYSTEMIC GAS EXCHANGE Gas Diffusion through the Respiratory Membrane Factors that affect the rate of gas diffusion through the respiratory & capillary membrane:- Thickness of the membrane. Surface area of the membrane. Diffusion coefficient of the gas. Partial pressure difference of the gas. Measurements of Gas Partial Pressure Dalton’s Law of Partial Pressure “In a mixture of gasses, the total pressure is equal to the sum of pressures contributed by each individual gas” These individual pressures are partial pressures Symbol for the partial pressure of a gas is Pg where g stands for the specific gas Partial Pressures and Gas Exchange Partial pressure of O2 and CO2 in well oxygenated blood PaO2 is symbol for partial pressure of oxygen in well oxygenated (arterial ) blood and is about 95 mm Hg PaCO2 is symbol for partial pressure of carbon dioxide in well oxygenated (arterial) blood and is about 40 mm Hg Partial Pressures and Gas Exchange Partial pressure of O2 and CO2 in poorly oxygenated venous blood PvO2 is symbol for partial pressure of oxygen in poorly oxygenated (venous) blood and is about 40 mm Hg PvCO2 is symbol for partial pressure of carbon dioxide in poorly oxygenated (venous) blood and is about 45 mm Hg Gas Exchange Diagram Alveolar Systemic O2= 104 PaO2=95 mm Hg CO2=40 PaCO2=40 mm Hg O2 Alveolus Well Oxygenated Blood CO2 O2 CO2 PvO2=40 mm Hg O2 =40 PvCO2=45 mm Hg Poorly Oxygenated Blood CO2 =45 Tissues Effect of alveolar ventilation on alveolar PO2 at two metabolic rates PO2 controlled by 1) Rate of absorption 2) Rate of O2 entry via ventilation. Metabolic rate 250 vs. 1000 ml O2/min Increasing metabolic rate means increased O2 absorption (due to increased utilization) and increased ventilation is required to maintain arterial PO2. Normal operating point Hyperventilation-ventilating above needs (PAO2>100 mm Hg) Hypoventilation-ventilating below needs (PAO2