PHYS 5024 Heart Lecture Notes Weeks 3 & 4 PDF
Document Details
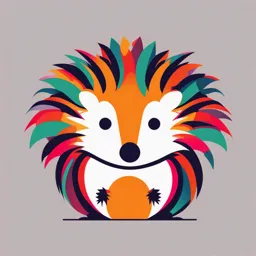
Uploaded by CompactCobalt3681
Sonoran University of Health Sciences
Tags
Summary
This document provides lecture notes on heart physiology, specifically covering cardiac muscle, action potentials, pacemaker activity, and electrocardiograms. It details the electrical and mechanical functioning of the heart, focusing on key aspects relevant to cardiac function.
Full Transcript
PHYS 5024 Lecture notes Weeks 3 & 4 Heart For this week read Chapter 12; next week read Chapter 13 in Rhoades and Bell Cardiac muscle relatively small cells (compared to skeletal muscle fibers) cell network: cells held together end-to-end adjacent cells linked...
PHYS 5024 Lecture notes Weeks 3 & 4 Heart For this week read Chapter 12; next week read Chapter 13 in Rhoades and Bell Cardiac muscle relatively small cells (compared to skeletal muscle fibers) cell network: cells held together end-to-end adjacent cells linked by gap junctions action potentials propagate from cell to cell calcium-activated calcium release from sarcoplasmic reticulum high oxygen requirement, many mitochondria Cardiac action potential key features: *long duration during which significant Ca++ entry into the cells *some heart cells have spontaneous firing *refractory period *Negative inside resting potential, close to the potassium equilibrium potential, due to a potassium channel that remains open when the cells are at "rest" (not actively generating an action potential) phases of the ventricular action potential (0-4) phase 0: voltage-gated sodium channels open. Na+ entry shifts the membrane potential from the resting potential to a positive inside value phase 1: similar to a neuronal action potential when sodium channels start to close and a voltage-gated potassium channel opens, but.... phase 2: voltage-gated calcium channels open, allowing Ca++ entry and holding the membrane potential near zero volts for an extended period phase 3: calcium channels close, potassium movement out of the cell again dominates, returning the potential to the resting potential phase 4: resting potential physiological relevance of Ca++ entry during phase 2: calcium entering the cell contributes significantly to increasing the cytoplasmic level of Ca++ *contributes to the force of contraction of heart muscle *target for autonomic regulation of force of contraction 1 receptors increase force of contraction atrial action potential: shorter phase 2 Pacemaker activity in the heart - SA and AV nodes phase 4: potassium permeability decreases and sodium and calcium permeabilities increase, shifting membrane potential more positive inside. phase 0: calcium influx Regulation of SA node conduction (chronotropic effects) parasympathetic: acetylcholine (Ach) slows the heart rate (bradycardia, < 60 beats/min) (increased potassium current, involves a G protein coupled receptor response, also inhibitory effects on If) sympathetic: norepinephrine increases the rate (tachycardia, > 100 beats/min) (increased Na+ current, If) seems to involve regulation of a cyclic nucleotide–gated channel "Familial Sinus Bradycardia Associated with a Mutation in the Cardiac Pacemaker Channel" http://content.nejm.org/cgi/content/full/354/2/151 Regulation of AV node conduction (dromotropic effect) ACh slows the conduction rate norepinephrine increases the conduction rate Spread of action potentials in the heart. SA node atrial muscle- 1 m/sec AV node- (0.05 – 0.1 m/sec) *regulation by drugs and conditions like hypoxia can block ventricular contraction *failure to conduct some signals from the atrium can help such as in atrial fibrillation Purkinje fibers (2 m/sec) – rapid spread to inner surface of ventricular muscle Ventricular muscle (0.3 m/sec) Electrocardiogram (ECG) changes in extracellular charge create an electrical dipole oriented positive towards the direction of action potential spread which can be detected by electrodes placed on the body. P wave: spread of atrial action potentials PR segment: corresponds to AV node conduction QRS complex: ventricular depolarization Q wave: depolarization in the septum R wave: reflects depolarization of ventricular muscle S wave: last part of ventricular depolarization ST segment: most ventricular cells are depolarized T wave: ventricular repolarization QT interval: close in duration to the duration of a ventricular action potential Conventional ECG electrode positions measure the electric potential between pairs of conductors (a "lead") Bipolar limb leads I, II and III of the Einthoven triangle 3 augmented limb leads (aVR, aVL, aVF) *exploring electrode is one of the limb electrodes *the reference voltage is from the combination of the other two limb electrodes the limb leads have ECG results of differing magnitude and polarity according to the hexaxial system of the frontal plane the chest leads reflect electric dipoles at various angles in the horizontal plane. *6 chest leads (V1 –V6) *exploring electrode at one of 6 positions on the chest *the three limb leads are combined as the reference voltage Examples of cardiac cycle irregularities detected by the ECG arrythmias *respiratory sinus arrhythmia *slowed heart rate due to parasympathetic stimulation can demonstrate pacemaker activity of the AV node atrial fibrillation. irregular rate, no P wave ventricular contraction from an ectopic "focus" or pacemaker *an example where the mean electrical axis is abnormal AV conduction block: P wave not synchronized with the QRS complex bundle branch block: abnormal and wide QRS complex Cardiac output (CO) -volume of blood pumped out of the heart per minute (L/min) -output is the product of the heart rate (HR) and the stroke volume (SV) *stroke volume: volume of blood ejected from ventricle during a beat of the heart * CO = SV x HR * CO is often normalized to body size * SV = (end-diastolic volume, EDV) minus (end-systolic volume) ejection fraction (EF) - a measure of heart performance * EF= (SV/EDV) x 100 The cardiac cycle -atrial systole -ventricular systole -ventricular diastole pressure changes -in a resting individual, atrial and ventricular pressures are nearly equal except during ventricular systole and the first phase of ventricular diastole (isovolumic relaxation). - atrial and ventricular pressures are low at the end of ventricular diastole, roughly equal to the low venous return pressure -"a wave": the increase in blood pressure in the heart during atrial systole *in a resting individual, atrial contraction causes only a small increase in ventricular volume because most ventricular filling has already been accomplished during diastole -ventricular systole: ventricular contraction generates a large increase in ventricular pressure *when ventricular pressure exceeds atrial pressure, the AV valves close. *"v wave": increasing atrial pressure during ventricular systole due to atrial filling *"c wave": small pulse of increased atrial pressure reflecting ventricular contraction valves -atrioventricular (AV) valves *left heart: mitral (bicuspid) *right heart: tricuspid *first heart sound: start of ventricular systole, valve closure -semilular valves *aortic *pulmonic *second heart sound: end of ventricular systole, valve closure other heart sounds (minor) -S3: associated with rapid filling of the ventricles during diastole -S4: associated with blood movement during atrial systole pressure changes (continued) -during ventricular diastole, when the atrial and ventricular pressures are again nearly equal, the AV valves open, blood flows through them and pressure decreases -increased ventricular pressure during ventricular contraction *rapid increase during isovolumic contraction until semilunar valve opening *some additional pressure increase during the rapid ejection phase *pressure falls during the reduced ejection phase (ventricular contraction is ending). *when ventricular pressure falls below arterial pressure, semilunar valves close and ventricular pressure continues to fall during isovolumic relaxation. *when atrial and ventricular pressures are equal, the AV valves open and ventricular filling starts. Pressures fall and match the "preload" Preload -during ventricular filling, the ventricles enlarge to the point where the resting (diastolic) force of the ventricle roughly matches the pressure of the venous return to the heart. -in a resting individual, the particular ventricular volume achieved and cardiac muscle fiber length at the start of ventricular systole corresponds to the preload -at the end of ventricular diastole, atrial and ventricular pressures are low and about the same as the pressure of the venous return to the heart -heart disease: smaller end-diastolic volumes and reduced stroke volume Afterload -the force against which the ventricle has to work in order to move blood from the ventricle into the artery -afterload is roughly the arterial pressure -if the afterload is increased, there is an immediate effect to reduce stroke volume aortic pressure -higher than ventricular pressure during most of the cardiac cycle * aortic valve closed -nearly the same as the left ventricular pressure during the ejection phase of the cardiac cycle. -dicrotic notch (incisura): some blood flows back into the heart just as the aortic valve closes causing a small dip in the aortic pressure Ventricular volume -decreases during the blood ejection phase of systole (after semilunar valve opening) as the force of contraction forces blood into the arteries. -increases during the filling phase of ventricular diastole (after the atrioventricular valve opens) until the volume corresponding to the preload is attained Starling's law of the heart (Frank-Starling relationship) -describes the relationship between ventricular end-diastolic volume and stroke volume - end-diastolic volume, cardiac fiber length, and pressure are all directly related - for typical fiber lengths and corresponding end-diastolic volume, longer lengths correspond to greater force of contraction - an increase in preload generally results in an increase in stroke volume -balancing *Starling's law provides a mechanism for balancing systemic and pulmonary outputs *example: if the right side of the heart pumps more blood than the left, left atrial pressure will increase as will left ventricle filling, stroke volume and left heart output. Regulation of cardiac fiber contractility positive inotropy (increased force of contraction) - Bowditch staircase (staircase phenomenon, treppe): faster heart rates normally cause a greater strength of contraction because of an intrinsic effect (independent of autonomic regulation) - sympathetic nerve stimulation increases the force of contraction *mechanism: the concentration of Ca++ reaching the contractile filaments is limiting for force generation in cardiac muscle. Ca++ entering through surface membrane calcium channels plays a significant role in triggering Ca++ release from the sarcoplasmic reticulum. Activation of 1 adrenergic receptors enhances calcium entry during the action potential and increases force of contraction (cAMP-dependent protein kinase phosphorylates the calcium channels causing enhanced calcium influx). - cardiac glycosides such as digitalis increase the force of contraction *mechanism: inhibition of the sodium-potassium pump and reduced sodium gradient. This reduces the ability of a sodium-calcium exchanger to remove calcium from the cells, making more calcium available for contraction. congestive heart failure - reduced pumping efficiency (ventricular function curve shifted right) - cardiac glycosides can be used to treat congestive heart failure * shifts ventricular function curve back towards normal "William Withering and digitalis, 1785 to 1985" http://www.pubmedcentral.nih.gov/pagerender.fcgi?tool=pmcentrez&artid=1415366&pageindex=1 negative inotropy (decreased force of contraction) -parasympathetic stimulation decreases force of contraction *mechanism: mAchR activation lowers calcium influx Pressure-Volume loops -normal -increased preload *larger end-diastolic volume, greater fiber length and force of contraction allows for an increased stroke volume -effect of afterload on stroke volume * if arterial pressure increases, more of the force of ventricular contraction must be exerted to push blood into the arteries and stroke volume will decrease * end systolic volume increases * preload also increases, but end-diastolic volume does not increase as much as end-systolic volume -effect of increased contractility * end systolic volume is decreased, stroke volume increases Effect of heart rate on stroke volume -increased heart rate: shorter diastole reduces the time for ventricle filling so stroke volume decreases -decreased heart rate: longer diastole allows longer ventricular filling and larger stroke volume Enlarged heart - hypertrophy and enlargement of the heart is a natural response to heart disease and inadequate heart output. - for a given ventricular filling pressure, a larger heart will have greater wall tension. If too large, then you are off the right end of the Starling ventricular function curve and further enlargement does not increase cardiac output. Diastolic compliance -some forms of heart disease reduce ventricular compliance. -the ventricle does not inflate normally for a given pressure -to maintain output, end-diastolic pressure can be increased, but this can cause unwanted fluid accumulation along the pulmonary circuit.