Week 3 - Cell - The Living Units PDF
Document Details
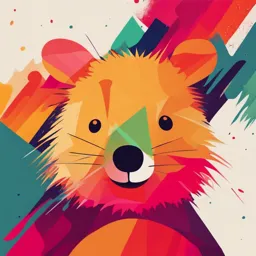
Uploaded by PrivilegedRubellite7992
Tags
Summary
This document covers the fundamental concepts of cell biology. It details the structure and function of cells, focusing on the plasma membrane, cytoplasm, and organelles. The document also includes a learning outcome, and definitions useful for understanding cell structures and functions.
Full Transcript
Cells: The Living Units Learning outcome • What is a plasma membrane and its structure • How does the substances move across the plasma membrane • Looking closer to the passive and active transports • How does the a cell generate a voltage across the plasma membrane • How does the plasma membrane...
Cells: The Living Units Learning outcome • What is a plasma membrane and its structure • How does the substances move across the plasma membrane • Looking closer to the passive and active transports • How does the a cell generate a voltage across the plasma membrane • How does the plasma membrane allows the cells to interact with the environment • Cytoplasm, structure and its organelles • Nucleus, the structure, its function and the role of DNA and RNA • How does cells grow and divide Cells: The Living Units • The cell is the smallest unit of life. When you define the properties of cells, you define the properties of life. • All organisms are made of one or more cells. Cells are the structural and functional building blocks of an organism. Different cell types have different functions within an organism, and the activity of an organism depends on the activities of individual cells and of all of the cells together. • Cells only arise from other cells. Most body cells arise by mitosis. However, sperm and ovum (egg) cells arise by a related process called meiosis. Cell Diversity • Over 250 different cell types that vary greatly in shape, size, and function. • Cells also vary in length- ranging from 2 micrometers in the smallest cells to over a meter in the nerve cells. • A cell's shape reflects its functions. • Regardless of these differences, all cells have the same basic parts and some common functions. Structure of the generalized cell A human cell has three main parts: • The plasma membrane: the outer boundary of the cell, which acts as a selectively permeable barrier. • The cytoplasm: the intracellular fluid packed with organelles, small structures that perform specific cell functions. • The nucleus: an organelle that controls cellular activities. Typically the nucleus lies near the cell 's center. Extracellular materials They are substances contributing to body mass that are found outside the cells. The major classes of extracellular materials are: 1. Extracellular fluid: Extracellular fluid (ECF) includes interstitial fluid, blood plasma, and cerebrospinal fluid. ECF dissolves and transports substances in the body. Interstitial fluid is the fluid in tissues that bathes all of our cells, and has endless major roles to play. 2. Cellular secretions. These secretions include substances that aid in digestion (intestinal and gastric fluids) and some that act as lubricants (saliva, mucus, and serous fluids). 3. Extracellular matrix. Most body cells are in contact with a jellylike substance composed of proteins and polysaccharides. Plasma Membrane (cell membrane) • plasma membrane separates two of the body's major fluid compartments-the intracellular fluid within cells and the extracellular fluid outside cells. • The plasma membrane is much more than a passive envelope. Its unique structure allows it to play a dynamic role in cellular activities. • The plasma membrane is a double layer of phospholipids with embedded proteins. The chemical composition of the plasma membrane and relate it to membrane functions. • Structure composed of a double layer, or bilayer, of lipid molecules with protein molecules "plugged into" or dispersed in it. The proteins, many of which float in the fluid lipid bilayer, form a constantly changing mosaic pattern. • Membrane Lipids: The lipid bilayer forms the basic "fabric" of the membrane. It is constructed largely of phospholipids, with smaller amounts of cholesterol. The chemical composition of the plasma membrane and relate it to membrane functions. The result is that all plasma membranes, indeed all biological membranes, share a sandwich-like structure: They consist of two parallel sheets of phospholipid molecules lying tail to tail, with their polar heads bathed in water on either side of the membrane. The plasma membrane is a phospholipid bilayer with embedded proteins arranged as a fluid mosaic. Functions of the Plasma Membrane: 1. 2. 3. 4. Physical barrier: Encloses the cell, separating the cytoplasm from the extracellular fluid. Selective permeability: Determines which substances enter or exit the cell. Communication: Plasma membrane proteins interact with specific chemical messengers and relay messages to the cell interior. Cell recognition: Cell surface carbohydrates allow cells to recognize each other. Membrane Proteins • There are two distinct populations of membrane proteins, integral and peripheral: 1. Integral proteins are firmly inserted into the lipid bilayer. Some protrude from one membrane face only, but most are transmembrane proteins that span the entire membrane and protrude on both sides. All integral proteins have both hydrophobic and hydrophilic regions. This structural feature allows them to interact with both the nonpolar lipid tails buried in the membrane and the water inside and outside the cell. Membrane Proteins • Peripheral Proteins Unlike integral proteins, peripheral proteins are not embedded in the lipid bilayer. Instead, they either attach loosely to integral proteins or have a hydrophobic region that anchors them into the membrane. • Peripheral proteins include a network of filaments that helps support the membrane from its cytoplasmic side. • Some peripheral proteins are enzymes. Others are motor proteins involved in mechanical functions, such as changing cell shape during cell division and muscle cell contraction. Peripheral proteins include a network of filaments that helps support the membrane from its cytoplasmic side Membrane proteins perform many tasks a) b) c) d) e) Transport Receptors for signal transduction Enzymatic activity Cell-cell recognition Attachment to the cytoskeleton and extracellular matrix (ECM) f) Cell-to-cell joining Membrane Carbohydrates and the Glycocalyx • The extracellular surface (but not the intracellular surface) of the membrane is decorated with short branching carbohydrates. • These are attached to most of the membrane's proteins and some of the membrane's lipids that are exposed on the exterior surface. • Lipids and proteins with sugars attached are called glycolipids and glycoproteins, respectively. Glycolipids have two fatty acid tails (like phospholipids), but a carbohydrate replaces the phosphate head group. • The glycocalyx consists of the fuzzy, sticky, carbohydrate-rich area at the cell surface created by the sugars of glycoproteins and glycolipids. Your cells are sugar-coated like breakfast cereal. • Because every cell type has a different pattern of sugars, it will provide a specific biological markers by which approaching cells recognize each other. Like Immune cells. Membrane Carbohydrates and the Glycocalyx Cell Junctions In many cases, the plasma membranes of adjacent cells are joined together by specialized cell junctions that allow neighboring cells to adhere and sometimes to communicate. Which includes: 1. Tight Junction: a series of integral protein molecules in the plasma membranes of adjacent cells fuse together like the zipper- Its Impermeable junction 2. Desmosomes: serve as anchoring junctions-mechanical couplings scattered like rivets along the sides of adjacent cells to prevent their separation. On the cytoplasmic face of each plasma membrane is a button like thickening called a plaque. Adjacent cells are held together by thin linker protein filaments (cadherins) that extend from the plaques and fit together like Velcro® in the intercellular (between cells) space. Desmosomes are abundant in tissues subjected to great mechanical stress, such as skin and heart muscle. Cell Junctions 3. A gap junction: is a communicating junction between adjacent cells. At gap junctions the adjacent plasma membranes are very close, and the cells are connected by hollow cylinders (called connexons) composed of transmembrane proteins. Different types of gap junctions are composed of different transmembrane proteins, and they determine what can pass through them from one cell to its neighbor. Ions, simple sugars, and other small molecules pass through these water-filled channels. Gap junctions are present in electrically excitable tissues, such as the heart and smooth muscle, where ion passage from cell to cell helps harmonize their electrical activity and contraction. Passive membrane transport • Passive membrane transport is diffusion of molecules down their concentration gradient. • The three types of passive transport across the plasma membrane are simple diffusion, facilitated diffusion, and osmosis. ** Diffusion: is the movement of molecules or ions from an area where they are in higher concentration to an area where they are in lower concentration. Movement from high to low concentration is also called movement down or along a concentration gradient. Passive membrane transport Simple Diffusion • In simple diffusion , substances diffuse directly through the lipid bilayer. Such substances are usually small nonpolar molecules that readily dissolve in lipids (are lipid soluble). These include gases (such as oxygen and carbon dioxide), steroid hormones, and fatty acids. • The two criteria that determine how easily a substance will pass by simple diffusion through a plasma membrane are (1) lipid solubility and (2) size. • Simple diffusion is not an all-or-none thing: Some substances diffuse readily and others hardly at all. For example, water is not lipid soluble and you would expect it to be repelled by the hydrophobic lipid tails of the membrane's core. However, its very small size allows very small amounts to move across the lipid bilayer by simple diffusion. Facilitated Diffusion • Certain molecules, notably glucose and other sugars, some amino acids, and ions are transported passively even though they are unable to pass through the lipid bilayer. Instead they move through the membrane by a passive transport process called facilitated diffusion in which the transported substance either: 1. binds to carrier proteins in the membrane and is ferried across. 2. moves through water-filled channel proteins. Carrier-mediated facilitated diffusion. • Carriers are transmembrane proteins that are specific for transporting certain polar molecules or classes of molecules, such as sugars and amino acids, that are too large to pass through membrane channels. Alterations in the shape of the carrier allow it to first envelop and then release the transported substance, allowing it to bypass the nonpolar regions of the membrane. Essentially, the carrier protein changes shape to move the binding site from one face of the membrane to the other. • Carrier-mediated transport is limited by the number of protein carriers that are available. For example, when all the glucose carriers are "engaged," they are said to be saturated, and glucose transport is occurring at its maximum rate. Glucose transport within the body is typically unidirectional-into the cells. Channel-mediated facilitated diffusion. • Channels are transmembrane proteins that transport substances, usually ions or water, through Aqueous Channels from one side of the membrane to the other. Channels are selective due to pore size and the charges of the amino acids lining the pore. • Leakage channels are always open and simply allow ions or water to move according to concentration gradients. • Gated channels are controlled (opened or closed), usually by chemical or electrical signals. Like carriers, many channels can be inhibited by certain molecules, show saturation, and tend to be specific. • Substances moving through them also follow the concentration gradient. Channel-mediated facilitated diffusion. Osmosis • The diffusion of a solvent, such as water, through a selectively permeable membrane is osmosis. Osmosis is extremely important in determining the distribution of water in the various fluid-containing compartments of the body (cells, blood, and so on). • As we mentioned earlier, even though water is highly polar, a small amount of it can "sneak through" the plasma membrane by osmosis because of its small size. Water also moves freely and reversibly through water-specific channels constructed by transmembrane proteins called aquaporins (AQPs) • As solute concentration increases, water concentration decreases • The total concentration of all solute particles in a solution is referred to as the solution's osmolarity Influence of membrane permeability on diffusion and osmosis. Influence of membrane permeability on diffusion and osmosis. Osmotic Pressure And The Hydrostatic Pressure • As a rule, the higher the amount of nondiffusible, or nonpenetrating, solutes in a cell, the higher the osmotic pressure and the greater the hydrostatic pressure must be to resist further net water entry • Osmotic imbalances cause cells to swell or shrink (due to net water gain or loss) until either: (1) the solute concentration is the same on both sides of the plasma membrane. (2) the membrane stretches to its breaking point. Osmotic Pressure And The Hydrostatic Pressure Tonicity Tonicity: refers to the ability of a solution to change the shape ( or plasma membrane tension) of cells by altering the cells' internal water volume. Isotonic solutions have the same concentrations of non-penetrating solutes as those found in (0.9% saline or 5% glucose). Cells exposed to isotonic solutions retain their normal shape, and exhibit no net gain of water Hypertonic solutions have a higher concentration of non-penetrating solutes than seen in the cell (for example, a strong saline solution). Cells immersed in hypertonic solutions lose water and shrink. Hypotonic solutions are more dilute (contain a lower concentration of non-penetrating solutes) than cells. Cells placed in a hypotonic solution plump up rapidly as water rushes into them. Distilled water represents the most extreme example of hypotonicity. Because it contains no solutes, water continues to enter cells until they finally burst. The effect of solutions of varying tonicities on living red blood cells. To summaries Active membrane transport • Directly or indirectly uses ATP. • An active process occurs whenever a cell uses energy to move solutes across the membrane. • The substance may be too large to pass through the channels, incapable of dissolving in the lipid bilayer, or moving against its concentration gradient. • There are two major means of active membrane transport: a) Active transport and b) Vesicular transport. Active Transport • Like carrier-mediated facilitated diffusion, Active transport requires transport proteins that combine specifically and reversibly with the transported substances. • Active transporters move solutes, most importantly ions, "uphill" against a concentration gradient. To do this work, cells must expend energy. • Active transport processes are distinguished according to their source of energy: a) Primary active transport, the energy to do work comes directly from hydrolysis of ATP by transport proteins called pumps. b) Secondary active transport, transport is driven by energy stored in concentration gradients of ions created by primary active transport pumps. Secondary active transport systems always move more than one substance at a time using a cotransport protein. Primary active transport • Primary active transport is the process in which solutes are moved across cell membranes against electrochemical gradients using energy supplied directly by ATP. • The action of the Na+ -K+ pump is an important example of primary active transport. Secondary active transport Secondary active transport is driven by the concentration gradient created by primary active transport. *Symport system: the two transported substances move in the same direction, Na- Glucose. *Antiport system: the transported substances "wave to each other" as they cross the membrane in opposite directions, Na-H. Vesicular Transport • In vesicular transport, fluids containing large particles and macromolecules are transported across cellular membranes inside bubble-like, membranous sacs called vesicles. • Vesicular transport moves substances into the cell (endocytosis) and out of the cell (exocytosis). • It is also used for combination processes such as transcytosis and vesicular trafficking. • Vesicular transport processes are energized by ATP (or in some cases another energy-rich compound, GTP-guanosine triphosphate) Endocytosis Events of endocytosis. Note the three possible fates for a vesicle and its contents. Endocytosis Three types of endocytosis differ in the type and amount of material taken up and the means of uptake. These are a)phagocytosis, b)pinocytosis, c)receptor-mediated endocytosis Exocytosis • Vesicular transport processes that eject substances from the cell interior into the extracellular fluid are called exocytosis. • Exocytosis is typically stimulated by a cell-surface signal such as binding of a hormone to a membrane receptor or a change in membrane voltage. • Exocytosis accounts for hormone secretion, neurotransmitter release, mucus secretion, and in some cases, ejection of wastes. Exocytosis Exocytosis, like other mechanisms in which vesicles are targeted to their destinations, involves a "docking" process in which transmembrane proteins on the vesicles, fancifully called v-SNAREs (v for vesicle), recognize certain plasma membrane proteins, called t-SNAREs (t for target), and bind with them. This binding causes the membranes to "corkscrew" together and fuse, rearranging the lipid monolayers without mixing them To summaries The Active Membrane Transport Processes The membrane potential • Selective diffusion establishes the membrane potential. • While all body cells have a membrane potential, it is especially important for nerve and muscle cells because they use changes in membrane potential as a form of communication. • In their resting state, plasma membranes of all body cells exhibit a resting membrane potential that typically ranges from - 50 to - 90 millivolts (mV), depending on cell type. • For this reason, all cells are said to be electrically polarized. • The minus sign before the voltage indicates that the inside of the cell is negative compared to its outside. • K+ and protein anions predominate inside body cells, and the extracellular fluid contains relatively more Na+, which is largely balanced by Cl-. The key role of K+ in generating the resting membrane potential. • The resting membrane potential is largely determined by K+ because at rest, the membrane is much more permeable to K+ than Na+. • The active transport of sodium and potassium ions by the Na+-K+ pump maintains the Na+ and K+ concentration gradients. Cell adhesion molecules and membrane receptors • allow the cell to interact with its environment • Cells interact with extracellular molecules that act as signposts to guide cell migration during development and repair. • The most important molecules cells use to interact with their environment fall into two large families-cell adhesion molecules and plasma membrane receptors. Roles of Cell Adhesion Molecules (CAMs) • Thousands of cell adhesion molecules (CAMs) are found on almost every cell in the body. • CAMs play key roles in embryonic development and wound repair and in immunity. • The molecular Velcro® that cells use to anchor themselves to molecules in the extracellular space. • The "arms" that migrating cells use to haul themselves past one another • SOS signals sticking out from the blood vessel lining that rally protective white blood cells to a nearby infected or injured area. • Mechanical sensors that transmit information about changes in the extracellular matrix into the cell. Roles of Plasma Membrane Receptors • A huge and diverse group of integral proteins that serve as binding sites are collectively known as member receptors. • Some function in contact signaling, and others in chemical signaling. Most are glycoproteins. • Contact signaling, in which cells come together and touch, is the means by which cells recognize one another. It is particularly important for normal development and immunity. Some bacteria and other infectious agents use contact signaling to identify their "preferred" target tissues. • Many signals originating outside of the cell are in the form of chemical messengers. Chemical signaling is the process in which a ligand, the chemical messenger, binds a specific receptor and initiates a response. Ligands include most neurotransmitters (nervous system signals), hormones (endocrine system signals), and paracrine (chemicals that act locally and are rapidly destroyed). Chemical messengers G proteins act as middlemen or relays between extracellular first messengers and intracellular second messengers that cause responses within the cell. Cytoplasm • Its the cellular material between the plasma membrane and the nucleus, is the site of most cellular activities. Although early microscopists thought that the cytoplasm was a structure less gel, the electron microscope reveals that it consists of three major elements: the cytosol, organelles, and inclusions. • The cytosol is the viscous, semitransparent fluid in which the other cytoplasmic elements are suspended. It is a complex mixture with properties of both a colloid and a true solution. Dissolved in the cytosol, which is largely water, are proteins, salts, sugars, and a variety of other solutes. Cytoplasm • Inclusions are chemical substances that may or may not be present, depending on cell type. Examples include stored nutrients, such as the glycogen granules in liver and muscle cells; lipid droplets in fat cells; and pigment (melanin) granules in certain skin and hair cells. • Organelles are the metabolic machinery of the cell. Each type of organelle carries out a specific function for the cell, some synthesize proteins, others generate ATP, and so on. Cytoplasmic organelles each perform a specialized task Cytoplasmic organelles each perform a specialized task Mitochondria • Are typically threadlike or lozenge-shaped membranous organelles. • In living cells they wriggle, elongate, and change shape almost continuously. • They are the power plants of a cell, providing most of its ATP supply. • The density of mitochondria in a particular cell reflects that cell's energy requirements, and mitochondria generally cluster where the action is. • Busy cells like kidney and liver cells have hundreds of mitochondria, whereas relatively inactive cells (such as certain lymphocytes) have just a few. Mitochondria A mitochondrion is enclosed by two membranes: 1. The outer membrane is smooth and featureless, 2. but the inner membrane folds inward, forming shelflike cristae that protrude into the matrix. 3. The gel-like substance matrix, teams of enzymes, some dissolved in the mitochondrial matrix and others forming part of the cristae membrane, breakdown intermediate products of food fuels (glucose and others) to water and carbon dioxide. 4. multistep mitochondrial process is called aerobic cellular respiration because it requires oxygen. Creating Ph group which change ADP to ATP 5. They contain their own DNA, RNA, and ribosomes and are able to reproduce themselves. 6. When cellular requirements for ATP increase, the mitochondria synthesize more cristae or simply pinch in half (a process called fission) to increase their number Ribosomes • Are small, dark-staining granules composed of proteins and a variety of RNAs called ribosomal RNAs. • Each ribosome has two globular subunits that fit together like an acorn • Ribosomes are sites of protein synthesis: 1. Free ribosomes float freely in the cytosol. They make soluble proteins that function in the cytosol, as well as those imported into mitochondria and some other organelles. 2. Membrane-bound ribosomes are attached to membranes, forming a complex called the rough endoplasmic reticulum. They synthesize proteins destined either for incorporation into cell membranes or lysosomes, or for export from the cell. ***Ribosomes can switch back and forth between these two functions Endoplasmic Reticulum (ER) • Is an extensive system of interconnected tubes and parallel sacs called cisterns. • The cavities of cisterns are filled with fluid. Coiling and twisting through the cytosol. • The ER is continuous with the outer nuclear membrane and accounts for about half of the cell 's membranes. • There are two distinct varieties: rough ER and smooth ER. Endoplasmic Reticulum (ER) Rough ER • The external surface of the is studded with ribosomes, which is why it is called "rough“. • Proteins assembled on these ribosomes thread their way into the fluid-filled interior of the ER cisterns • When complete, the newly made proteins are enclosed in vesicles for their journey to the Golgi apparatus where they undergo further processing. • The rough ER has several functions: 1. Its ribosomes manufacture all proteins secreted from cells. For this reason, the rough ER is particularly abundant and well-developed in most secretory cells, antibody-producing immune cells, and liver cells, which produce most blood proteins. 2. It is also the cell's "membrane factory" where integral proteins and phospholipids that form part of all cellular membranes are manufactured. Within the cisterns, sugar groups are attached to those proteins that will eventually face the extracellular environment. Smooth ER • Is continuous with the rough ER and consists of tubules arranged in a looping network. • Its enzymes play no role in protein synthesis. Instead, the enzymes catalyze reactions involved with the following tasks: 1. Metabolize lipids, synthesize cholesterol and phospholipids, and synthesize the lipid components of lipoproteins (in liver cells) 2. Synthesize steroid-based hormones such as sex hormones (for example, testosterone-synthesizing cells of the testes are full of smooth ER) 3. Detoxify drugs, certain pesticides, and cancer-causing chemicals (in liver and kidneys) 4. Break down stored glycogen to form free glucose (in liver cells especially) 5. Store calcium ions in most cell types [skeletal and cardiac muscle cells] have an elaborate smooth ER (called the sarcoplasmic reticulum) Golgi Apparatus • The Golgi apparatus consists of stacked and flattened membranous sacs, shaped like hollow dinner plates, associated with groups of tiny membranous vesicles • Golgi apparatus is the principal "traffic director" for cellular proteins. Its major function is to modify, concentrate, and package the proteins and lipids made at the rough ER and destined for export from the cell. Processing and distribution of newly synthesized proteins. Peroxisomes • Resembling small lysosomes, are spherical membranous sacs containing a variety of powerful enzymes, the most important of which are oxidases and catalases. • Oxidases use molecular oxygen (02) to detoxify harmful substances, including alcohol and formaldehyde. Their most important function is to neutralize free radicals, highly reactive chemicals with unpaired electrons that can scramble the structure of biological molecules. • Free radicals and hydrogen peroxide are normal by-products of cellular metabolism, but they have devastating effects on cells if allowed to accumulate. • Peroxisomes are especially numerous in liver and kidney cells, which are very active in detoxification. also play a role in energy metabolism by breaking down and synthesizing fatty acids. • Most new peroxisomes form by budding off of the ER via a special machinery that differs from that used for vesicles destined for modification in the Golgi apparatus. Lysosomes • Are spherical membranous organelles containing activated hydrolytic (digestive) enzymes. • Lysosomes are large and abundant in phagocytes, the cells that dispose of invading bacteria and cell debris. • Lysosomal enzymes can digest almost all kinds of biological molecules. • They work best in acidic conditions and so are called acid hydrolases. • The lysosomal membrane is quite stable, but it becomes fragile when the cell is injured or deprived of oxygen and when excessive amounts of vitamin A are present. • When lysosomes rupture, the cell digests itself, a process called Autolysis • The lysosomal membrane is adapted to serve lysosomal functions in two important ways. First, it contains H+ (proton) "pumps," which are ATPases that gather hydrogen ions from the surrounding cytosol to maintain the organelle's acidic pH. Second, it retains the dangerous lysosomal enzymes (acid hydrolases) while permitting the final products of digestion to escape so that they can be used by the cell or excreted. Lysosomes function as a cell's "demolition crew" by: 1. Digesting particles taken in by endocytosis, particularly ingested bacteria, viruses, and toxins 2. Degrading stressed or dead cells and worn-out or nonfunctional organelles, a process more specifically called autophagy 3. Performing metabolic functions, such as glycogen breakdown and release 4. Breaking down bone to release calcium ions into the blood. The endomembrane system • Is a system of organelles that work together mainly to (1) produce, degrade, store, and export biological molecules, and (2) degrade potentially harmful substances. • It includes the ER, Golgi apparatus, secretory vesicles, and lysosomes, as well as the nuclear envelope-that is, all of the membranous elements that are either structurally connected or arise via forming or fusing transport vesicles • The nuclear envelope is directly connected to the rough and smooth ER • The plasma membrane, though not actually an endo-membrane, is also functionally part of this system. The cytoskeleton • "cell skeleton," is an elaborate network of rods running through the cytosol and hundreds of accessory proteins that link these rods to other cell structures. • It acts as a cell's "bones," “muscles," and "ligaments" by supporting cellular structures and providing the machinery to generate various cell movements. • The three types of rods in the cytoskeleton are microfilaments, intermediate filaments, and microtubules. None of these is membrane covered. Cytoskeleton Microtubules | Cell Biology - YouTube The cytoskeleton Actin filaments push or pull on the plasma membrane Strongly resist tension and attached to desmosomes Centrosome or cell center, determine the cell shape, and distribution of organelles. Organelles move by motor proteins Centrosome and Centrioles • The centrosome acts as a Microtubule Organizing Center. • The centrosome matrix is best known for generating microtubules and organizing the mitotic spindle in cell division. • It has few distinguishing marks other than a granular-looking matrix that contains paired centrioles, small, barrel-shaped organelles oriented at right angles to each other. • Each centriole consists of a pinwheel array of nine triplets of microtubules, each connected to the next by nontubulin proteins and arranged to form a hollow tube. • Centrioles also form the bases of cilia and flagella. Cilia and microvilli are two main types of cellular extensions • Cilia are whip like, motile cellular extensions that occur in large numbers, on the exposed surfaces of certain cells. • Ciliary action moves substances in one direction across cell surfaces. For example, ciliated cells that line the respiratory tract propel mucus upward away from the lungs. • Cilia is made when the centrioles multiply and line up beneath the plasma membrane at the cell 's free surface. Microtubules then "sprout" from each centriole, forming the ciliary projections by exerting pressure on the plasma membrane. • Centrioles forming the bases of cilia and flagella are referred to as basal bodies. • As a cilium moves, it alternates rhythmically between a propulsive power stroke, when it is nearly straight and moves in an arc, and a recovery stroke, when it bends and returns to its initial position. • Flagella are also projections formed by centrioles, but are substantially longer than cilia. The only flagellated cell in the human body is a sperm, which has one propulsive flagellum, commonly called a tail. Cilia *** Cilia propel other substances across a cell 's surface, whereas a Flagellum propels the cell itself. How Do Cilia and Flagella Move? – YouTube Microvilli • Are tiny, fingerlike extensions of the plasma membrane that project from an exposed cell surface. • They increase the plasma membrane surface area and are most often found on the surface of absorptive cells such as intestinal and kidney tubule cells. • Microvilli have a core of bundled actin filaments that extend into a mat of actin filaments, called the terminal web, near the surface of the cell. • Actin is often a contractile protein, but in microvilli and the Terminal Web it acts as a mechanical "stiffener" that shapes the cell. Nucleus • For cells, the control center is the gene-containing nucleus • The nucleus is the genetic library, it contains the instructions needed to build nearly all the body's proteins. • Additionally, it dictates the kinds and amounts of proteins to be synthesized at any one time in response to signals acting on the cell. • Most cells have only one nucleus, but some, including skeletal muscle cells, bone destruction cells, and some liver cells, are multinucleate, they have many nuclei. • The presence of more than one nucleus usually signifies that a larger-than-usual cytoplasmic mass must be regulated. • Anucleated cells cannot reproduce and therefore live in the bloodstream for only three to four months before they deteriorate. • Without a nucleus, a cell cannot produce mRNA to make proteins, and when its enzymes and cell structures start to break down they cannot be replaced. Nucleus The nucleus includes the nuclear envelope, the nucleolus, and chromatin • the Nuclear Envelope, a double membrane barrier separated by a fluid-filled space. The outer nuclear membrane is continuous with the rough ER of the cytoplasm and is studded with ribosomes on its external face. The inner nuclear membrane is lined by the nuclear Lamina, a network of laminas (rodshaped proteins that assemble to form intermediate filaments) that maintains the shape of the nucleus and acts as a scaffold to organize DNA in the nucleus. • Nuclear envelope is punctured by nuclear pores. Proteins, called a nuclear pore complex, lines each pore, forming an aqueous transport channel and regulating entry and exit of molecules (e.g., mRNAs) and large particles into and out of the nucleus. • Nuclear envelope is selectively permeable, but here substances pass much more freely than elsewhere. Small molecules pass through the relatively large nuclear pore complexes unhindered. Protein molecules imported from the cytoplasm and RNA molecules exported from the nucleus move through the central channel of the pores. This process is energy dependent and guided by soluble transport proteins. The nuclear envelope encloses a jellylike fluid called nucleoplasm Nucleoli • Dark-staining spherical bodies where the ribosomal subunits are assembled. Nucleoli are NOT membrane bounded. • Typically, there are one or two per nucleus, but there may be more. • Nucleoli are usually largest in growing cells that are making large amounts of tissue proteins. • Nucleoli are aggregations of all of the components needed to synthesize and assemble ribosomal subunits. • They center around the DNA that codes for ribosomal RNA (rRNA). • As rRNA molecules are synthesized, they combine with proteins to form the two kinds of ribosomal subunits. (The proteins are manufactured on ribosomes in the cytoplasm and imported into the nucleus.) • The finished subunits leave the nucleus through the nuclear pores and enter the cytoplasm, where they join to form functional ribosomes. Chromatin • Chromatin appears as a fine, unevenly stained network, but special techniques reveal it as a system of bumpy threads weaving through the nucleoplasm. Chromatin is composed of approximately: • 30% DNA, our genetic material • 60% globular Histone proteins which package and regulate the DNA • 10% RNA chains, newly forming. • The fundamental units of chromatin are Nucleosomes, which consist of flattened discshaped cores of eight histone proteins connected like beads on a string by a DNA molecule. • The DNA twice around each nucleosome and continues on to the next cluster via linker DNA segments • Histones provide a physical means for packing the very long DNA molecules (2 meters) in a compact and orderly way, but they also play an important role in gene regulation. • In a non-dividing cell, for example, the presence of Methyl Groups on histone proteins shuts down the nearby DNA. As another example, addition of Acetyl Groups to histone exposes different DNA segments, or genes, so that they can dictate the specifications for synthesizing proteins or various RNA species. Chromatin • Active chromatin segments, referred to as extended chromatin, are NOT usually visible under the light microscope. • The generally inactive condensed chromatin segments are darker staining and more easily detected. • The most active body cells have much larger amounts of extended chromatin. • When a cell is preparing to divide, the chromatin threads coil and condense enormously to form short, bar-like bodies called Chromosomes • Chromosome compactness prevents the chromatin strands from tangling and breaking during the movements that occur during cell division. The cell cycle • The cell cycle is the series of changes a cell goes through from the time it is formed until it reproduces. It has two main phases: • Interphase, in which the cell grows and carries on its usual activities • Cell division or the mitotic phase, during which it divides into two cells Interphase • Is the period from cell formation to cell division. Early cytologists, unaware of the constant molecular activity in cells and impressed by the obvious movements of cell division, called interphase the resting phase of the cell cycle. • However, it can be misleading because during interphase a cell is carrying out all its routine activities and is "resting" only from dividing. Perhaps a more accurate name for this phase would be metabolic phase or growth phase. Subphases: lnterphase is divided into G1, S, and G2 subphases (the Gs stand for gaps before and after the S phase, and S is for synthetic) G1 (gap 1 subphase): The cell is metabolically active, synthesizing proteins rapidly and growing vigorously. This is the most variable phase in terms of length. G1 typically lasts several minutes to hours sometimes days or even years. As G1 ends, the centrioles start to replicate in preparation for cell division. S phase: DNA is replicated, ensuring that the two future cells being created will receive identical copies of the genetic material. New histones are made and assembled into chromatin. One thing is sure, without a proper S phase, there can be no correct mitotic phase. G2 (gap 2 subphase): The final phase of interphase is brief. Enzymes and other proteins needed for division are synthesized and moved to their proper sites. By the end of G2, centriole replication. At the end of this phase is the G2/M checkpoint when the cell ensures that all DNA is replicated and damaged DNA has been repaired. If so, the cell is now ready to divide. DNA Replication https://www.youtube.com/watch?v=TNKWgcFPHqw DNA Replication DNA must be replicated exactly, so that identical copies of the cell's genes can be passed to each of the two resulting daughter cells. Replication involves the following sequence of events: 1. Uncoiling: Enzymes forming a replication bubble. 2. Separation: the hydrogen bonds between base pairs are broken. Is known as the replication fork. 3. Assembly: the enzyme DNA polymerase positions complementary free nucleotides along the template strands. Since each new molecule consists of one old and one new nucleotide strand, this mechanism is known as semiconservative replication. 4. Restoration: Ligase enzymes splice short segments of DNA together, restoring the double helix structure. ***During the replication process, histones (made in the cytoplasm and imported into the nucleus) associate with the DNA, completing the formation of two new chromatin strands. Cell Division In most body cells, cell division, which is called the M (mitotic phase) of the cell cycle, involves two distinct events: mitosis and cytokinesis: • Mitosis the division of the nucleus, is the series of events that parcels out the replicated DNA of the parent cell to two daughter cells. Described as four phasesprophase, metaphase, anaphase, and telophase-mitosis is actually a continuous process. In human cells it typically lasts about an hour or less. • Cytokinesis the division of the cytoplasm, begins during late anaphase and is completed after mitosis ends. A contractile ring made of actin filaments draws the plasma membrane inward to form a cleavage trough over the center of the cell. The furrow deepens until it pinches the cytoplasmic mass into two parts, yielding two daughter cells. Each is smaller and has less cytoplasm than the parent cell, but is genetically identical to it. Mitosis is the process of nuclear division in which the chromosomes are distributed to two daughter nuclei. Together with cytokinesis, it produces two identical daughter cells. Control of Cell Division • The ratio of cell surface area to cell volume. As a cell grows, its volume increases more rapidly than its surface area. • Chemical signals such as growth factors and hormones released by other cells. • The availability of space. Normal cells stop proliferating when they begin touching, a phenomenon known as contact inhibition Gene • Gene is a segment of a DNA molecule that carries instructions for creating one polypeptide chain. (Note, however, that some genes specify the structure of certain varieties of RNA as their final product.) • The four nucleotide bases adenine (A), cytosine (C), guanine (G), and thymine (T). are the "letters" of the genetic alphabet, and the information of DNA is found in the sequence of these bases. • Each sequence of three bases, called a triplet, can be thought of as a "word" that specifies a particular amino acid. For example, the triplet AAA calls for the amino acid phenylalanine. • It specifies the number, kinds, and order of amino acids needed to build a particular polypeptide. • Variations in the arrangement of A, T, C, and G allow our cells to make all the different kinds of proteins needed. The ratio between DNA bases in the gene and amino acids in the polypeptide is 3: 1 (because each triplet stands for one amino acid) Gene • Our genes consist of long sequences of DNA, only some of which code for protein. • The coding regions are called Exons. Exons are separated from each other by long intervening sequences called introns. • At first glance, introns may seem wasteful. However, they can act as control elements and they can allow for making different (related) proteins from one gene by omitting or including certain exons. The Role of RNA in Protein Synthesis • RNA is single stranded, and it has the sugar ribose instead of deoxyribose, and the base uracil (U) instead of thymine (T). Three forms of RNA typically act together to carry out DNA's instructions for polypeptide synthesis: • Messenger RNA (mRNA), relatively long nucleotide strands resembling "half-DNA" molecules. mRNA carries the coded information to the cytoplasm, where protein synthesis occurs. • Ribosomal RNA (rRNA), along with proteins, forms the ribosomes, which consist of two subunits-one large and one small. The two subunit types combine to form functional ribosomes, which are the sites of protein synthesis. • Transfer RNA (tRNA), small, roughly L-shaped molecules that ferry amino acids to the ribosomes. There they decode mRNA's message for amino acid sequence in the polypeptide to be built. ***Because rRNA and tRNA do not transport codes for synthesizing other molecules, they are the final products of the genes that code for them. They act together to "translate" the message carried by mRNA. Polypeptide synthesis involves two major steps: 1. Transcription, in which DNA's information is encoded in mRNA 1. Translation, in which the information carried by mRNA is decoded and used to assemble polypeptides Figure: summarizes the information flow in these two major steps. It indicates the "RNA processing" that removes intrans from mRNA before this molecule moves into the cytoplasm. Transcription Translation Translation is the process in which genetic information carried by an mRNA molecule is decoded in the ribosome to form a particular polypeptide. The "translators" are tRNA molecules that can recognize and bind specifically both to an mRNA codon and an amino acid. Translation occurs in three phases: initiation, elongation, and termination. Translation DNA transcription and translation McGraw Hill - YouTube Rough ER processing of proteins. An signal sequence in a newly forming protein causes the signal recognition particle (SRP) to direct the mRNAribosome complex to the rough ER. Other Roles of DNA • Other Roles of DNA The story of DNA doesn't end with the production of proteins encoded by exons. Scientists are finding that DNA also codes for a surprising variety of active RNA species that are not translated into proteins, including the following: • MicroRNAs (miRNAs) are small RNAs that can interfere with and suppress mRNAs made by certain exons, effectively silencing them. • Small interfering RNAs (siRNAs) are like miRNA but originate outside the cell. For example, our cells can use an infecting virus's RNA to create siRNAs that then interfere with viral replication. References and Further Reading • Elaine N. Marieb and Katja N. Hoehn (2019). Human Anatomy and Physiology. 11th Edition. Pearson Education. Chapter 3