Topic 1 Introduction to Spine PDF
Document Details
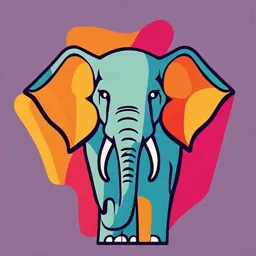
Uploaded by EnergeticOrphism
Universiti Kuala Lumpur Royal College of Medicine Perak
Tags
Summary
This document is a lecture or presentation on the Introduction to Spine, emphasizing physiotherapy in spine rehabilitation. It covers osteokinematics, arthrokinematics, and spinal structure/function, along with specific examples like spinal compression, bending, and torsion. This document is from Universiti Kuala Lumpur, Royal College of Medicine Perak, RFB 30302.
Full Transcript
TOPIC 1 INTRODUCTION TO SPINE PHYSIOTHERAPY IN SPINE REHABILITATION RFB 30302 Learning outcome At the end of this topic you can able to: 1. Explain the osteokinematics. 2. Explain the arthrokinematics of spine. 3. Structure and function of the spine. ...
TOPIC 1 INTRODUCTION TO SPINE PHYSIOTHERAPY IN SPINE REHABILITATION RFB 30302 Learning outcome At the end of this topic you can able to: 1. Explain the osteokinematics. 2. Explain the arthrokinematics of spine. 3. Structure and function of the spine. Osteokinematics Osteokinematics describes clear movements of bone which are visible from the outside. They are the gross movement that happens between two bones. They arise from rotation around the joint axis. Some examples are: Flexion and extension Abduction and adduction Lateral rotation and medial rotation AXIAL COMPRESSION Force acting through the long axis of the spine at right angles to the disks. Occurs as a result of the Force of gravity Ground reaction forces Forces produced by the ligaments and muscular contractions The disks and vertebral bodies resist most of the compressive force. BENDING Bending causes both compression and tension on the structures of the spine. In forward flexion: Anterior structures (anterior portion of the disk, anterior ligaments, and muscles) are subjected to compression; Posterior structures are subjected to tension. Resistance offered to the tensile forces by: Collagen fibers in the posterior outer annulus fibrosus, zygapophyseal joint capsules, and posterior ligaments. Help to limit extremes of motion and provide stability in flexion. TORSION Torsional forces are created during axial rotation that occurs as a part of the coupled motions that take place in the spine. This kind of force tries to twist the bone along its long axis Torsional forces generally result in short or long spiral fractures SHEAR Shear forces act on the mid plane of the disk and tend to cause each vertebra to undergo translation move anteriorly, posteriorly, or from side to side in relation to the inferior vertebra In the lumbar spine, Zygapophyseal joints resist some of the shear force, and the Disks resist the remainder. ARTHROKINEMATICS OF SPINE ARTHROKINEMATICS is the general term for the specific movements of joint surfaces. Normal joint surface movement is necessary to ensure long-term joint integrity. (Joint surface movements are sometimes called joint play motions or component motions). Joint surfaces move with respect to one another by simultaneously Rolling. Gliding. Spinning. Structure of the Spine What is the vertebral column? a curved stack of 33 vertebrae structurally divided into five regions: cervical region - 7 vertebrae thoracic region - 12 vertebrae lumbar region - 5 vertebrae sacrum - 5 fused vertebrae coccyx - 4 fused vertebrae Picture retrieved from http://www.becomehealthynow.com/images/organs/spine/ant_lat.jpg Structure of the Spine What is a motion segment? two adjacent vertebrae and the associated soft tissues considered the functional unit of the spine Structure of the Spine Transverse process Posterior Interspinous longitudinal ligament Anterior ligament Supraspinous longitudinal ligament ligament Vertebral Cartilaginous body end-plate Intervertebral joint and facet Intervertebral Spinous disc process Intervertebral Ligamentum foramen with flavum Vertebral canal nerve root Posterior Anterior Two adjacent vertebrae and the associated tissues comprise the motion segment. Structure of the Spine What are the joints between adjacent vertebrae? intervertebral symphysis joints on the anterior side two gliding diarthrodial facet joints on the posterior side Structure of the Spine What is the function of the facet joints? to channel and limit the range of motion in the different regions of the spine to assist in load bearing, sustaining up to 30% of the compressive load on the spine, particularly when the spine is in hyperextension Structure of the Spine What are the intervertebral discs? fibrocartilaginous discs that cushion the anterior spinal symphysis joints composed of a nucleus pulposus (colloidal gel with a high fluid content) surrounded by the annulus fibrosus (a thick, fibrocartilaginous ring that forms the disk exterior) Picture retrieved from http://upload.wikimedia.org/wikipedia/commons/6/62/Gray94.png Lumbar Vertebra Picture retrieved from http://upload.wikimedia.org/wikipedia/commons/b/b2/Gray430.png Movements of the Spine What movements of the spine are allowed? The movement capabilities of the spine are those of a ball and socket joint, including movement in all three planes and circumduction. Mechanically: Annulus fibrous – acts like coiled spring Nucleus pulposus – acts like ball bearing Movements of the Spine What muscles contribute to flexion of the spine in the abdominal region? rectus abdominis internal obliques external obliques Picture retrieved from http://upload.wikimedia.org/wikipedia/common s/3/32/Illu_trunk_muscles.jpg Movements of the Spine What muscles contribute to extension of the spine in the thoracic and lumbar regions? erector spinae - spinalis, longissimus, iliocostalis semispinalis - capitis, cervicis, thoracis deep spinal muscles - mulitifidi, rotatores, interspinales, intertransversarii, levatores costarum Picture retrieved from http://upload.wikimedia.org/wikipedia/common s/9/90/Gray389.png Movements of the Spine What muscles contribute to lateral flexion of the lumbar spine? quadratus lumborum psoas major PLUS the lumbar flexors and extensors when developing tension unilaterally Loads on the Spine What forces commonly act on the spine? body weight tension in the spinal ligaments tension in the spinal muscles any external loads carried in the hands Loads on the Spine In normal standing position, body weight acts anterior to the spine, creating a forward bending load (moment) on the spine. Loads on the Spine Because the spine is curved, body weight, acting vertically, has components of both compression (Fc) and shear (Fs) at most motion segments. Fs Fc wt Loads on the Spine Muscle tension During lifting, both Shear compression and reaction force anterior shear act on the Joint spine. Tension in the center spinal ligaments and Compression muscles contributes to reaction force compression. Sample Problem 1 How much tension must be developed by the erector spinae with a moment arm of 6cm from L5-S1 joint center to maintain the body in the position shows on your right? Given that Head : 58 N Arms : 81 N Trunk : 328 N Box : 111 N Weight of the head: 58 N Weight of the arms: 81 N Weight of the trunk: 328 N Weight of the box: 111 N Distance from L5-S1 to the head’s force: 40 cm (0.40 m) Distance from L5-S1 to the arms’ force: 25 cm (0.25 m) Distance from L5-S1 to the trunk’s force: 20 cm (0.20 m) Distance from L5-S1 to the box’s force: 40 cm (0.40 m) Moment arm of the erector spinae: 6 cm (0.06 m) Step 1: Calculate the Moments for Each Force Each force creates a moment about the L5-S1 joint: Moment=Force×Distance Head moment: 58 N×0.40 m=23.2 Nm Arms moment: 81 N×0.25 m=20.25 Nm Trunk moment: 328 N×0.20 m=65.6 Nm Box moment: 111 N×0.40 m=44.4 Nm Step 2: Calculate the Total Moment Acting on L5-S1 Total Moment=23.2+20.25+65.6+44.4=153.45 Nm Step 3: Calculate the Tension in the Erector Spinae The tension in the erector spinae must generate an equal and opposite moment to maintain equilibrium. T×0.06 m=153.45 Nm T=153.45 Nm/0.06 m=2557.5 N Final Answer: The tension that must be developed by the erector spinae muscles is 2557.5 N. Loads on the Spine compression tension Lumbar hyperextension can create a bending load (moment) in the posterior direction. Loads on the Spine Lumbar hyperextension produces compressive loads at the facet joints. hyperextension Loads on the Spine Superior view Lateral view Spinal rotation generates shear stress in the intervertebral discs. Human Posture When Lifting Common Injuries Low back pain Soft tissue Injuries Acute fractures Stress Fracture – spondylolysis (fracture in vertebral neural arch) Disc herniation CERVICAL SPINE CERVICAL Consist of seven vertebrae Two distinct unit within the cervical vetebral column may be describe i) craniovetebral / suboccipital ( atlas and axis) ii) lower cervical C3-C7 CERVICAL SPINE The cervical spine supports the head, provides attachment for muscles of the neck and upper extremity, and, along with the rest of the spine, protects the spinal cord. It must meet the demand of providing a large range of motion (ROM) to ensure optimal functioning of the special senses such as vision, smell and hearing that are housed in the head. Atlas (C1) Between skull and lower cervical spine Function : 1. to cradle the occiput and to transmit forces from the head to the cervical spine 2. Adapted for attachment of ligaments and muscles superior articular facet on lateral mass - contain kidney shaped articular facets for the occiput and form a ring by anterior and posterior arches The superior aspect of each lateral mass exhibits a deep socket that is concave anteroposteriorly and mediolaterally, consistent with the curvature of the occipital condyles so that the skull rests securely on the atlas. The size and shape of the sockets vary greatly, but in general, the articular surfaces of these superior facets are directed upward and medially, with their outer margins projecting more superiorly. In long, deeply concave sockets, the anterior wall may face backward and the posterior wall forward. In most C1 vertebrae, each socket is completely or incompletely divided into two facets or into a dumbbell-shaped facet having a nonarticular waist. The occipital condyles transmit the weight of the head to the axis (C2) via the large lateral masses of the atlas (C1). The robust transverse processes of the atlas are the primary site of muscle attachment for this vertebra. The size of each transverse process accommodates the loading associated with suspension of the scapula through the attachment of the levator scapulae muscle. Consequently, any movement of the upper limb exerts compressive forces on the entire cervical spine. The length of each transverse process increases the moment arms of the muscles attached to it but also allows the vertebral artery to clear the large lateral masses of the axis below. The anterior arch that joins the lateral masses of the atlas is short and slender, since it is uninvolved in transmitting large forces. A small, smooth facet lies centrally on the posterior aspect of the anterior arch for articulation with the odontoid process of the axis. The position of the anterior arch against the odontoid process ensures that there is a bony block to posterior translation of the atlas. Enclosed by the anterior and posterior arches and lateral masses, the central foramen of the atlas has two distinct parts. The smaller anterior part partially encircles the odontoid process, or dens, while the larger posterior portion is the vertebral foramen proper Axis (C2) The axis accepts the load of the head and atlas and transmits that load to the remainder of the cervical spine. It also provides axial rotation of the head and atlas. The broad, laterally placed, superior articular facets of the axis accept and transmit loads from the head and atlas, while the centrally placed odontoid process, or dens, acts as a pivot around which the anterior arch of the atlas spins and glides to produce axial rotation. C2 The superior articular facets of the axis are lateral to the dens and face upward and laterally and slope inferiorly and laterally. They support the lateral masses of the atlas and transmit the load of the head and atlas inferiorly and anteriorly to the C2–C3 intervertebral disc and inferiorly and posteriorly to the C2–C3 zygapophysial, or facet, joints. The inferior articular facet is located posterior to the superior facet in a position similar to the articular processes of the lower cervical vertebrae Laminae: broad and robust - meeting at a broad, roughened spinous process. Spinous process: broad, divided into 2. Like the transverse processes of the lower cervical vertebrae, each transverse process of the atlas and axis contains a transverse foramen that, with the other foramina on the same side, form a canal through which the vertebral artery travels on its way to the foramen magnum. Column C3–C7 Vertebrae Support the axial load of the head and vertebrae Keep the head upright Support the reactive forces of muscles Provide mobility of the head Column C3–C7 Vertebrae The vertebrae, therefore, exhibit features that reflect these load-bearing, stability, and mobility functions. Together, the lower five vertebrae may be considered as a triangular column consisting of an anterior pillar composed of the vertebral bodies and two posterior columns composed of the right and left articular pillars of the articulating superior and inferior articular processes A- ANTERIOR PILLOR B&C- LATERAL PILLORS The vertebral bodies exhibit a modified block like quality reflecting their ability to bear and transmit axial loads. Due to the presence of the uncinate processes, which arise as bony projections along the posterolateral margins of the superior vertebral endplates, the superior surface of the body of a lower cervical vertebra is concave transversely, while in the sagittal plane, the superior surface slopes forward and downward. Inferiorly, the surface of the body is concave anteroposteriorly, with an anterior lip that projects anteroinferiorly toward the anterior superior edge of the vertebra below. Posteriorly, the articular processes bear the superior and inferior articular facets. Generally, the superior facets are directed superiorly and posteriorly, while the inferior facets are directed anteriorly and inferiorly). The orientation of the facets contributes to the function of each vertebra. In the upright posture, the superior facet lies between the transverse and frontal planes, and as a consequence, it helps support the weight of the head and stabilizes the vertebra above against forward translation. However, at each level, there are subtle differences in orientation of the facets. In addition to facing superiorly and posteriorly, the superior facet of C3 also faces medially by about 40°. The superior articular facets change from a posteromedial orientation at the C2/C3 level to posterolateral orientation at C7/T1. The transition typically occurs at the C5/C6 level. Knowledge of the height of the articular processes is important, as it has been demonstrated that the height of the articular processes is perfectly related to the location of the instantaneous axes of rotation. It is articular height, not slope, that is the major determinant of the patterns of motion of the cervical vertebrae. The unique morphology exhibited by the seventh cervical vertebra reflects its load-bearing function. The superior articular facets sit high relative to the superior surface of the vertebral body and are steeply inclined in the coronal plane. Such geometry appears to provide optimal stability, guarding against forward translation at this transition from the cervical to the thoracic spine. Total Motion of the Cervical Spine Total motion of the cervical spine is typically determined by describing head motion relative to the thorax or shoulder girdle. In addition, age and sex have been associated with variations in neck ROM. Normal variation in ROM in subjects suggests that when measuring individual patients, a clinician should allow for natural variation of 12° to 20°. Atlantoaxial Joints Axial rotation at the atlantoaxial level is extremely important functionally, for movement at this level accounts for 50% of the total range of axial rotation of the neck. Indeed, the first 45° of rotation of the head to either side occurs at the C1– C2 level before any lower cervical segments move in this plane. Axial rotation of the atlas to the left requires anterior displacement of the right lateral mass and a reciprocal posterior displacement of the left lateral mass. The inferior articular cartilages of the atlas must therefore slide down the respective slopes of the convex superior articular cartilages of the axis Limitation of axial rotation is essential, as the spinal cord and vertebral arteries cross this joint. The reported normal range of axial rotation to one side in living subjects is between 37.5° and 49°. In axial rotation of the atlantoaxial joint from the neutral position (A). The inferior articular facet of the atlas slides down the anterior slope of the convex superior facet of the axis during contralateral rotation (B). Down the posterior slope of the convex superior facet of the axis during ipsilateral rotation (C). The shape of the odontoid process allows the anterior arch of the atlas to slide upward and slightly backward, thereby producing extension of the atlas on the axis. Flexion takes place by a downward and forward glide, with an additional slight anterior translation of the anterior arch on the odontoid process. The total range of flexion–extension reported in vivo varies between 11° and 21°. Side bending does not result from pure lateral translation. As the superior articular facets of the axis slope down and laterally, lateral translation would produce impaction of the contralateral lateral mass of the atlas on the superior lateral mass of the axis. Lateral translation and side bending at the lateral atlantoaxial joint A. The superior articulating facets of the axis slope laterally and inferiorly. B. As the atlas translates laterally, its inferior facet impacts on the superior facet on the axis. C. As the atlas translates, one inferior facet slides up on the underlying superior facet as the other rides down, imparting a lateral tilt to the atlas. Segmental Craniovertebral Motion The characteristic movements of the atlanto-occipital and atlantoaxial joints that have been described do not occur in isolation. Rather, these joints of the head, atlas, and axis normally function as a composite unit. As noted previously, the atlas acts essentially as a passive washer, structurally tied to the occipital condyles by joint geometry and soft tissues. Consequently, when the head moves during axial rotation, the head and atlas move in concert on the axis. During flexion and extension of the head and neck, the atlas exhibits what is known as paradoxical motion. For example, during flexion, the atlas may flex or it may extend, and during extension of the neck, the atlas may also flex or it may extend. The equilibrium of the resting position is thus susceptible to small variations in the position of compression forces passing through the lateral masses. If the compression load is exerted anterior to the fulcrum of the articular surfaces of the lateral atlantoaxial joint, the atlas tilts into flexion. If the compression load is exerted posterior to the fulcrum, the atlas tilts into extension. During side bending of the head to the left, the atlas rotates to the right, while the axis rotates to the left. The compressive load of the head passes from the ipsilateral lateral mass of the atlas down to the C2/C3 zygapophysial joint and zygapophysial joints below. Guidance of this motion has also been attributed to the posterior attachments of the alar ligaments onto the odontoid process. It has been postulated that during side bending of the head, the contralateral alar ligament is tensioned. Due to the posterior attachment of the alar ligament on the odontoid process, a forced rotation of the axis occurs in the direction of side bending. Segmental Motion of the Lower Cervical Region The nature and range of segmental motion in the lower cervical spine are influenced by both the geometry of the zygapophysial joints and the morphology of the interbody joints. Pure anterior translation cannot occur because the inferior articular processes of the upper vertebra impact against the superior articular processes of the lower vertebra. Further, translation occurs if the upper vertebra tilts forward, drawing its inferior articular processes up the superior articular processes below. Segmental Motion of the Lower Cervical Region Flexion in the lower cervical spine, therefore, is always a combination of anterior translation and anterior rotation in the sagittal plane. The reverse occurs in extension, with coupling of posterior sagittal rotation and posterior translation. Flexion in the lower cervical spine combines anterior translation and sagittal plane rotation of the superior vertebra. Coupling of motion during rotation in the lower cervical spine Traditionally, axial rotation to the left is described as coupled with ipsilateral side bending resulting from the right inferior facet gliding superiorly on the underlying superior facet. Side bending Traditionally, side bending to the left in the lower cervical spine is described as coupled with ipsilateral rotation resulting from the posterior glide of the left inferior facet on the underlying superior facet The motion of the saddle joint between adjacent lower cervical vertebrae occurs in the plane of the facet joints, about an axis perpendicular to the plane. Superior vertebral body can rock side to side within the concavity of the uncinate processes, pivoting about the anterior annulus fibrosus while the facets slide freely upon one another Sagittal plane view of the head and neck illustrates a flexion moment (M) around the point of rotation, or axis, (O) produced by the weight of the head (W). The weight of the head is applied at the head's center of gravity (CG). The extensor musculature must produce an extension moment (E) to balance the head. LIGAMENT OF THE CRANIOVETEBRAL JOINTS Transverse ligament Alar ligaments Membrana tectoria Atlanto occipital and atlantoaxial membranes Apical ligament LIGAMENTS OF THE LOWER CERVICAL JOINT Longitudinal Ligaments Ligamentum flavum Ligamentum nuchae THORACIC SPINE Structure of the thoracic vertebrae - the longest segment ( 12 separate vertebrae) The upper thoracic spine - 1st – 4th thoracic vertebrae ( inferior surface larger than superior surface Diameter – slightly larger in an anterior posterior direction than in a medial lateral direction Wedge shaped – normal kyphotic curve of the thoracic spine that is characterized by a posterior convexity Thicker posteriorly than anteriorly Normal kyphosis of the thoracic and wedging of vertebrae – large loads applied to the thoracic vertebrae bodies – compression fracture In the thoracic region of the spine, the vertebrae also have costal facets which articulate with the ribs Thoracic spine more structural stability but less flexibility than the cervical and lumbar regions. Movement of the Thoracic spine and thorax Segmental motion Thoracic spine – less segmental mobility than the cervical or lumbar regions Upper and middle : 2-6 degree of combined flexion and extension Flexion and extension increase in the lower thoracic spine in the presence of the vertebral ribs Side bending limited : due to ribs - slightly increase in lower thoracic region – where the ribs have no sternal attachment and provide little barrier to side bending motion Rotation : upper and middle thoracic > lumbar Coupled motion : greater in side bending and rotation Upper thoracic : side bending coupled with ipsilateral rotation Middle and lower : ipsilateral / contralateral motion Differences in segmental mobility : due to orientation of the facet joint Thoracic joint (facet ) ; more vertically aligned , superior facets facing posteriorly , inferior facet facing anteriorly and slightly medially Segmental motion varies throughout the vertebral column. Flexion/extension mobility in the thoracic spine increases from the superior to the inferior segments. Motion of the thoracic spine, like that of the cervical and lumbar regions depends on the orientation of the facet joints and on the thickness of the intervertebral discs. Coupled motion: A coupled motion consists of a primary motion that occurs in one plane and is accompanied automatically by motion in at least one other plane. Although coupling appears to occur in all motions of the vertebral column, it is greatest in side bending and rotation. Coupling appears less in magnitude and more variable in the thoracic spine than in the cervical spine where side bending is coupled primarily with ipsilateral rotation. Superior and lateral views reveal that the thoracic and lumbar vertebral facets are more vertically aligned than are the cervical facets. The cervical and thoracic facets face more anteriorly and posteriorly; the lumbar facets face more medially and laterally. Flexion of the thoracic spine requires anterior translation of the inferior facet, limited by the vertical orientation of the facet and by the facet joint capsule. Total Mobility of the Thoracic Spine Total excursions reported for the thoracic spine are varied, ranging from approximately 20° to almost 50° in the sagittal plane. When measured in the standing position, total flexion mobility is similar to but slightly greater than total extension mobility. RIB CAGE Rib cage attach the vertebral column posteriorly to the sternum anteriorly – limit thoracic movement .There are 12 ribs on each side, for a total of 24. The upper seven : true ribs, attaching directly to the sternum anteriorly. Ribs 8-10 : false ribs because they attach indirectly to the sternum via the costal cartilage of the seventh rib. The 11th and 12th : floating ribs because they have no anterior attachment. Motions of the Ribs with Thoracic Motion Ribs are attached to all of the thoracic vertebrae, movement of these vertebrae also produces rib motion. Flexion and extension of the thoracic spine are accompanied by depression and elevation of the ribs, respectively. Flexion of the thoracic spine causes approximation of the ribs, which contributes to the limitation of total thoracic flexion ROM. Similarly, extension of the thoracic spine causes the ribs to separate and consequently tends to expand the chest. Side bending of the thorax produces approximation of the ribs on the side of the concavity and separation on the side of the convexity, contributing to the limitation in side-bending excursion. Right rotation of the thoracic spine in the transverse plane rotates the right transverse process posteriorly and the left transverse process anteriorly. This rotation tends to pull the right rib posteriorly and to push the left rib anteriorly. Movements of The Costal Cartilages and Sternum Elevation of the ribs causes a slight anterior and superior movement of the sternum and torsion of the costal cartilages. Joints and articulation The ribs articulate with the vertebrae in mainly two areas: (1) the bodies of the vertebrae (2) the transverse processes. It’s called as costovertebral joints The articulating surface on the vertebral body : facet and is located laterally and posteriorly on the body near the beginning of the neural arch. Some ribs articulate partially with two bodies. 1. superior part of the vertebral body below 2. inferior part of the vertebral body above. These facets are often called demifacets because they articulate with only about half of the rib. A facet that articulates with the tubercle and neck of the rib is located on the anterior tip of the transverse vertebra LUMBAR SPINE LUMBAR JOINT Consist of a large , cylindrical vertebral body anteriorly , with a bony ring or neural arch posteriorly Vetebral bodies along with IVD = provide the vertical dimension (length) of the lumbar spine and sustain most of the compressive load Neural arch = sustains most of the torsional load bearing acting on the lumbar spine most often injured absorbs the majority of our body weight and any weight we carry. COG located anterior to the second sacral vertebra. Most movement occurs between L4/L5 and L5/S1 = IVD herniation Pedicles = roughly cylindrical Sustain high compressive and tensile loads that occur during spinal rotation , flex and extension Laminae : flat. Vertebral foramen : triangular shape Upper lumbar spine : oval shape ORIENTATION OF THE FACET JOINTS Direction : parallel to sagittal plane Movt : flexion and extension Load bearing of the lumbar spine Resist anterior shear force Resist torsion Resisting compressive forces The lumbar spine functions as a complex interplay of musculoskeletal and neurovascular structures creating a mobile, yet stable, transition between the thorax and pelvis. In addition to mobility and stability, the lumbar spine provides the fibro-osseous pathway for the inferior portion of the spinal cord, the cauda equina, and the lumbosacral spinal nerves traveling to and from the trunk and lower extremities. LIGAMENT 1. Ant. Longitudinal 2. Post. Longitudinal 3. Supraspinous 4. Ligamentum Flavum 5. Interspinous 6. Intertransverse 7. iliolumbar Motion of lumbar spine FLEXION - Limited by tension in the posterior annulus fibrosis and posterior ligament EXTENSION - Less than flexion - Excessive lumbar extension = increased loads on posterior elements on the lumbar spine ROTATION - Excessive rotation = impact to IVD - Due to sagittal alignment of the facet joint , the transverse plane motion of the rotation is quite restricted - Joint coupling Rotation in Lumbar spine To withstand compressive loads, the disc is much less able to tolerate torsional (rotational) forces. During torsional stress on the IVD, the annulus fibrosus is loaded in tension. Recall, however, that the annulus fibrosus is a series of obliquely arranged fibers; thus, during rotation of a vertebral body, a portion of these fibers is not under tension. Therefore, only a portion of the annulus fibrosus is able to resist a torsional stress. Fortunately, for the lumbar spine, the sagittal plane arrangement of the facet joints in this region limits rotation and thus protects against these forces. Rotation in Lumbar spine This protective mechanism is significantly reduced when the spine is in flexion; thus, a common mechanism of disc injury is combined rotational and forward-bending movements. Stress on the fibers of the annulus fibrosus during lumbar rotation. The crisscross arrangement of the collagen fibers results in only a portion of the fibers being loaded. Gross Motion of the Lumbar Spine Sagittal plane alignment of the lumbar facet joints favours flexion and extension but greatly limits rotation. The height of the IVD acts to maintain the alignment of the joint surfaces as well as maintaining tension on the segmental ligaments. The vertical dimension of the IVD space also is related to the available motion at a given motion segment, since the deformation of the disc contributes to the motion between adjacent vertebrae. In the lumbar spine, the normal disc spaces are larger than are those of the thoracic spine. This contributes to the relatively large arc of motion that is possible in the lumbar spine. With disc space narrowing, such as occurs with disc degeneration, changes in the positional relationships of the vertebrae to one another can adversely affect joint mechanics LUMBAR FLEXION Lumbar flexion (forward bending) is achieved by a “flattening” or perhaps a slight reversal of the normal lumbar lordosis. When a person bends forward from the standing position, segments are recruited from rostral to caudal (i.e., the upper lumbar segments move into flexion first followed by the middle then lower segments). Lumbar flexion is limited by tension in the posterior annulus fibrosus and the posterior ligament system. Angular Displacement at the Segmental Levels of the Lumbar Spine Lumbar extension Lumbar extension, or backward bending, occurs in a similar but opposite manner to lumbar flexion (i.e., it is an increase in the lumbar lordosis). The overall magnitude of lumbar extension is much less than is that of lumbar flexion because of the unique bony geometry of the lumbar vertebrae. As the lumbar spine extends from a normal lordosis, the spinous processes approach one another, and tension in the anterior longitudinal ligament restricts motion. LUMBAR ROTATION Lumbar rotation, or twisting, as previously described, is potentially deleterious to the IVDs if it is excessive. Because of the roughly sagittal plane alignment of the facet joints, the transverse plane motion of rotation is quite restricted in the lumbar spine, limited by the approximation of the facet joint surfaces. Anatomically, the facet joint surfaces at L5–S1 tend to have a more oblique arrangement than do the other segments of the lumbar spine. Segmental Motion of the Lumbar Spine Segmental motion of the spine occurs at the three-joint complex of the motion segment or vertebral unit, which is composed of two adjacent vertebrae and the tissues included within them. The plane of its facet joints and the height of the IVD influence the movements of a motion segment. EMG studies demonstrate that the erector spinae cease electrical activity about halfway through a full forward bend and remain silent until the individual returns about halfway toward the upright position. The transversospinales muscles produce contralateral rotation by pulling the spinous process of the superior vertebrae toward the transverse process of the inferior vertebrae The extensor muscles and posterior ligaments apply an internal extension moment (MINT) to balance the external flexion moment (MEXT) “Hoop stress” created within the IVD during compressive load bearing. Compressive loading on the nucleus pulposus causes it to exert radial stresses on the annulus fibrosus. The concept of the nucleus pulposus acting as a ball bearing during lumbar motion. This principle results in deformation of the nucleus in the direction opposite the motion. During lumbar flexion, the nucleus pulposus tends to deform posteriorly. In lumbar extension, the nucleus pulposus tends to deform anteriorly. MECHANISM OF INJURY IN LUMBAR SPINE Lumbar spine is subjected to large compressive and shear forces. However, the margin of safety is much larger in compressive loading than in shear loading since the spine can tolerate well over 10 kN in compression, but 1,000 N of shear force causes injury with cyclic loading and onetime loading of 2,000 N [448 lb] is approaching ultimate strength. Lumbar spine flexion shows that the anterior shear forces can reach dangerous levels from the upper body reaction (Rs), interspinous ligaments (1 and 2), and reorientation of the lumbar extensor fibers of longissimus and iliocostalis muscles (3), which decrease their ability to support shear as they change their orientation during lumbar flexion. (Rc, compression reaction force; mg, upper body weight.) Loads during a fall Landing on the buttocks pushes the pelvis anteriorly and creates a posterior shear on the lumbar spine. PELVIC GIRDLE Four bones make up the pelvic girdle: i) Sacrum ii) coccyx, iii) two hip bones (ilium, ischium & pubis) joints or articulations: i) right and left sacroiliac joints posterolaterally, ii) symphysis pubis anteriorly iii) lumbosacral joint superiorly FUNCTIONS i) supports the weight of the body through the vertebral column and passes that force on to the hip bones. ii) receives the ground forces generated when the foot contacts the ground and transmits them upward toward the vertebral column. iii) During walking, the pelvic girdle moves as a unit in all three planes to allow for relatively smooth motion. iv) it supports and protects the pelvic viscera, provides attachment for muscles, makes up the bony portion of the birth canal in females. MALE and FEMALE -more oval in females - heart-shaped in males. -The pelvic cavity is shorter and less funnel-shaped in females. The sacrum is shorter, and less curved. - The acetabula and ischial tuberosities are farther apart. - pelvic arch is wider and more rounded in females Sacroiliac joint is a synovial, nonaxial joint between the sacrum and the ilium. It is described as a plane joint; however its articular surfaces are very irregular = helps to lock the two surfaces together. functions: i) transmit weight from the upper body through the vertebral column to the hip bones. ii) It is designed for great stability and has very little mobility. SI joint motion Nutation : sacral flexion, -occurs when the base of the sacrum (on the superior end) moves anteriorly and inferiorly -This causes the inferior portion of the sacrum and the coccyx to move posteriorly - pelvic outlet becomes larger Counternutation = sacral extension, -The base of the sacrum moves posteriorly and superiorly causing the tip of the coccyx to move anteriorly. - The pelvic inlet becomes larger. LIGAMENT OF SACRAL Three accessory ligaments : i) sacrotuberous ligament is a very strong & triangular- prevents forward rotation of the sacrum. ii) The sacrospinous ligament is also triangular- shaped and lies deep to the sacrotuberous ligament = to attach to the spine of the ischium. iii) iliolumbar ligament connects the transverse process of L5. Pelvic girdle motion The pelvic motions occur in all three planes. i) standing in the upright position, the pelvis should be level; in the sagittal plane, the anterior superior iliac spine (ASIS) and pubic symphysis should be in the same vertical plane. Anterior tilt : pelvis tilts forward, moving the ASIS anterior to the pubic symphysis. Posterior tilt when the pelvis tilts backward, moving the ASIS posterior to the pubic symphysis Lateral tilt = when the two iliac crests are not level. Because the pelvis moves as a unit For example : right unilateral stance, the joint axis would be the right hip. The side of the pelvis farthest away would be theleft side. During walking, the pelvis is level when both legs are in contact with the ground. However, when one leg leaves the ground (swing phase), it becomes unsupported and the pelvis on that side drops slightly Pelvic rotation : transverse plane around a vertical axis when one side of the pelvis moves forward or backward in relation to the other side. both ASISs should be in the same plane. With forward rotation of the pelvis, left leg is weight bearing and the right leg is swinging forward. the unsupported side is the point of reference. This causes the right side of the pelvis to rotate forward moving the right ASIS forward of the left ASIS. If the right leg were to swing backward pelvic rotation : occurring because the pelvis is moving on the weight-bearing hip joint.