Problem Solving PDF
Document Details
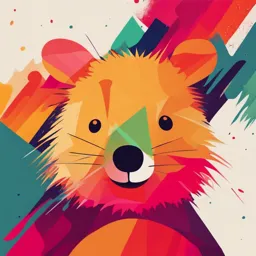
Uploaded by AdmirableFlute
Tags
Summary
"Problem solving" document provides examples of calculations related to spine mechanics. It examines factors like compressive force, shear force, and tensile force within the vertebral column and intervertebral discs.
Full Transcript
Problem solving: Problem (1): Calculate durability of the spine if there is flat lumber curve in comparison to totally straight curve Answer: In case of flat lumber curve there is only 3 curves: (3)square+1= 9+1= 10 times more durable than straight curve Problem (2): If the load falling on L3 i...
Problem solving: Problem (1): Calculate durability of the spine if there is flat lumber curve in comparison to totally straight curve Answer: In case of flat lumber curve there is only 3 curves: (3)square+1= 9+1= 10 times more durable than straight curve Problem (2): If the load falling on L3 is 50 Kg, then calculate how much compressive force on the parts of the vertebra and calculate how much shearing forces the facets will be able to carry? Answer: Load on L3= 50*10= 500N The vertebra consists of two parts anterior vertebral body and posterior body ring the vertebral body carry 80% of total compressive force) and the bony rings carry 20% of total compressive force) so: Compression force on anterior vertebral body = 80*500/100= 400N Compression force on the bony rings= 20*500/100= 100N The facets carry 50% of total shear force so: Shear force on the facets = 50*500/100=250N of shear force Problem (3): During loading by a 8kg external weight, calculate the compression forces on parts of the intervertebral disc? And calculate the tensile force on the posterior part of annulus fibrosus? Answer: The intervertebral disc consists of two parts annulus fibrosus and the nucleus pulposus The load on the intervertebral disc = 8 *10= 80N Compression force on the nucleus pulposus = 1.5 * externally applied load/area= 1.5 *80 = 120N Compression force on the annulus fibrosus = 0.5* externally applied load/area= 0.5*80= 40N. Tensile load on the posterior part of annulus fibrosus = 4 times applied axial compression load on annulus fibrosus = 4*40 = 160N Mechanical stability of lumber spine Mechanical stability of lumber spine Stability of the spine can be expressed in terms of stiffness. The stiffness of spine is the ability of the spine to resist an applied load. Stability is often regarded to be a static principle. Instead, stability and control should be thought of as a dynamic process of controlling static positions when appropriate in the functional context, but allowing the trunk to move with control in other situations. Stability would be optimal if stiffness was maximized and no lumbo-pelvic movement was allowed. However, in reality, the muscle system modulates or changes stiffness to match the demands of internal and external forces. The spine is subjected to the following stresses not only during normal functional activities but also at rest: shear stresses torsion bending axial compression The ability of spine to resist these loads varies among spinal regions and depends on: a- Type, duration and rate of the load b- Structural elements of the spine: Vertebral joints, discs, muscles, joint capsules and ligaments. The spinal stabilization system: The spinal stabilization system consists of integration between the following subsystems: 1- The passive subsystem. 2- The active subsystem. 3- The neural control subsystem. 1- The passive subsystem: It incorporates the osseous, articular structures and the spinal ligaments, all of which contribute to the control of spinal movement and stability. While being integral components of the spinal stabilization system, the passive elements offer most restraint towards the end of the range of movement, but they do not provide substantial support around the neutral position, where the spine exhibits least stiffness. 2- The active subsystem: It refers to the force generating capacity of the muscles themselves, which provides the mechanical ability to stabilize the spinal segment. The trunk muscles was categorized based on architectural properties into: (a) local muscle systems and (b) global muscle systems (a) The local muscle system included deep muscles and the deep portions of some muscles that have their origin or insertion on the lumbar vertebrae. These muscles control the stiffness and intevertebral relationship of the spinal segments and the posture of lumbar segments. Although this system of muscles is essential for stability, it is not sufficient for stability as the muscles are ineffective for control of spinal orientation. The role of the local muscle system in the support of the spinal segments, and its role in complex functions where the control of the spine must be matched to the demands of internal and external forces. The lumbar multifidus muscle, with its vertebra to vertebra attachment, is a prime example of a muscle of the local system. The smaller intersegmental muscles, such as the intertransversarii and interspinales, may not predominate as mechanical stabilizers but have a proprioceptive role instead. It was suggested that the posterior fibers of the obliques internus abdominis, which inserts to the thoracolumber fascia, form part of the local system. The deepest muscle, the transversus abdominis, with its direct attachments to the lumbar vertebrae through the thoracolumbar fascia and the decussations with its opposite in the midline, can also be considered a local muscle of the abdominal muscle group. (b) The global muscle system encompasses the large, superficial muscles of the trunk that do not have direct attachment to the vertebrae and cross multiple segments. These muscles are the torque generators for spinal motion and act like guy ropes to control spinal orientation, balance the external loads applied to the trunk and transfer load from the thorax to the pelvis. Muscles that may be considered as part of the global system are: 1- The obliqus internus abdominis 2-the obliqus externus abdominis, 3-the rectus abdominis, 4-the lateral fibers of the quadratus lumborum 5-portions of the erector spinae. 3- The neural control subsystem The muscle system is only as good as the system that drives it, the control subsystem. This system must sense the requirements of stability and plan strategies to meet those demands. The neural control subsystem must coordinate muscle activity in advance of predictable challenges to stability - and coordinate responses to afferent feedback from unpredictable challenges. The system must activate muscles at the right time, by the right amount, in the correct sequence and then turn muscles off appropriately. Factors affecting stability of the spine: I-Intra abdominal pressure II-Trunk muscles co contraction during motion III- Facet joints IV- spinal ligaments V- Pelvic motion I- Intra-abdominal pressure (IAP): Intra-abdominal pressure is created within abdominal cavity as a result of the coordinated action of: A. Abdominal muscles in the anterior border of abdominal cavity especially transverse abdominus (TrA) B. Diaphragm in the upper border of abdominal cavity C. Pelvic floor muscles in the lower border of abdominal cavity D. Multifidus muscle in the posterior border of abdominal cavity Intra-abdominal pressure (IAP) provides stability to the spine (anteriorly) and it contributes to trunk control. The abdominal cavity functions as a pressurized 'balloon' in front of the spine, with a force up on the diaphragm and down on the pelvic floor to extend the trunk * Transverse abdominus (TrA): When the TrA contracts bilaterally, it reduces the circumference of the abdominal wall and flattens the abdominal wall in the lower region to increase the lAP and tension in the thoracolumbar and anterior fascias. TrA is considered to have its major effects on lumbopelvic stability via: 1. increases in IAP 2. increases fascial tension 3. Compression of the sacroiliac joints and, potentially, the pubic symphysis. Fascial tension: Tension in the thoracolumbar fascia has long been considered to contribute to spinal stability. Up to 40% of trunk stability in the coronal plane may be produced by tension of the middle layer of the thoracolumbar fascia. TrA could produce an extensor torque (extensor moment) owing to the oblique orientation of the fibers of the thoracolumbar fascia by converting lateral tension to longitudinal tension. Lateral tension on thoracolumbar fascia can be exerted by TrA and to lesser extend by internal obliqus muscle N.B: Two issues are important to consider: First, unless activity of the diaphragm and pelvic floor muscles accompanies that of TrA, contraction of TrA will simply displace the abdominal contents with minimal effect on the lAP and fascial tension Second, TrA also contributes to control of abdominal contents and respiration, and these functions must be coordinated. ** The diaphragm: The major action of the diaphragm is inspiration The largest contribution from the diaphragm is likely to be through its role as a major contributor to IAP. Furthermore, its activity is required to prevent displacement of the abdominal viscera so that activity of TrA increases tension in the thorcolumbar fascia. Functions of intra-abdominal pressure: 1. It decreases the compressive force on the lumbar spine (it acts like a balloon). 1- It decreases the extension moment of back muscles to 10-40% of extension load. As it produce extensor moment reflected in decrease extensor muscle activity. 2- It contributes to mechanical stability of the spine through co-activation between antagonistic trunk flexors and extensors. 3- It increases during static and dynamic conditions in many everyday tasks as in lifting, lowering, walking and running 4- Intra-abdominal pressure (IAP) provides stability to the spine (anteriorly) and it contributes to trunk control. II- Trunk muscles co-contraction during motion: A- During flexion: The first 50-60 degrees flexion The movement occurs at lumber spine then anterior tilting of pelvis will share in a lumber pelvic rhythm. At first abdominal muscles work with psoas major muscle of the hip then flexion of trunk will be controlled by erector spinae of the back and posterior hip extensor muscles control forward pelvic tilting. At 90 degree of trunk flexion: Quadratus lumborum stars to contribute controlling the movement At full flexion 130 degree: Quadratus lumborum and deep erector spinae are active while superficial erector spinae are inactive and that is called flexion relaxation phenomenon. The counterbalance will be from the posterior back ligament During extension: The initial phase of extension is controlled by back extensor but in hyperextension the back muscles show less activity of extensors while the abdominal muscles become active *N.B: Flexion relaxation phenomena: III- Facet joint: The load bearing function of the facet joints depends on their orientation and position of the spine Orientation of facets of the intervertebral joints determines the motion of the spine. The facets start from 45 degree to the transverse plane (parallel to the frontal plane) at the cervical region then their inclinations are graduated plane till reach 90 degree with the transverse plane (45 degree to frontal) at the lumbar region. Apophyseal joints are primarily responsible for guiding intervertebral motion, much as railroad tracks guide the direction of a train. The geometry, height, and spatial orientation of the articular facets within the apophyseal joints greatly influence the prevailing direction of intervertebral motion. e.g: The L5–S1 apophyseal joints provide an important source of anterior-posterior stability to the lumbosacral junction. Acting as mechanical barricades, the articular processes permit certain movements but block others. In general, the near–vertically oriented apophyseal joints within the lower thoracic, lumbar, and lumbosacral regions block excessive anterior translation of one vertebra on another. Functionally this is important because excessive anterior translation. significantly compromises the volume of the vertebral canal—the space occupied by the spinal cord or passing spinal nerve roots. Horizontal facet surfaces favor axial rotation, whereas vertical facet surfaces (in either sagittal or frontal planes) block axial rotation. In forward bending coupled with rotation, the load is high on facets. But load on facets is greatest on hyperextension of spine reaches 30% of the total load. Facets can resist 50% of total shear forces due to its orientation. IV- spinal ligaments: The vertebral column is supported by an extensive set of ligaments. Spinal ligaments limit motion, help maintain natural spinal curvatures, and, by stabilizing the spine, protect the delicate spinal cord and spinal nerve roots. All ligaments provide intrinsic support to the spine All ligaments have high collagen content but ligamentum flavum has high elastin content so it has more elasticity Ligamentum flavum is under tension even in neutral position. It pre-stresses the intervertebral discs as it is located at a distance from the center of motion of disc. The ligamenta flava (plural) extend throughout the vertebral column, situated immediately posterior to the spinal cord. The highly elastic nature of the ligamentum flavum is interesting from both a functional and a structural perspective. In addition to providing gradual resistance to the full range of flexion, its inherent elasticity also exerts a small but constant compression force between vertebrae, even in the anatomic position. The elasticity may prevent the ligament from buckling inward during full extension. A forceful buckling, or in-folding, might otherwise pinch and injure the adjacent spinal cord. The interspinous ligaments drawn taut only at the more extremes of flexion. The supraspinous ligaments resist separation of adjacent spinous processes, thereby resisting flexion. The intertransverse ligaments are poorly defined, thin or membranous structures that extend between adjacent transverse processes. These tissues become taut in contralateral lateral flexion and, to a lesser degree, forward flexion. The anterior longitudinal ligament becomes taut in extension and slack in flexion. In the cervical and lumbar regions, tension in the anterior longitudinal ligament helps limit the degree of natural lordosis. The posterior longitudinal ligament becomes increasingly taut with flexion The capsular ligaments of the apophyseal joints are relatively loose (lax) in the anatomic (neutral) position, but at least some fibers become increasingly taut as the joint approaches the extremes of all its movements. Passive tension is naturally greatest in motions that create the largest relative movement between joint surfaces. Summary of ligaments: When sagittal plane movement is considered, for example, any ligament located posterior to the vertebral body is stretched (and thereby pulled taut) during flexion. Conversely, any ligament located anterior to the vertebral body is stretched during extension. So all ligaments except the anterior longitudinal ligament would become taut in flexion. V- Pelvic motion: The pelvis is a ring-shaped structure made up of three bones: the sacrum and the two innominate (hip) bones. The three bones are held together in a ring shape by ligaments. The innominat bones are themselves made from the fusion of three other bones, the ilium, the ischium and the pubis. The posterior structures of the pelvis, the sacrum and the ilia carry out the actual weight bearing function. The pubis joins the bones together, completing the ring shape and acting to prevent the pelvis from collapsing under weight bearing. Sacroiliac joint function acts as a shock absorber and protects the intervertebral joints. The pelvis can be likened to an arch that transfers the load of superincumbent body parts to the femoral heads in standing and to the ischial tuberosities (part of the two ischia) in sitting. Under weight-bearing, the tendency for the sacrum to slide forwards anteriorly is resisted by the strong ligaments between the sacrum and the ilia. It is the posterior sacroiliac ligaments that stabilize the joint between the sacrum and the ilia. The lumbar spine arises from the sacrum and the degree of lumbar lordosis depends on the sacral angle, which, in turn, depends on the tilt of the pelvis. The pelvis can be represented as in the following figure, as part of a lever system, with the hip joint regarded as a fulcrum. Many muscles attached to the pelvis can be considered as guy ropes or stays that fixate the pelvis onto the heads of the femora. These muscles can exert torques on the pelvis, which cause change in pelvic tilt (even though this is not their main function). The hamstring, gluteal, iliopsoas, erectores spinae muscles and other muscles together with the ligaments of the hip joint are part of the lumbo-pelvic system. The tilt of the pelvis in the anterior/posterior plane depends on equilibrium of the torques exerted by the antagonistic muscles in the system. The simplest countryman understands that one cannot put on the top story of a house until one has built the ground floor and foundations; yet medical men are constantly trying to alter the position of the upper bricks of the spinal column without adjusting the base on which they stand. Some ergonomists could be accused of making the same mistake when trying to design seats to prevent excessive lumbar flexion. Parents’ admonitions to young children to ‘sit up straight and don’t slouch’ may be equally mistaken. When looking at the posture of the spine at work, it is necessary to consider the factors that determine the position of the pelvis, such as the slope of the seat or the position of the feet on the floor. * N.B: All the previous factors provide intrinsic stability of the spine in addition to other factors related to bony structure of the spine such as trabecular system of the vertebral bone (vertical trabeculae resist compression while horizontal and oblique trabeculae resist tensile and shearing stresses as mentioned before). External stabilizer or support of the lumbar spine (Back belt): it is not advised to use it for a long time especially for patients with weakness of the abdominal muscles which leads to more weakness of the muscles. It is better to strengthen the abdominal muscles using abdominal exercise.