Protein Structure Determination using NMR PDF
Document Details
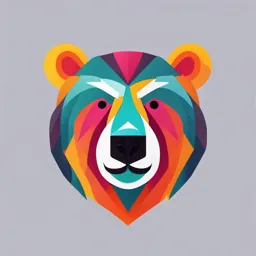
Uploaded by YouthfulGothicArt
Tags
Summary
This document describes the process of protein structure determination using Nuclear Magnetic Resonance (NMR). It details the various steps, from sample preparation to analyzing the results. The techniques and principles behind NMR are explained in a way that is accessible to those with a background in biochemistry.
Full Transcript
Protein structure determination using Nuclear Magnetic Resonance Overview of NMR structure determination Express and Collect multi- Assign each Collect an Use NOE purify 15N 13C dimensional resonance peak NOE spectrum interatomic distance...
Protein structure determination using Nuclear Magnetic Resonance Overview of NMR structure determination Express and Collect multi- Assign each Collect an Use NOE purify 15N 13C dimensional resonance peak NOE spectrum interatomic distance labeled spectra (several to a specific for structure constraints to protein (~10mg) different protein atom calculation determine the experiments) structure The overall logic of NMR structure determination The chemical shift of a given atom serves as an address that is consistent across all spectra Through-bond coupling experiments tell you which atom is bonded to which, and allows you to ultimately associate a given peak with a specific atom in the structure Through-space coupling experiments tell you which protons are close in space, irrespective of whether or not the atoms are bonded Knowing which atom pairs are close in space, one can produce a series of structures consistent with the observed data The structure is reported as an “ensemble” of several related solutions all consistent with the data Note – NMR is very different than scattering based techniques NMR active nuclei While 1H is naturally abundant, determining the structure of a reasonable sized protein also requires that we use NMR active 13C and 15N atoms Since these are rare in natural sources, natural proteins are not suitable for NMR Recombinant proteins are instead grown in minimal media with carbon (13C glucose) and nitrogen (15N ammonium) sources labelled Need relatively large amounts of Stryer 5 Table 4.4 protein (milligrams) Sample preparation You need millilitres of millimolar sample (lots of protein) It may take months to do all your measurements, so sample has to be stable – precipitation or proteolysis are potential problems Protein samples need to have the appropriate isotope labels for the experiments envisaged Buffers and salts need to be NMR invisible (no CH or NH groups) Generally, once you make the appropriately labelled sample, you can do all your structure determination experiments with it The instrument - an NMR spectrometer A tube containing the protein sample is inserted into the magnet The NMR magnet is made from superconducting coils cooled with liquid helium The probe measures radio waves - the signal used to reconstruct the structure http://bchs.uh.edu/~kecknmr/images/bruker800.JPG The physics of NMR - nuclear spin precession axis Atomic nuclei have a quantum w property known as “spin” Individual nuclei may be thought of as bar magnets that precess around an axis at a rate (w) of millions of times per second (slow!) The result, averaged over time, is a net magnetization along the precession axis Nuclear Spin K. Bishop, U Maine The spins tend to align with an external magnetic field Bo In the absence of any If an external magnetic field (Bo) external magnetic field, this is applied, the spins will tend to net magnetization points in a align in slight excess with the random direction field K. Bishop, U Maine In an external magentic field, being aligned with the field is a lower energy state than being aligned against it The interaction with the external magnetic field is weak, so only a very small excess of the nuclei align with versus against the field Increasing the magnetic field strength increases the energy difference, giving a stronger signal Stryer5 Fig. 4.43 Perturbing the spin distribution In the presence of an external magnetic field, a small excess of nuclei tend to align with the field Adding energy to the system can result in fewer nuclei being aligned with the field than at equilibrium This is an excited (high energy) state of the system Synchronized precession By default, nuclei precess at random relative to one another It is possible to synchronize the nuclei, so they precess in lockstep This too is an excited state of the system Radio wave free induction decay Free Induction Decay (FID) Radio frequency pulse The sample is excited (i.e. shifted into a non-equilibrium state) using a specifically designed pulse of radiowaves After the pulse has ended, you measure the faint radio frequency signal emitted by the sample as it relaxes back into the ground state This signal drops off exponentially over time, and is known as the Free Induction Decay (FID) Converting the FID to frequencies Free Induction Decay Fourier Transform time frequency in ppm K. Bishop, U Maine The data is measured as an amplitude of signal as a function of time The Fourier transform (FT) is a mathematical operation that converts time dependent signals into a set of frequencies Chemical Shift The electrons in the neighbourhood of an atom partially shield the nucleus from the external magnetic field The electrons near an atom depend primarily on the atom type and its chemical environment As a consequence, different atoms of a given element will precess at slightly different frequencies The chemical shift of an atom is largely dictated by its chemical environment (e.g CH2 vs CH3 vs OH protons above) Chemical shifts are given in parts per million relative to a standard Characteristic chemical shifts of protons The chemical shift depends on the proton’s chemistry Chemical shift in protein spectra In protein spectra, chemical shift also depends (weakly) on the structural environment of a nucleus as the electrons of nearby residues shield a nucleus, with varying effectiveness The chemical shift of a particular atom is a characteristic of that atom, and can be used as the “address” of that atom This characteristic shift is what allows an atom to be identified in all the different spectra the spectroscopist collects If the protein structure changes, the chemical shift may change slightly - you can exploit this e.g. to monitor ligand binding Problem: proteins have large numbers of protons, so the address is often not unique Spins of adjacent nuclei influence one another 1H Different nuclei can influence each other’s spins (and therefore NMR signals) by two effects – Scalar coupling - atoms connected through 1H 1H covalent bonds influence each other through shared electrons – Nuclear Overhauser Effect - atoms close in space (whether they are bonded or not) influence each other through their magnetic fields Scalar coupling - through bonds l Scalar coupling goes through chemical bonds l Scalar coupling results in the splitting of peaks l If two atoms are connected by at most three chemical bonds, scalar coupling will occur l The strength of scalar coupling depends on the dihedral angle l Scalar coupling therefore gives some information about dihedral angles such as Phi and Psi Multidimensional NMR different delays collect spectrum Multidimensional spectra are collected as a series of 1D spectra These spectra are initiated by (at least) as many excitatory pulses as dimensions we are collecting The delay time between pulses is varied systematically Each collected spectrum will then differ from the others in a time delay dependent fashion Changes in a given peak in the spectrum over time reflect the influence of nearby nuclei 2D NMR experiments l For 2D NMR we use two pulses with variable delay, and collect multiple spectra with increasing delays Integrating 2D NMR Experiments Interferogram FID at fixed f2 t1 t1 t1 f2 FT of t1 2D plot of data f1 f1 f2 f2 Contour plot. The end result is a 2D contour plot where peaks represent interactions between two atoms Bax and Morris, 1981 Multidimensional NMR experiments An N-dimensional NMR experiment effectively uses physics to ask the question “are these N nuclei coupled” In a 2D scalar coupling experiment, if the two atoms are connected by a bond, then we will see a peak in the spectrum at the point where their respective chemical shifts intersect 1H at 4.01 ppm is attached to a 13C at By changing the frequency characteristics 55.45 ppm of the pulse applied, one can select which elements will resonate Multidimensional NMR 1D spectrum 2D spectrum 3D spectrum Peaks in a 1D spectrum tell us about the properties of individual nuclei Peaks in multidimensional spectra arise from interactions between linked atoms (through space or through bonds) Often different dimensions represent different elements (H/C/N) 3D NMR Experiments Ca Ha N l Extra dimensions can be added by adding more pulses and evolution times l The increased dimensionality reduces peak overlap l However it also greatly increases time to required to collect data Zhou, 2006 Assignment Spectra that probe through bond-coupling can be used to map individual peaks onto the chemical structure of the protein Starting out with one peak, you can map out the connections between nuclei, and see which part of the structure the connections are consistent with Using two specially designed multi-dimensional spectra, you can map out the connections between the backbone protons of all pairs of successive residues Helpful is the fact that typical chemical shift of CHa and CHb shifts differ between amino acids, G has an extra CHa, etc. Side chains are mapped out in different experiments Ultimately, you can map out the full chemical structure of the protein, and infer the chemical shift of each proton Solving the NMR structure The goal of assignment (which makes up the bulk of the specta collected) is to figure out the characteristic chemical shift of each NMR active atom in the protein Once done, you can in future always “recognize” each atom in experiments which tell you about the structure NOE experiments contribute most of the structural information, but other pieces of data also help Constraints Constraints are observations that helps distinguish the correct structure from incorrect structures NOE provide distance constraints of less than 5 Å (The most important constraints in protein NMR) Coupling constants restrict possible dihedral angles Other constraints… Chemical shifts – give some info about an atom’s environment E.g. you can tell if a hydrogen atom is participating in a hydrogen bond NOE - interactions through space NOEs (nuclear Overhauser Effects) arise because of dipolar coupling (interaction between local fields) Each nucleus acts as a small bar magnet Each nucleus is sensitive to the direction the other nuclei in its N N neighbourhood are pointing, S S Nearby magnetic fields reinforce or undermine the external magnetic field NOEs are NMR measurements that exploit this effect to measure interactions of atoms through space NOEs K. Bishop, U Maine NMR Interactions - through space Since the NOE effect depends on distance, r 25 kDa) very challenging Intensive user involvement - lots of thoughtful interpretation needed, with minimal reliable automation (slow) Strengths of Protein NMR Can monitor protein dynamics - a blind spot for other techniques Flexible proteins or regions are not a major problem No need for crystals Once you have completed an assignment, individual NMR peaks can be used as probes of their local environment Can monitor changes in the structure as the protein's environment changes - e.g. as the protein folds Can monitor binding, even for very low affinity interactions Can monitor individual residue titrations and determine pKa’s Conclusion: NMR is a powerful biophysical tool, but not the easiest way to determine routine structures