Protein Energetics - T01 PDF
Document Details
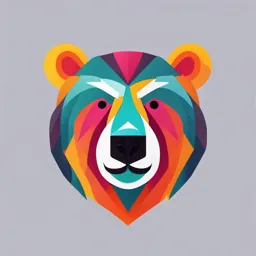
Uploaded by YouthfulGothicArt
Tags
Summary
This document covers protein energetics. It discusses the factors affecting the pKa values of protein residues, the role of dielectric constants in protein interactions, and the entropic costs associated with protein folding.
Full Transcript
### The pKa of residues in structured proteins vary from their solution values - If you titrate a pure amino acid in water, the ionization energy reflects only interactions with water - Within a structures, local interactions will often favour a specific amino acid being either neutral...
### The pKa of residues in structured proteins vary from their solution values - If you titrate a pure amino acid in water, the ionization energy reflects only interactions with water - Within a structures, local interactions will often favour a specific amino acid being either neutral or charged - This alters the energetics of ionization, resulting in a shift in the pKa value - E.g. in xylanase, adjacent glutamate residues repel electrostatically if both are charged - This favours Glu172 being protonated, shifting the pKa by \~2 pH units Mobile dipoles screen charge-charge interactions ------------------------------------------------ - Recall -- the dielectric constant attenuates charge interactions - The dielectric constant is an empirical value that reflects the observation that electrostatic interactions in media are weaker than ideal values in a vacuum - Microscopically, local solvent dipoles tend to try to reorient so as to counteract the local electrostatic field - Water has a strong dipole moment and is very mobile, so the dielectric constant of water is very high \~80 - Organic solvents have much weaker dipole moments (from the C-H bond) and therefore very low dielectric constants e.g. cyclohexane D = 2 - Protein interiors have intermediate values that depend on location The dielectric constant in a protein ------------------------------------ - Proteins have strong dipoles that are constrained by the need to maintain strong H-bonds - The core of the protein is mostly occupied by non-polar side chains and somewhat resembles a non-polar solvent, with dielectric constant somewhere around 10 - Closer to the surface, the sidechains tend to be polar, the backbone is more flexible, and lots of structural water molecules mean that the dielectric constant is similar to water Charged residue interactions in proteins -------------------------------------------------------------- - Charged residues are very common on the proteins surface, where they can interact with the solvent and with dissolved ions - Mobile dissolved ions compete for protein charge-charge interactions, weakening them in most environments - Charged residues help solubilize the protein - Oppositely charged residues are often not paired, and in most proteins, charge-charge interactions add little net stability - Protein interiors are low dielectric constant - Charged residues are [rarely buried] - Burying charged residues requires energetically costly stripping off of water - Buried charge residues require stabilization by multiple H-bonds and tend to have a specific structural or functional role Salt bridges ================================== - A salt bridge is an interaction between a negatively charged residue with a positively charged group - Salt bridges require both favourable electrostatic and hydrogen bonds - These interactions are strong and help stabilize proteins - The charge-charge interaction adds - In a less dielectric medium, it will be a proportionately stronger - Because salt bridges are temperature independent, they are important for stabilizing thermophilic proteins ### Cation-Pi interactions and Pi-pi stacking - Pi electrons are delocalized and create localized partial charges - This allows pi-bonded groups to stack favourably in certain configurations - The larger partial negative charge in aromatic side chains can interact favourably with positive charges - Arg and Lys can interact strongly with aromatic residues (Trp, Tyr and Phe) - Interaction strength \~12 kJ/mol - Cation-pi interactions are common in proteins - Even Arg-Arg stacking is favourable, despite the charge-charge repulsion Entropic contributions to protein energetics -------------------------------------------- - The entropy of a system is a measure of the number of states available to a system - Everything else being equal, conformations that include a larger number of states are statistically more likely to be occupied than conformations associated with fewer states ("ordered") - Changes to more ordered states only occur when coupled to other favourable changes in the overall energetics - Entropic contributions to protein energetics arise from two major component: - Changes in the intrinsic entropy of the protein (conformational entropy) - Changes in the entropy of water (the hydrophobic effect) ### Folding a protein entails loss of conformational entropy - Protein chains have many single bonds and are quite flexible - In solution, they sample enormous numbers of discrete conformations - A folded structure has many, many fewer conformations - Reducing the number of states the protein samples exacts a large entropic cost - Proteins prefer to remain as flexible - Folding a protein comes at a large entropic cost and only occurs if specific interactions sufficiently stabilize the final structure Conformational entropy and amino acid architecture -------------------------------------------------- - Single bonds outside of rings can rotate freely - With the exception of Met, all hydrophobic amino acids have at most two freely rotatable single bonds in their side chain - This minimizes the entropic cost of burying these side chains - Many hydrophilic amino acids are more flexible (e.g. Glu, Gln, Lys, Arg) and are generally surface exposed Liquid water structure ====================== - The behaviour of liquid water is driven by the strong, directional hydrogen bonds it forms - Water molecules form extended, open networks of hydrogen bonds, with essentially all molecules forming four H-bonds at any given time - The connectivity of this network changes continuously, as waters continuously move, reorient and form new interactions with other nearby molecules ###  t Solutes can hinder these ^...^ interactions - Non-polar solutes cannot hydrogen bond with water molecules - Because H-bonds are very strong, the water molecules rearrange themselves so as to maintain a maximal number of H-bonds, despite the presence of the non-polar solute - This is done by creating cage-like structures around the solute - Maintaining such cages severely restricts the conformational possibilities for the water molecules at or near the interface - This loss of conformational freedom results in an [entropic] penalty for dissolving non-polar molecules - This is known as the hydrophobic effect ### The hydrophobic effect can drive non- polar solutes to interact - When non-polar solutes are dissolved in water, each maintains a separate water cage - If the molecules associate, there is less total exposed surface, meaning that some of the cage water is released back to bulk solvent (circled) - This results in a [net entropic gain] when non-polar molecules to associate - This is a very important driver in maintaining protein and DNA structure Measuring hydropathy ==================== - Hydrophobicity is not a force, but rather an empirical [effect] - It is a [property of the system], not of any individual component - You therefore cannot measure hydrophobicity directly - The term **hydropathy** is used to describe the relative preferences for aqueous and nonpolar environments - Hydropathy is used as an approximation of hydrophobicity - Hydropathy can be measured as a partition coefficient between water and a non-polar solvent - Note -- this is an empirical measurement, and the exact values differ depending on which non-polar solvent you use Measuring partition coefficients ======================================================= - K~D~, the partition coefficient of a molecule between water and a nonpolar solvent is calculated as: K~D~ = \[X\]~nonpolar~ ~solvent~/ \[X\]~water~ - The free energy of transfer ( GTr) is then: GTr = -RT lnKD - GTr is a commonly used as a measure of the hydrophobicity of a molecule #### Octanol-based hydrophobicity measure of amino acids Free energy of transfer ======================= - A negative G~Tr~ indicates that the molecule prefers a non- aqueous environment - At 25°C the unfavorable transfer of a nonpolar molecule from a nonpolar liquid to water is primarily a result of the unfavorable change in entropy - H 0 while S \> 0 due to increased ordering of water molecules around the nonpolar molecule - As temperature increases the ordered water shell around nonpolar molecules tends to melt out and become more like bulk water - This makes the hydrophobic effect less pronounced at higher temperatures, allowing hot water to dissolve non-polar solutes better than cold ### Hydrophobic interactions of amino acid residues - G~Tr~ is correlated with the surface area of nonpolar side chains - G~Tr~ for other residues is dominated by their polar groups - At 25˚C, the hydrophobic effect can be approximated as **0.1 kJ/mol/Å2** buried non-polar surface - E.g. Phe side chain is \~140 Å2 surface area, so it can contribute \~14 kJ/mol through the hydrophobic effect if completely buried (roughly = 1 H bond) Key interactions - summary ========================== +-----------------+-----------------+-----------------+-----------------+ | **Interaction** | **Distance** | | | +=================+=================+=================+=================+ | **Charge-charge | \~3 Å | | | | ** | | | | | | | | | | **interaction** | | | | +-----------------+-----------------+-----------------+-----------------+ | **Hydrogen | 2.4-3.4 Å | | | | bond** | | | | +-----------------+-----------------+-----------------+-----------------+ | **Cation-pi | \~4 Å | | | | interaction** | | | | +-----------------+-----------------+-----------------+-----------------+ | **van der Waals | 3.5 -- 4 Å | | | | interaction** | | | | +-----------------+-----------------+-----------------+-----------------+ | **Hydrophobic | undefined | | | | effect (per** | | | | | | | | | | **residue)** | | | | +-----------------+-----------------+-----------------+-----------------+ #### The lipid bilayer -- a non-aqueous environment - The central \~30 Å of the membrane is essentially exclusively occupied by hydrophobic lipid tails (C=C, CH~2~ and CH~3~) - Beyond this is another \~7Å which is dominated by the lipid head groups - Outside this is water - Protein embedded in the membrane has to interact with all of these environments favourably #### Membrane embedded proteins have a broad central region comprised of hydrophobic residues  cytoplasm - The central region of the protein spanning the membrane is almost exclusively hydrophobic - Aromatic residues (with a partly hydrophilic character) are concentrated at the solution/membrane interface Bound lipid molecules ============================================ - High resolution membrane protein structures typically show some bound lipid molecules - These lipids are tightly bound as they stay associated with the protein through detergent extraction - The lipids often bind in the grooves between helices - This well-ordered lipid layer is analogous to the layer of well-ordered water molecules binding the surface of soluble proteins, and is often integral to the structure - The diversity of proteins arises from polymerizing chemically distinct amino acids - 20 amino acids can be encoded by standard codons - Additional chemical diversity can be generated by covalently modifying amino acids once the protein is translated Interactions within the backbone ================================ pairs - Forming a peptide bond makes an 2o amide group - This group has a single hydrogen bond donor in the nitrogen - Two free electron pairs in the carboxyl oxygen can act as hydrogen bond acceptors - Hydrogen bonds between these peptide groups drive the formation of secondary structure Non-polar amino acids ===================== - Gly, Ala, Val, Leu, Ile, Met, Pro, Cys and Phe are all non-polar - None of these amino acids can use their side chains to make (strong) hydrogen bonds - As a result, none form favourable interactions with water and prefer to be buried in the protein interior - They mostly serve as 3D puzzle pieces that will pack together in the interior of the protein - The degree to which they prefer burial is directly proportional to their surface area - This preference for burial [is not absolute], and all can be found reasonably often on protein surfaces, e.g. in ligand binding sites Glycine -- small and flexible ============================= - Glycine is the smallest amino acid - It can readily be buried or surface exposed - Glycine is uniquely [achiral] - Because it lacks a bulky side chain, it can adopt backbone conformations no other amino acid can - Glycine is non-polar, but is not bulky enough to contribute much to the hydrophobic effect Proline - rigid =============== - Proline has an [imine] ring - This ring connects the alpha carbon to the amine N - This greatly limits the ability of proline to rotate around the single Cα-N bond - It also means that proline in a polymeric chain [cannot act as a hydrogen bond donor] - The imine ring allows Pro to more readily adopt *cis* conformations smaller hydrophobic amino acids -------------------------------  - Ala, Val, Leu and Ile are all small hydrophobic residues - All prefer to be buried, Ala less so than the others - They differ in volume and shape -- important as this gives a choice of "pieces" with which to pack protein interiors Methionine ========== - Secondary methylated sulfide separated from Cα by two methylenes - The sulfur atom has 2 free electron pairs, but since Sulfur is not electronegative, these do not form hydrogen bonds - Occasionally Met is found as a metal ligand - Methionine is non-polar - Chemically inert, and is the most flexible of the non-polar amino acids ### Aromatic amino acids (1): Phenylalanine - Phenylalanine has a phenyl ring attached by a single linking methylene group - It has no hydrogen bonding ability, is chemically inert, and strongly prefers to be buried O- ### Aromatic amino acids (2): Tyrosine - Tyrosine has a phenol ring linked by a methylene group - The hydroxyl can form three hydrogen bonds (1 donor, 2 acceptors) - Resonance stabilization within the ring allows the the hydroxyl group to be deprotonated - pKa is around 10 - Tyr is often buried as it is fairly non- polar, though the presence of the hydroxyl group allows it to be readily surface exposed - Commonly involved in enzyme reactions ### Aromatic amino acids (3): Tryptophan - Tryptophan has an [indole] ring attached - The nitrogen in the 5-membered ring is protonated and acts as a hydrogen bond donor (circle) - Otherwise, the side chain is very hydrophobic, and generally is buried within proteins - When surface exposed it can be used for stacking on ligands (e.g. sugar rings) - Trp is the bulkiest amino acid thiol Cysteine ======== - Cys has a primary thiol connected by single methylene - The sulfur atom has 2 free electron pairs plus a proton in tetrahedral geometry - But sulfur is not electronegative, so Cys [does not make] [hydrogen bonds] - Cys can fairly readily be deprotonated -- pKa is around - Cys also [readily participates in redox reactions] Cysteine: Disulfide Bonds ========================= - Two cysteine residues in close proximity in an [oxidizing] [environment] can oxidize to form a **covalent** bond - This is called a disulfide bond, (or disulfide bridge). - Disulphide bonds help lock tertiary structure into place - The cytoplasm is [reducing] - Disulfide bonds only form in extracellular proteins, or proteins within organelles e.g. ER The 21st a.a.: selenocysteine ============================= - Selenocysteine resembles cysteine, except that S is replaced by Se (selenium) - Selenocysteine is incorporated co- translationally into a UGA stop codon, but only if a transcript that has additional recognition sequences - Selenocysteine has a lower pKa (5.5) and lower reduction potential than Cys - It is generally only found in specialized redox proteins - 56 human proteins (out of \~20k) are known to incorporate this amino acid Small hydroxy-amino acids =========================  serine (Ser; S) threonine (Thr; T) - Serine has a hydroxyl group attached by a single methylene - In threonine, the methylene group is methylated - In both amino acids, the hydroxyl group has a single proton and two free electron pairs (=Tyr) - Ser and Thr cannot be ionized (pKa \> 14) Basic amino acids(1): arginine ------------------------------ - Arginine has a guanidine group linked by three methylene carbon groups - The guanidine group is resonance stabilized, so planar and rigid - The guanidine group has five hydrogen bond donating protons, all in plane - This is the most of any amino acid - The guanidine group is only deprotonated at non-physiological pH (\~12.5) - It is essentially always positively charged - The +1 charge is divided over the three nitrogen atoms Basic amino acids(2): lysine --------------------------------------------------- pKa 10.5 - Lysine has a primary amine group linked by [four] methylenes - The amine group has three hydrogen bonds donating protons, in tetrahedral geometry - Lysine is almost always positively charged - Lysine is the most flexible amino acid more basic pH ### Basic amino acids (3): histidine - Imidazole ring attached by single methylene - Imidazole is planar and can readily be protonated: pKa \~ 6.5 - If protonated, +1 charge; otherwise neutral - Both nitrogen atoms are donors if protonated, and have \~+0.5 charge each - 1 donor and 1 acceptor if deprotonated Acidic amino acids: =================== #### aspartate & glutamate - Asp and Glu differ in that Asp has one linking methylene, Glu has two - The carboxylate group is almost always negatively charged, but is occasionally stabilized in a neutral state (e.g. in some enzymes) - It has four hydrogen bond acceptors, all [in plane] with the carboxylate Amide amino acids: ================== ### asparagine and glutamine  - Asn and Gln are the amide analogs of Asp and Glu - The primary amide side chain has a nitrogen with two hydrogen bond donors, and an oxygen with two acceptors - These are all in plane with the amide group atoms