Transmission Media & Wireless Communication Study Notes PDF
Document Details
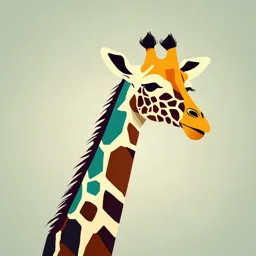
Uploaded by WellReceivedAlgebra467
Tags
Related
- COTN1220_04_Guided_And_Wireless_Media (PDF)
- CPE 303 Data and Digital Communication - Transmission Media PDF
- 1.6 Compare and Contrast Transmission Media and Transceivers PDF
- Transmission Media and Transceivers PDF
- Transmission Media & Wireless Technologies Study Notes PDF
- Networking Devices and Transmission Media - PDF
Summary
These study notes cover transmission media, including wired and wireless types. It details cellular technology, from early generations (1G, 2G, 3G) to modern 4G and 5G, and explores satellite communication, highlighting its advantages for remote areas and global coverage.
Full Transcript
**Transmission media**Â refers to the various channels through which data signals are transmitted between devices on a network. These media are the physical pathways that connect computers, switches, routers, and other network devices, enabling communication and data exchange. Transmission media can...
**Transmission media** refers to the various channels through which data signals are transmitted between devices on a network. These media are the physical pathways that connect computers, switches, routers, and other network devices, enabling communication and data exchange. Transmission media can be broadly classified into guided (wired) and unguided (wireless) media. Guided (wired) media involves physical cables that guide the data signals along a specific path. Common types of guided media include twisted pair cable, coaxial cable, and fiber optic cable. Unguided (wireless) media transmits data signals through the air or space without using physical conductors. Common types of unguided media include radio waves, microwaves, and infrared. Each type of transmission medium has unique characteristics, advantages, and limitations, which influence its suitability for different networking environments and applications. **Cellular Wireless** **Cellular wireless communication allows mobile devices to connect to the internet and make voice calls by transmitting data through a cell tower network. This technology provides mobile connectivity across large cities, rural regions, and entire countries.** **Mobile Network Operators (MNOs), also known as wireless carriers or telecom providers, manage cellular radio architectures. These operators build, maintain, and manage the infrastructure for cellular communication, including cell towers, base stations, and the core network. MNOs offer services such as voice calling, text messaging, and mobile internet access to their subscribers.** **Cellular technology has evolved through several generations, each bringing significant improvements in speed, capacity, and capabilities.** **The generations of cellular technology before 4G brought significant advancements and widespread impacts. 1G introduced basic mobile voice communication but faced limitations in security, voice quality, and accessibility. The transition to 2G brought digital technology, enhancing security and voice quality while introducing SMS, making mobile phones more accessible, and fostering economic growth. The arrival of 3G revolutionized mobile communication by enabling mobile internet access and multimedia services, leading to the proliferation of smartphones and global connectivity. These advancements transformed social interactions and daily life, stimulated economic development, and set the stage for the high-speed, data-driven capabilities of 4G and beyond.** **4G, or Fourth Generation cellular technology, introduced in the late 2000s, brought significant improvements in mobile communication with the adoption of LTE (Long Term Evolution) technology. It offers high-speed internet access, HD video streaming, and VoIP (Voice over IP) capabilities, providing speeds ranging from 100 Mbps to 1 Gbps. 4G greatly enhanced the reliability and speed of mobile networks, enabling more advanced applications and services.** **5G, or Fifth Generation cellular technology, emerged in the late 2010s, marked by the introduction of NR (New Radio) technology. 5G offers ultra-fast internet speeds up to 10 Gbps, low latency, and massive device connectivity. It is ideal for IoT (Internet of Things), autonomous vehicles, smart cities, and real-time applications like virtual reality. It supports a wide range of new use cases and promises to revolutionize mobile connectivity with its advanced capabilities.** **Satellite Wireless** **Satellite communication is a wireless transmission media that sends and receives data signals via satellites orbiting the Earth. This technology provides connectivity in remote and underserved areas where traditional wired or ground-based (terrestrial) wireless networks are not feasible. Satellite communication works through a process known as uplink and downlink: data is transmitted from a ground station (uplink) to a satellite in space, which then relays the signal back to another ground station or user terminal (downlink). The satellite acts as a repeater, amplifying and retransmitting the signal to cover great distances.** **There are two primary types of satellites: geostationary satellites and low Earth orbit (LEO) satellites. Geostationary satellites are positioned about 22,236 miles above the Earth's equator and maintain a fixed position relative to the Earth\'s surface. They are perfect for broadcast services and long-distance communication. On the other hand, LEO satellites orbit at much lower altitudes (between 311 and 1,242 miles) and offer lower latency and faster data speeds compared to geostationary satellites. LEO satellites are part of large constellations to ensure continuous global coverage.** **Satellite communication has several advantages, including global coverage, reliability, and broadcast capabilities. Satellites can connect virtually any location on Earth, including remote and rural areas, oceans, and mountains. Satellite networks are less susceptible to terrestrial disruptions such as natural disasters or infrastructure damage. Additionally, they are ideal for broadcasting services like television and radio, where the same signal needs to be distributed over a wide area.** **Satellite communication has limitations. Geostationary satellites have a much higher latency (approximately 500 - 700 milliseconds) compared to satellites. This is due to the long distance signals must travel. The monetary investment in launching and maintaining satellites is considerable, resulting in increased costs passed on to customers. Unfavorable weather conditions, such as snow, rain, or storms, can have an impact on signal quality and reliability.** **Applications of satellite communication are varied and include broadband internet services in remote and rural areas, distribution of television and radio broadcast signals, support for global positioning systems (GPS) for navigation, ensuring communication capabilities in disaster-affected areas, and offering secure communication for military operations and strategic applications.** **802.11 Standards** **The 802.11 standards, created by the Institute of Electrical and Electronics Engineers (IEEE), are the guidelines for wireless local area network (WLAN) communication. These standards define the protocols and technologies for wireless networking, ensuring that different devices work together seamlessly. The 802.11 family covers frequency bands, data rates, modulation techniques, and security protocols. By following these standards, manufacturers can produce wireless networking equipment that functions well in various settings, whether a home network, a large enterprise, or a public Wi-Fi hotspot. Each new version of the 802.11 standards brings speed, range, reliability, and security improvements to meet the growing demand for wireless connectivity.** **Wireless radio technology is essential for transmitting and receiving data over the air through electromagnetic waves. These radios are the core components of wireless communication systems, enabling device communication without physical connections. By converting digital data into radio waves, wireless radios transmit these waves through the air to be received by another radio, which then converts them back into digital data. This technology is ubiquitous in modern communication systems, including Wi-Fi, Bluetooth, cellular networks, and satellite communications, offering unparalleled flexibility and mobility.** **Wireless frequency refers to the specific rate at which electromagnetic waves oscillate during transmission. This rate, measured in Hertz (Hz), determines the wave\'s characteristics, such as its ability to penetrate obstacles, range, and data-carrying capacity. Different wireless communication technologies use different frequencies, balancing signal range, data rate, and interference. Lower frequencies generally offer longer ranges and better penetration through obstacles, while higher frequencies can transmit more data over shorter distances.** **A wireless frequency band is a defined range of frequencies used for transmitting radio waves. Regulatory bodies govern these bands to ensure efficient and interference-free spectrum use. Each band is designated for specific communication types, such as Wi-Fi, cellular networks, and satellite communications. Wi-Fi commonly operates in the 2.4 GHz and 5 GHz bands, each with different trade-offs regarding range, speed, and interference. Additionally, the 6 GHz band has been introduced in Wi-Fi 6E, offering even higher speeds and reduced congestion due to more available channels. The choice of a frequency band significantly impacts the performance and suitability of a wireless communication system for various applications.** **In wireless communication, a channel is a smaller section within a frequency band set aside for a specific communication path. Channels let multiple devices communicate simultaneously within the same frequency band without interfering with each other. Each channel has its center frequency and bandwidth. Choosing and managing channels effectively is vital to optimizing network performance, minimizing interference, and ensuring reliable communication, especially in environments with many wireless devices.** **2.4 GHz Wi-Fi Band** **The 2.4 GHz Wi-Fi band is one of the most popular frequency bands for wireless communication. It has a longer range and can penetrate obstacles like walls and furniture, making it perfect for home and small office setups. However, the 2.4 GHz band is often crowded with various devices like microwaves, Bluetooth gadgets, and cordless phones, which can cause a lot of interference. This band supports 14 channels, but because some channels overlap, it can lead to congestion and reduced performance. Despite these drawbacks, the 2.4 GHz band is still widely used because it can cover larger areas with fewer access points.** **5 GHz Wi-Fi Band** **The 5 GHz Wi-Fi band became popular over the 2.4 GHz band because it has more channels and experiences less interference from commonly found household devices. This band provides faster data rates and better performance, making it attractive for tasks such as streaming videos and online gaming. It offers up to 24 channels that don\'t overlap, helping to reduce network congestion and improve overall performance. Due to the fact it uses a higher frequency, the signals can be more easily blocked by walls and other obstacles, resulting in a shorter transmission range. In these instances, additional access points will be necessary to provide the same coverage as 2.4 GHz. The 5 GHz band excels in environments where high speed and reduced interference are most important.** **6 GHz Wi-Fi Band** **The 6 GHz Wi-Fi band, introduced with Wi-Fi 6E, is a big step forward in wireless communication. It offers faster speeds and more capacity because it has more spectrum and wider channels. With 59 non-overlapping channels, it cuts down on congestion and interference, making for a smoother and more efficient connection. The higher frequency means faster data transfer rates, which is great for things that need a lot of bandwidth and low latency, like virtual reality and large file transfers. However, similar to the 5 GHz band, the 6 GHz signals don\'t travel as far and are more easily blocked by obstacles. This band is instrumental in densely populated areas and places where devices compete for bandwidth.** **802.11a Standard** **The 802.11a standard was one of the earliest wireless standards officially ratified in 1999. Operating in the 5 GHz frequency band, 802.11a has a maximum theoretical data transfer rate of 54 Mbps. This standard utilizes orthogonal frequency-division multiplexing (OFDM) to efficiently transmit data, reducing interference and improving performance in environments with numerous wireless devices. While its higher frequency allows for faster data transmission, it also results in a shorter range than standards operating in the 2.4 GHz band. The 802.11a standard is particularly suited for enterprise networks and environments where minimizing interference is crucial.** **802.11b Standard** **The 802.11b standard, ratified in 1999, operates in the 2.4 GHz frequency band and supports a maximum theoretical data rate of up to 11 Mbps. Utilizing direct-sequence spread spectrum (DSSS) technology, 802.11b offers reliable performance but is more susceptible to interference from other devices operating in the same frequency range, such as microwaves and Bluetooth. Despite its slower speeds and potential for interference, 802.11b was widely adopted due to its cost-effectiveness and sufficient performance for early wireless networking needs.** **802.11g Standard** **The 802.11g standard, ratified in 2003, was designed to overcome the shortcomings of its predecessors. It operates in the 2.4 GHz frequency band and achieves theoretical data rates up to 54 Mbps. By employing orthogonal frequency-division multiplexing (OFDM) or direct-sequence spread spectrum (DSSS), 802.11g combines the best features of 802.11a and 802.11b. This standard is backward compatible with 802.11b devices, ensuring seamless integration and improved speeds in existing networks. The 802.11g standard became a popular choice for both home and enterprise wireless networks due to its balance of speed, range, and compatibility.** **802.11n Standard** **The 802.11n standard represents a significant leap in wireless networking technology, supporting the 2.4 GHz and 5 GHz frequency bands and offering theoretical data rates of 600 Mbps. Key to its performance are technologies such as multiple input multiple output (MIMO) and channel bonding, which enhance data throughput and range. Backward compatible with 802.11a/b/g, 802.11n can integrate with older devices while experiencing improvement in speed and reliability. This standard became a cornerstone of modern wireless networks, suitable for high-demand applications such as video streaming and online gaming. The 802.11n standard was ratified in 2009.** **802.11ac Standard** **While advancing wireless performance, the 802.11ac standard operates exclusively in the 5 GHz frequency band and delivers data rates up to 1.3 Gbps (Wave 1) and up to 3.47 Gbps (Wave 2). Ratified in 2013, it employs wider channels, advanced modulation techniques, and multi-user MIMO (MU-MIMO) to achieve these high speeds. The 802.11ac standard is backward compatible with 802.11a/n devices, ensuring smooth transitions and enhanced performance for modern applications requiring high bandwidth, such as 4K video streaming and large file transfers.** **802.11ax Standard** **The 802.11ax standard (Wi-Fi 6) operates in the 2.4 and 5 GHz bands, with an additional option for the 6 GHz band in Wi-Fi 6E. This standard significantly enhances efficiency and performance, with potential data rates reaching 9.6 Gbps. It is backward compatible with earlier 802.11 standards, meeting the increasing need for fast, reliable wireless connections in residential and commercial environments. Wi-Fi 6 was ratified in 2019, and Wi-Fi 6E in 2020.** **Wired Transmission** **Wired transmission media, or guided media, refers to the physical cables used to transmit data signals in a network. These media include various types of cables, each with specific characteristics and applications. Key topics under wired transmission media include the 802.3 standards for Ethernet networking, the differences between single-mode and multimode fiber optics, the use of Direct Attach Copper (DAC) cables, including twinaxial cables, and the properties and applications of coaxial cables. Additionally, understanding cable speeds and the distinction between plenum and non-plenum cables is crucial for designing and maintaining efficient and safe wired networks.** **802.3 Standards** **The IEEE developed the 802.3 standards to define the specifications for Ethernet networking. These standards cover various aspects of Ethernet, including physical layer specifications, data rates, and media types. The 802.3 standards ensure interoperability and compatibility among different network devices and provide guidelines for implementing reliable and high-speed wired networks. Understanding these standards is essential for designing, deploying, and managing Ethernet networks in residential and commercial environments, supporting a range of applications from basic Internet access to complex enterprise solutions.** **xBASE-y Ethernet Naming Convention** **The xBASE-y naming convention describes various Ethernet standards and provides information about the technology\'s data transmission speed, the type of transmission, and the physical medium used. This convention describes:** **x - This part of the name specifies the data transmission speed of the Ethernet standard. The number is typically given in megabits per second (Mbps) or gigabits per second (Gbps). For example:** **10BASE-T10 Mbps** **100BASE-TX100 Mbps** **1000BASE-T1000 Mbps (1 Gbps)** **10GBASE-T10 Gbps (10000 Mbps)** **BASE - The term \"BASE\" indicates that the Ethernet standard uses baseband transmission. Baseband transmission means that the entire bandwidth of the cable is used for a single data channel, as opposed to broadband transmission, which can carry multiple signals on different frequencies.** **y - The final part of the name specifies the physical medium (or media type) used for the Ethernet standard and sometimes includes information about the maximum segment length. Common notations include:** **T: Twisted pair cabling** **TX: Twisted pair cabling (with additional specifications)** **SX: Short-wavelength laser over multimode fiber** **LX: Long-wavelength laser over single-mode fiber** **SR: Short range over multimode fiber** **LR: Long range over single-mode fiber** **For example, 1000Base-T denotes an Ethernet implementation that operates at a maximum data transfer rate of 1000 Mbps (1000), uses baseband signal transmission (BASE), and runs over twisted pair cabling (-T).** **Twisted Pair Cables** **Twisted pair copper cables are widely used for data transmission in Ethernet networks. These cables are popular due to their cost-effectiveness, ease of installation, and reliable performance. Copper cables transmit data through electrical signals that travel along the copper conductors. As the signals travel, they can experience attenuation, or signal loss, over distance, reducing the effectiveness and speed of data transmission.** **Twisted Pair Category Ratings** **Category ratings, often referred to as CAT ratings, are specifications for twisted pair cables used in Ethernet networks. These ratings define the cable\'s performance characteristics, such as maximum data transmission speed, frequency, and shielding. Different Ethernet standards require specific Category cables to ensure reliable and efficient data transmission.** **Category ratings for twisted pair cables were developed by the American National Standards Institute (ANSI), the Telecommunications Industry Association (TIA), and the Electronic Industries Alliance (EIA). The TIA and EIA work together to create and publish standards for telecommunications and electronic equipment, including structured cabling systems used in networking.** **ANSI TIA/EIA category standards for twisted pair cabling.** **IEEE 802.3 Specifications for Copper Twisted Pair Cabling** **The IEEE 802.3 standards encompass a wide range of Ethernet networking technologies. The following is an overview of some key 802.3 standards for copper twisted pair cabling and their characteristics:** **IEEE 802.3 Ethernet Standards for twisted pair copper cable.** **10BASE-T and 100BASE-TX** **10BASE-T and 100BASE-TX are two legacy Ethernet standards that played significant roles in the development of local area networks (LANs). 10BASE-T was one of the earliest Ethernet standards and is now largely obsolete, having been replaced by faster technologies. On the other hand, 100BASE-TX, or Fast Ethernet, provided a significant speed boost over 10BASE-T and was widely used in network upgrades. However, it has largely been superseded by Gigabit Ethernet (1000BASE-T) in modern network installations.** **1000BASE-T** **1000BASE-T, commonly known as Gigabit Ethernet, represents a significant advancement in Ethernet technology, providing much faster data transmission compared to its predecessors. This standard allows for seamless integration into existing Ethernet networks, offering a cost-effective upgrade path from older technologies like 10BASE-T and 100BASE-TX. By enhancing network performance and supporting a wide range of applications, 1000BASE-T has become the standard for modern networking.** **40GBASE-T** **40GBASE-T is an advanced Ethernet standard designed to provide ultra-high-speed data transmission, significantly enhancing network performance. It was developed to address the needs of data centers and enterprise networks. This increase in performance supports very high bandwidth requirements, enabling faster data processing, storage access, and virtualization. This standard offers a scalable and efficient upgrade path from lower-speed Ethernet technologies, ensuring compatibility with existing network infrastructure while delivering superior performance.** **Fiber Optic Cables** **Fiber optic cables implemented for Ethernet communication primarily operate in the infrared light spectrum. Light signals travel through the core of the fiber optic cable, reflecting off the cladding to keep the signal contained within the cable. This method allows data transmission over much longer distances without significant signal loss. Surrounding the cladding is the buffer, which provides an additional protective layer for the delicate core. The strength member offers further strength and protection and is designed to withstand the stress of cable installation.** **Basic construction of a single-core fiber optic cable.** **Despite their efficiency, fiber optic cables can still experience attenuation, especially over very long distances. To mitigate this, repeaters or amplifiers boost the signal and maintain data integrity over extended lengths.** **The advantages of fiber optic cable (compared to copper cable) include higher bandwidth, longer distances, immunity to electromagnetic interference, and higher security (it is more difficult to tap without detection).** **On the other hand, the disadvantages of fiber optic cable (compared to copper cable) include higher installation and maintenance costs, fragility, and more complex installation.** **Single Mode Fiber (SMF) and Multimode Fiber (MMF)** **Fiber optic cables are specified based on several characteristics. The mode refers to whether the fiber is Single Mode Fiber (SMF) or Multimode Fiber (MMF). SMF, with its smaller core, allows only one light mode to propagate, making it ideal for long-distance and high-bandwidth applications. In contrast, MMF supports multiple light modes, which are suitable for shorter distances and high-speed data transfer.** **Fiber optic cables use glass or plastic fibers for the core, and glass fibers are preferred for high-performance and long-distance communication due to their lower attenuation and higher bandwidth capabilities. Plastic fibers are used for short-distance and cost-sensitive applications.** **Single mode fiber (SMF) cables typically have a core diameter of 8-10 micrometers, with a cladding diameter of 125 micrometers. It typically uses infrared wavelengths of 1310 nm and 1550 nm.** **MMF cables have larger core diameters, usually 50 or 62.5 micrometers, with a cladding diameter of 125 micrometers. It operates at the 850 nm and 1310 nm infrared wavelengths.** **Core and cladding diameter comparison.** **IEEE 802.3 Specifications for Fiber Optic Cabling** **The IEEE 802.3 standards include the use of fiber optic cabling. The following is an overview of some key 802.3 standards for fiber optic cabling and their characteristics:** **IEEE 802.3 Ethernet Standards for fiber optic cable.** **100BASE-SX, 100BASE-FX** **100BASE-SX is designed for short-distance, high-speed networking within buildings, typically using multimode fiber and operating at an 850 nm wavelength. While it was once a popular choice for Fast Ethernet connections in local area networks (LANs), its use has significantly declined with the widespread adoption of Gigabit Ethernet (1000BASE-SX) and higher-speed standards. Today, 100BASE-SX is largely considered obsolete and is rarely used in new installations.** **100BASE-FX, designed for longer distances and using a 1300 nm wavelength with both multimode and single-mode fibers, was also widely used for extending network connections across campuses and between buildings. Like 100BASE-SX, its use has declined as faster Ethernet standards, such as 1000BASE-FX and 10 Gigabit Ethernet, have become more common.** **Both of these standards may still be found in some legacy systems where upgrading to higher speeds is not necessary or cost-effective.** **1000BASE-SX, 1000BASE-LX** **1000BASE-SX and 1000BASE-FX are both standards for Gigabit Ethernet over fiber optic cables, each suited to different needs. 1000BASE-SX is great for short distances, like within buildings and data centers, using multimode fiber and operating with an 850 nm wavelength. It\'s a cost-effective choice for high-speed networking in these environments. On the other hand, 1000BASE-FX is designed for longer distances, using a 1300 nm wavelength and working with both multimode and single-mode fibers. It\'s ideal for connecting different buildings on a campus or other scenarios where you need a reliable connection over a greater distance.** **10GBASE-SR, 10GBASE-LR** **10GBASE-SR and 10GBASE-LR are both standards for 10 Gigabit Ethernet, each suited for different networking scenarios. 10GBASE-SR is designed for short-range applications, using multimode fiber and operating at an 850 nm wavelength. It is ideal for high-speed connections within data centers and enterprise environments, supporting distances up to 400 meters. On the other hand, 10GBASE-LR is intended for long-range applications, utilizing single-mode fiber and operating at a 1310 nm wavelength. It supports distances up to 10 kilometers, making it suitable for connecting data centers, campuses, and metropolitan area networks.** **Ethernet standards higher than 40 Gbps, such as 100G, 200G, and 400G, are increasingly prevalent in data centers, enterprise networks, telecommunications, and high-performance computing environments. The demand for higher bandwidth, scalability, and performance drives this adoption, with future trends pointing toward even higher speed standards.** **Coaxial Cables** **Coaxial cable consists of a central copper or copper-clad steel conductor that carries the signal, surrounded by a dielectric insulator for spacing, a metallic shield (aluminum or copper foil/braiding) for EMI and RFI protection, and an outer jacket (PVC or polyethylene) for durability and physical protection. This design ensures efficient high-frequency signal transmission with minimal interference and robust durability.** **Basic construction of a coaxial cable. (Source: L-com.com)** **While coaxial cable has largely been replaced by twisted pair and fiber optic cables in many networking applications, it remains relevant in specific areas such as broadband internet access, television and satellite services, in-building signal distribution, and security systems. Its excellent shielding properties and durability continue to make it a valuable medium for certain modern networking and communication needs. The use of appropriate radio grade (RG) ratings, such as RG-6, ensures that coaxial cables meet the specific requirements of these applications.** **Direct Attach Copper (DAC) Cables** **Direct Attach Copper (DAC) cables are high-speed, short-range connections commonly used within data centers. They consist of twinaxial copper cables with integrated transceivers at both ends.** **Basic construction of a twinaxial cable. (Source: Pasternack.com)** **DAC cables support various Ethernet standards and speeds, including 10 Gbps (10GBASE-CU), 40 Gbps (40GBASE-CR4), and 100 Gbps (100GBASE-CR4). Ideal for distances up to 7 meters, DAC cables provide plug-and-play simplicity, low latency, and high performance for high-density networking environments, making them perfect for connecting servers to top-of-rack (ToR) switches, storage systems, and network interface cards.** **Direct Attach Copper (DAC) cable with 10G SFP+ transceivers. (Source: FS.com)** **Direct Attach Copper (DAC) cable connecting two high-speed switches.** **(Source: Cables-Solutions.com)** **Plenum versus Non-Plenum Cable** **A plenum is a space used for circulating air in a building\'s heating, ventilation, and air conditioning (HVAC) systems. Common plenum spaces include the areas above drop ceilings and below-raised floors, which are implemented to facilitate the movement of air. Because air flows through these spaces, any materials used within plenums, such as network cables, must meet strict fire safety standards to prevent the spread of smoke and toxic fumes in the event of a fire.** **(Left) Non-plenum airspace- all air movement is contained in ducts.** **(Right) Plenum airspace- return air movement is in open space. (Source)** **In networking, the terms \"plenum\" and \"non-plenum\" refer to the types of cabling used in different building environments, particularly concerning their fire resistance and safety characteristics.** **Plenum cables are specifically designed for use in plenum spaces. Made with fire-retardant materials that produce less smoke and fewer toxic fumes when burned, these cables meet stringent fire safety standards and are essential for preventing the spread of fire through air ducts. These characteristics make plenum cables more expensive than their non-plenum counterparts. Plenum cables are identified by the markings "CMP" and "OFNP."** **Non-plenum cables are used in areas where air circulation does not occur, such as within walls or between floors. These cables do not have the same fire-resistant properties and are typically made from standard PVC, making them a more cost-effective option for general use where stringent fire safety measures are not required. Non-plenum cables are identified by the markings "CMR" and "OFNR."** **Plenum and non-plenum cables are available in copper twisted pair, coaxial, and fiber optic forms, each designed to meet specific fire safety standards for different installation environments.** **Transceivers** **Transceivers are components in networking that enable the transmission and reception of data signals. They convert electrical signals from network devices into optical signals for transmission over fiber optic cables or vice versa. Transceivers are used in a variety of protocols and come in multiple form factors to accommodate different network requirements.** **Protocols** **Ethernet - Ethernet transceivers are used to connect network devices such as switches, routers, and servers, enabling data communication over Ethernet networks. They support various Ethernet standards, including Fast Ethernet (100 Mbps), Gigabit Ethernet (1 Gbps), 10 Gigabit Ethernet, and higher speeds. Ethernet transceivers are widely used in both local area networks (LANs) and wide area networks (WANs) for reliable and high-speed data transfer.** **Fibre Channel - Fibre Channel transceivers are designed for storage area networks (SANs), providing high-speed data transfer between data storage systems and servers. Fibre Channel is known for its low latency, high reliability, and ability to handle large volumes of data. Fibre Channel transceivers are primarily used in data centers for connecting storage devices.** **Form Factors** **Small Form-Factor Pluggable (SFP) - SFP transceivers are compact, hot-swappable modules that can be easily inserted into network devices. They support data rates up to 4.25 Gbps and are used for both fiber optic and copper connections. This form factor is commonly used in switches, routers, and other networking equipment for both short-range and long-range data transmission. SFPs support various standards, including Ethernet and Fibre Channel.** **10 GbE, LC MMF, Small-Form Factor Pluggable Plus (SFP+) transceiver with dust cap inserted. (Source)** **Quad Small Form-Factor Pluggable (QSFP) - QSFP transceivers are similar to SFPs but support higher data rates, typically ranging from 4 Gbps to 100 Gbps or more. The \"quad\" designation indicates that QSFP modules can support four channels of data simultaneously. They are used in high-density data center environments for applications requiring high bandwidth, such as 40 Gigabit Ethernet (40GbE) and 100 Gigabit Ethernet (100GbE). QSFP modules are ideal for backbone and aggregation layer connections in modern networks.** **40GBASE-LR4, LC SMF, Quad Small-Form Factor Pluggable Plus (QSFP+) transceiver with dust cap inserted. (Source: FS.com)** **Copper Connector Types** **RJ11** **Registered jack (RJ11) connectors are typically used for twisted pair telephone lines and are commonly found in residential and office environments. They have six positions but usually use only two or four of these for wiring. They are primarily used for connecting telephones, modems, and other telecommunication devices to the telephone network.** **RJ45** **Registered jack (RJ45) connectors are the standard for Ethernet networking and are used to connect twisted pair cables to network devices such as switches, routers, and computers. They have eight positions and use all eight for wiring, supporting various Ethernet standards from Fast Ethernet (100 Mbps) to Gigabit Ethernet (1 Gbps) and beyond. RJ45 connectors are commonly used in both residential and commercial environments for establishing wired network connections, including local area networks (LANs) and internet connectivity.** **Registered jacks. (Source: UNC Group)** **Bayonet Neill-Concelman (BNC)** **BNC (Bayonet Neill--Concelman) connectors are used to connect and disconnect coaxial cables quickly. They feature a bayonet mount mechanism that provides a secure connection. BNC connectors are implemented in professional video and radio frequency (RF) applications, including television broadcasting, military equipment, and test instruments.** **F-Type** **F-Type connectors are threaded connectors commonly used with coaxial cables for television and cable internet connections. They provide a reliable and secure connection through a screw-on design. These coaxial connectors are primarily used in residential and commercial applications for connecting cable television, satellite television, and cable modems.** **Coaxial cable connectors. (Source: BNC, F-Type)** **Fiber Optic Connector Types** **Fiber optic cable connectors. SC and SC have dust caps inserted, and LC and MPO have dust caps removed. (Source: FS.com)** **Subscriber Connector (SC)** **SC connectors are square-shaped, push-pull connectors known for their ease of use and reliability. They feature a simple push-in and pull-out mechanism, making them convenient for quick connections. It can be used for single-mode and multimode fiber optic cables. SC connectors are often used with Gigabit Ethernet and 10 Gigabit Ethernet.** **Lucent Connector (LC)** **LC connectors are smaller, compact connectors with a push-pull design similar to SC connectors. They have a latch mechanism for secure connections and are higher density, making them ideal for high-density applications. LC connectors are used with both single-mode and multimode fiber optic cables and are implemented for Gigabit Ethernet and 10 Gigabit Ethernet.** **Straight Tip (ST)** **ST connectors are round, bayonet-style connectors that require a twist and lock mechanism. They are one of the oldest types of fiber optic connectors and are known for their ruggedness. They are primarily used with multimode fiber optic cables but can also be used with single-mode fibers. The ST connector is considered a legacy option, primarily due to its older design and the shift towards more compact and higher-density connectors like LC. Historically, this connector was used for Fast Ethernet and Gigabit Ethernet implementations.** **Multi-Fiber Push-On MPO)** **The MPO (Multi-Fiber Push-On) connector is a high-density fiber optic connector extensively used in modern networking, especially in applications requiring high bandwidth and efficient space utilization. It is designed to handle multiple optical fibers, typically 12 or 24, within a single rectangular ferrule, enabling dense connections. The MPO connector, featuring a push-pull latching mechanism, allows for easy insertion and removal. It is commonly used in 40 Gigabit Ethernet and 100 Gigabit Ethernet applications. MPO connectors are prevalent in data centers, high-speed networks, and environments where space is limited and high bandwidth is critical. They are often used for backbone and horizontal cabling, as well as in parallel optics applications.** **802.11 Standards** The **802.11 standards**, created by the Institute of Electrical and Electronics Engineers (IEEE), are the guidelines for wireless local area network (WLAN) communication. These standards define the protocols and technologies for wireless networking, ensuring that different devices work together seamlessly. The 802.11 family covers frequency bands, data rates, modulation techniques, and security protocols. By following these standards, manufacturers can produce wireless networking equipment that functions well in various settings, whether a home network, a large enterprise, or a public Wi-Fi hotspot. Each new version of the 802.11 standards brings speed, range, reliability, and security improvements to meet the growing demand for wireless connectivity. Wireless radio technology is essential for transmitting and receiving data over the air through electromagnetic waves. These radios are the core components of wireless communication systems, enabling device communication without physical connections. By converting digital data into radio waves, wireless radios transmit these waves through the air to be received by another radio, which then converts them back into digital data. This technology is ubiquitous in modern communication systems, including Wi-Fi, Bluetooth, cellular networks, and satellite communications, offering unparalleled flexibility and mobility. Wireless frequency refers to the specific rate at which electromagnetic waves oscillate during transmission. This rate, measured in Hertz (Hz), determines the wave\'s characteristics, such as its ability to penetrate obstacles, range, and data-carrying capacity. Different wireless communication technologies use different frequencies, balancing signal range, data rate, and interference. Lower frequencies generally offer longer ranges and better penetration through obstacles, while higher frequencies can transmit more data over shorter distances. A wireless frequency band is a defined range of frequencies used for transmitting radio waves. Regulatory bodies govern these bands to ensure efficient and interference-free spectrum use. Each band is designated for specific communication types, such as Wi-Fi, cellular networks, and satellite communications. Wi-Fi commonly operates in the 2.4 GHz and 5 GHz bands, each with different trade-offs regarding range, speed, and interference. Additionally, the 6 GHz band has been introduced in Wi-Fi 6E, offering even higher speeds and reduced congestion due to more available channels. The choice of a frequency band significantly impacts the performance and suitability of a wireless communication system for various applications. In wireless communication, a channel is a smaller section within a frequency band set aside for a specific communication path. Channels let multiple devices communicate simultaneously within the same frequency band without interfering with each other. Each channel has its center frequency and bandwidth. Choosing and managing channels effectively is vital to optimizing network performance, minimizing interference, and ensuring reliable communication, especially in environments with many wireless devices. **2.4 GHz Wi-Fi Band** The 2.4 GHz Wi-Fi band is one of the most popular frequency bands for wireless communication. It has a longer range and can penetrate obstacles like walls and furniture, making it perfect for home and small office setups. However, the 2.4 GHz band is often crowded with various devices like microwaves, Bluetooth gadgets, and cordless phones, which can cause a lot of interference. This band supports 14 channels, but because some channels overlap, it can lead to congestion and reduced performance. Despite these drawbacks, the 2.4 GHz band is still widely used because it can cover larger areas with fewer access points. **5 GHz Wi-Fi Band** The 5 GHz Wi-Fi band became popular over the 2.4 GHz band because it has more channels and experiences less interference from commonly found household devices. This band provides faster data rates and better performance, making it attractive for tasks such as streaming videos and online gaming. It offers up to 24 channels that don\'t overlap, helping to reduce network congestion and improve overall performance. Due to the fact it uses a higher frequency, the signals can be more easily blocked by walls and other obstacles, resulting in a shorter transmission range. In these instances, additional access points will be necessary to provide the same coverage as 2.4 GHz. The 5 GHz band excels in environments where high speed and reduced interference are most important. **6 GHz Wi-Fi Band** The 6 GHz Wi-Fi band, introduced with Wi-Fi 6E, is a big step forward in wireless communication. It offers faster speeds and more capacity because it has more spectrum and wider channels. With 59 non-overlapping channels, it cuts down on congestion and interference, making for a smoother and more efficient connection. The higher frequency means faster data transfer rates, which is great for things that need a lot of bandwidth and low latency, like virtual reality and large file transfers. However, similar to the 5 GHz band, the 6 GHz signals don\'t travel as far and are more easily blocked by obstacles. This band is instrumental in densely populated areas and places where devices compete for bandwidth. **802.11a Standard** The 802.11a standard was one of the earliest wireless standards officially ratified in 1999. Operating in the 5 GHz frequency band, 802.11a has a maximum theoretical data transfer rate of 54 Mbps. This standard utilizes orthogonal frequency-division multiplexing (OFDM) to efficiently transmit data, reducing interference and improving performance in environments with numerous wireless devices. While its higher frequency allows for faster data transmission, it also results in a shorter range than standards operating in the 2.4 GHz band. The 802.11a standard is particularly suited for enterprise networks and environments where minimizing interference is crucial. **802.11b Standard** The 802.11b standard, ratified in 1999, operates in the 2.4 GHz frequency band and supports a maximum theoretical data rate of up to 11 Mbps. Utilizing direct-sequence spread spectrum (DSSS) technology, 802.11b offers reliable performance but is more susceptible to interference from other devices operating in the same frequency range, such as microwaves and Bluetooth. Despite its slower speeds and potential for interference, 802.11b was widely adopted due to its cost-effectiveness and sufficient performance for early wireless networking needs. **802.11g Standard** The 802.11g standard, ratified in 2003, was designed to overcome the shortcomings of its predecessors. It operates in the 2.4 GHz frequency band and achieves theoretical data rates up to 54 Mbps. By employing orthogonal frequency-division multiplexing (OFDM) or direct-sequence spread spectrum (DSSS), 802.11g combines the best features of 802.11a and 802.11b. This standard is backward compatible with 802.11b devices, ensuring seamless integration and improved speeds in existing networks. The 802.11g standard became a popular choice for both home and enterprise wireless networks due to its balance of speed, range, and compatibility. **802.11n Standard** The 802.11n standard represents a significant leap in wireless networking technology, supporting the 2.4 GHz and 5 GHz frequency bands and offering theoretical data rates of 600 Mbps. Key to its performance are technologies such as multiple input multiple output (MIMO) and channel bonding, which enhance data throughput and range. Backward compatible with 802.11a/b/g, 802.11n can integrate with older devices while experiencing improvement in speed and reliability. This standard became a cornerstone of modern wireless networks, suitable for high-demand applications such as video streaming and online gaming. The 802.11n standard was ratified in 2009. **802.11ac Standard** While advancing wireless performance, the 802.11ac standard operates exclusively in the 5 GHz frequency band and delivers data rates up to 1.3 Gbps (Wave 1) and up to 3.47 Gbps (Wave 2). Ratified in 2013, it employs wider channels, advanced modulation techniques, and multi-user MIMO (MU-MIMO) to achieve these high speeds. The 802.11ac standard is backward compatible with 802.11a/n devices, ensuring smooth transitions and enhanced performance for modern applications requiring high bandwidth, such as 4K video streaming and large file transfers. **802.11ax Standard** The 802.11ax standard (Wi-Fi 6) operates in the 2.4 and 5 GHz bands, with an additional option for the 6 GHz band in Wi-Fi 6E. This standard significantly enhances efficiency and performance, with potential data rates reaching 9.6 Gbps. It is backward compatible with earlier 802.11 standards, meeting the increasing need for fast, reliable wireless connections in residential and commercial environments. Wi-Fi 6 was ratified in 2019, and Wi-Fi 6E in 2020.