Week 5 Quiz Study Guide PDF
Document Details
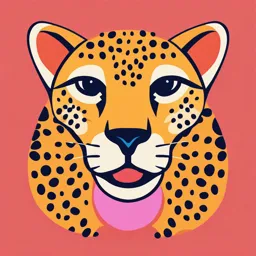
Uploaded by InviolableMorningGlory6288
Tags
Summary
This study guide covers concepts related to pharmacology and drug biochemistry. The guide describes different processes like drug absorption, distribution, metabolism and elimination.
Full Transcript
Week 5 Quiz Study Guide Covers Weeks 1-4 Material Concepts to Know and Apply Assignments 35% Discussions 10% Quizzes 30% (10% each quiz) Fina...
Week 5 Quiz Study Guide Covers Weeks 1-4 Material Concepts to Know and Apply Assignments 35% Discussions 10% Quizzes 30% (10% each quiz) Final Exam 25% Axon – A long, slender projection of a neuron that transmits electrical impulses away from the cell body. Classic synaptic neurotransmission – The process where neurotransmitters are released from a presynaptic neuron into a synapse to activate receptors on a postsynaptic neuron. Concentration-time curve – A graphical representation showing how the concentration of a drug in the body changes over time. Degradation – The breakdown of molecules, such as neurotransmitters or drugs, into inactive components. Dendrite – A branched extension of a neuron that receives signals from other neurons. Drug absorption – The process by which a drug enters the bloodstream from its site of administration. Drug distribution – The movement of a drug from the bloodstream into tissues and organs. Excitation-secretion coupling – The process linking electrical excitation of a cell to the secretion of substances like neurotransmitters or hormones. G-linked proteins – G-protein-coupled receptors (GPCRs) that transmit signals from extracellular molecules to intracellular pathways. Half-life, steady state – Half-life is the time it takes for a drug’s concentration to decrease by half; steady state is when drug intake equals elimination, leading to a stable concentration in the body. Ionic filter – A selective structure in ion channels that permits specific ions to pass through based on size and charge. Ion-gated channels – Proteins in the cell membrane that open or close in response to voltage changes or ligand binding, controlling ion flow. Neuronal gene expression – The process by which neurons regulate the production of proteins based on genetic instructions. Pharmacokinetics – The study of how drugs are absorbed, distributed, metabolized, and excreted by the body. Pharmacodynamics – The study of how drugs exert their effects on biological systems. Phase I metabolism – The modification of drugs by enzymes (e.g., oxidation, reduction, hydrolysis) to increase solubility. Phase II metabolism – The conjugation of drugs with molecules (e.g., glucuronidation, sulfation) to facilitate excretion. Second messengers – Intracellular signaling molecules (e.g., cAMP, Ca²⁺) that relay signals from receptors to target cellular processes. Signal transduction cascade – A series of biochemical reactions converting an extracellular signal into a cellular response. Retrograde neurotransmission – The process where postsynaptic neurons send signals back to presynaptic neurons to regulate neurotransmitter release. Transduction cascades – Molecular pathways that relay and amplify cellular signals from receptors to intracellular targets. Transcription factors – Proteins that regulate gene expression by binding to specific DNA sequences. Volume neurotransmission – The diffusion of neurotransmitters beyond the synapse to influence multiple neurons in a broader area. Key Points Although there are many types of second-messenger systems, there are four main types to know: o G-protein-linked systems (know the G-protein-linked system step by step) o ion-channel-linked systems o hormone linked systems o and neurotropics 1. G-protein-linked systems G-protein-coupled receptors (GPCRs) activate intracellular signaling via G- proteins, which regulate second messengers like cAMP or Ca²⁺. Steps: Ligand binds to GPCR. GPCR activates the associated G-protein (GDP → GTP exchange). G-protein subunits modulate target enzymes or ion channels. Second messengers (e.g., cAMP, IP₃) amplify the signal. Cellular response occurs (e.g., gene expression, ion flux). Signal terminates as GTP is hydrolyzed back to GDP. 2. Ion-channel-linked systems Ligand-gated or voltage-gated ion channels open or close in response to a signal, allowing specific ions (e.g., Na⁺, K⁺, Ca²⁺) to enter or exit the cell, altering cellular activity like neurotransmission or muscle contraction. 3. Hormone-linked systems Hormones (e.g., insulin, cortisol) bind to receptors, triggering intracellular cascades via second messengers like cAMP or direct gene modulation, leading to changes in metabolism, growth, or homeostasis. 4. Neurotropic systems Neurotrophic factors (e.g., BDNF, NGF) activate receptors like Trk (tyrosine kinase) to regulate neuronal survival, differentiation, and synaptic plasticity through signaling cascades such as MAPK, PI3K/Akt, and Ca²⁺ pathways. Know the steps in the signal transduction cascade and the order of those steps. o Signal Reception – A signaling molecule (ligand) binds to a specific receptor on the cell surface or inside the cell. o Transduction – The receptor undergoes a conformational change, activating intracellular signaling pathways (e.g., phosphorylation cascades, second messengers like cAMP or Ca²⁺). o Amplification – The signal is amplified as it passes through multiple signaling intermediates, ensuring a robust response. o Integration & Modulation – Cross-talk with other signaling pathways fine- tunes the response. o Cellular Response – The signal triggers specific cellular effects such as gene expression, metabolic changes, or cytoskeletal rearrangement. o Termination – The signal is deactivated to prevent overactivation and maintain homeostasis (e.g., ligand degradation, receptor desensitization). Explain how the intra/extra cellular messengers are activated. o Intracellular and extracellular messengers are activated when signaling molecules (e.g., hormones, neurotransmitters) bind to specific receptors on the cell surface or within the cell. This binding induces a conformational change in the receptor, which activates intracellular signaling proteins or second messengers like cAMP, IP₃, or Ca²⁺. These messengers then propagate the signal inside the cell, leading to various cellular responses, such as gene expression or changes in cell metabolism. What factors influence gene expression? o Genetic makeup, which determines the presence and function of genes. o Penetrance and expressivity, which affect how often and how strongly a gene is expressed. o Sex-limited inheritance, which restricts the expression of a gene to one sex. o Genomic imprinting, which alters the expression of a gene depending on its parental origin. o Codominance, which allows both alleles of a gene to be observed. o Chromosomal inactivation, which silences one copy of a gene in females. o Environmental factors, such as oxygen, temperature, drugs, chemicals, and light, which can modify the activity of genes and proteins. Know the following terms: agonists, partial agonists, antagonists, and inverse agonists on a receptor site, and enzymes. o Agonists - Molecules that bind to a receptor and activate it, producing a biological response that mimics the action of a natural ligand. o Partial agonists - Molecules that bind to a receptor and activate it but produce a weaker response than full agonists, even at maximum receptor occupancy. o Antagonists - Molecules that bind to a receptor but do not activate it, blocking the receptor from being activated by agonists or endogenous ligands. o iInverse agonists - Molecules that bind to a receptor and produce the opposite effect of an agonist, typically reducing the receptor's baseline activity. o Enzymes - Proteins that catalyze biochemical reactions, speeding up the conversion of substrates into products by lowering the activation energy required for the reaction to proceed. Know about ion channels (the two major ones are a big target for drug therapies): o Ligand-gated ion channels (may also be called inotropic receptors or ion- channel-linked receptors): ▪ Allosteric modulators - Allosteric modulators are molecules that bind to a site on a receptor or enzyme other than the active site, causing a conformational change that alters its activity. Positive allosteric modulators (PAMs) enhance the receptor's function, while negative allosteric modulators (NAMs) reduce its activity. This allows for more precise regulation of receptor function. ▪ The concept of agonists can also be applied to these channels and receptors. Agonists Antagonists Partial agonists Inverse ▪ These channels open in response to the binding of a specific molecule (ligand), such as a neurotransmitter. They are involved in synaptic transmission and cellular signaling. Examples include nicotinic acetylcholine receptors and GABA receptors. Voltage-gated or voltage-sensitive ion channel. ▪ voltage-gated or voltage-sensitive ion channel is a type of ion channel that opens or closes in response to changes in the electrical potential (voltage) across the cell membrane. These channels play a crucial role in the generation and propagation of electrical signals in excitable cells, such as neurons and muscle cells. When the membrane potential reaches a certain threshold, the channel undergoes a conformational change that allows specific ions (e.g., sodium, potassium, calcium) to flow in or out of the cell, which helps propagate action potentials and regulate cellular activity. Understand pharmacokinetics, absorption, distribution, metabolism, and elimination. Absorption: The process by which a drug enters the blood stream after administration. It occurs through various routes, such as oral, intravenous, or transdermal, and is influenced by factors life drug formulation and the presence of food. Distribution the movement of a drug from the bloodstream into various tissues and organs. It depends on factors like blood flow, protein binding, and the drug's ability to cross cell membranes. Metabolism – The process by which the body chemically alters the drug, usually in the liver, to make it easier to excrete. This process often involves the CYP450 enzyme system and can result in either activation or deactivation of the drug. Elimination – The removal of the drug from the body, primarily through the kidneys (urine) or liver (bile), and sometimes through other routes like sweat or breath. The drug's half-life plays a key role in how long it stays in the system before being eliminated. Explain the CYP450 system and be able to identify whether CYP450 system is a substrate, inducer, or inhibitor. o The CYP450 system (Cytochrome P450) is a group of enzymes found primarily in the liver, responsible for the metabolism of various drugs, toxins, and endogenous substances. These enzymes play a crucial role in the oxidation of compounds, facilitating their elimination from the body. o There are three main actions of the CYP450 system related to drug metabolism: substrates, inducers, and inhibitors: ▪ Substrate - A substance (often a drug) that is metabolized by a specific CYP450 enzyme. When a drug is a substrate of a CYP450 enzyme, it undergoes transformation or breakdown by that enzyme. Example: Warfarin is a substrate of CYP2C9. ▪ Inducer - A substance that increases the activity of a CYP450 enzyme, leading to enhanced metabolism of its substrates. This often results in lower plasma levels of the substrate drug, potentially reducing its therapeutic effect. Example: Rifampin is an inducer of several CYP450 enzymes (e.g., CYP3A4). ▪ Inhibitor - A substance that decreases the activity of a CYP450 enzyme, leading to slower metabolism of its substrates. This can result in higher plasma concentrations of the substrate, increasing the risk of adverse effects. Example: Grapefruit juice inhibits CYP3A4, which can increase levels of certain medications, like statins. o Understanding whether a drug is a substrate, inducer, or inhibitor of CYP450 enzymes is important for predicting drug interactions and tailoring treatment regimens Know and apply the implications of pharmacodynamics and steady state: An inducer “induces,” or makes, the enzymes work more diligently to metabolize the substrate. Therefore, drug levels of the substrate would be lower than expected and you would likely have to consider a higher dose. An inhibitor “inhibits” the enzyme's ability to metabolize a drug as fast as it normally would, and you would have to consider cutting the dose down of a substrate. Anxiety and Sleep/Wake Explain pathophysiology of anxiety disorders. o The pathophysiology of anxiety disorders involves neurotransmitter imbalances (especially serotonin, norepinephrine, and GABA), autonomic nervous system dysfunction (overactive fight-or-flight responses), and hyperactivity of the amygdala, leading to exaggerated fear responses. Cognitive factors like negative thought patterns and avoidance behaviors also play a role. Additionally, genetic predisposition and environmental stressors, such as trauma, can trigger or worsen anxiety disorders. Know function and purpose of brain neuroanatomy and circuitry relating to anxiety spectrum disorders: Amygdala and hippocampus relationship (how does this affect anxiety)? o The amygdala processes fear and triggers the body's stress response, while the hippocampus helps assess context and past experiences. In anxiety, an overactive amygdala and a compromised hippocampus (due to stress or trauma) lead to exaggerated fear responses and difficulty properly assessing threats, contributing to heightened anxiety Know the amygdala’s relationship between brain regions (locus coeruleus, ralphe nucleus, para brachial nucleus, basal ganglia, cerebellum, thalamus, and brainstem) o The amygdala interacts with several brain regions to regulate emotional and physiological responses. It connects with the locus coeruleus for norepinephrine release, the raphe nucleus for serotonin modulation, and the parabrachial nucleusfor controlling respiratory and cardiovascular reactions. The amygdala also affects the basal ganglia (behavioral responses), cerebellum (motor adjustments), thalamus (sensory processing), and brainstem (autonomic responses), playing a key role in the "fight or flight" reaction. Dysfunction in these pathways can contribute to anxiety disorders. Limbic system o The limbic system plays a key role in anxiety disorders by regulating emotions and stress responses. Hyperactivity in the amygdala leads to exaggerated fear, while impaired function of the hippocampus and reduced regulation from the prefrontal cortex contribute to heightened anxiety. The hypothalamus and anterior cingulate cortex also play roles in stress responses and emotional regulation. Dysfunction in these areas leads to excessive worry, fear, and physical symptoms of anxiety. Noradrengic pathway influence of anxiety, location of noreprinephrine o The noradrenergic pathway, with norepinephrine produced in the locus coeruleus (in the PONS of the brainstem), regulates arousal and stress responses. Overactivation of this system can contribute to heightened anxiety, leading to symptoms like increased heart rate and nervousness. Dysregulation of norepinephrine signaling, particularly from the locus coeruleus, is closely linked to anxiety disorders. Explain the pathophysiology of insomnia. o Insomnia is caused by a combination of factors, including neurotransmitter imbalances (like GABA, serotonin, and norepinephrine), disrupted circadian rhythms, and heightened arousal (physical and mental). These factors interfere with the ability to fall asleep or stay asleep. Additionally, negative sleep-related thoughts and behaviors can worsen insomnia, making it more persistent. Know what and why specific classifications of pharmaceuticals are the first line of treatment for anxiety spectrum disorders. o The first-line pharmaceutical treatments for anxiety spectrum disorders are primarily selective serotonin reuptake inhibitors (SSRIs) and serotonin- norepinephrine reuptake inhibitors (SNRIs). These medications are preferred because: o SSRIs (e.g., fluoxetine, sertraline, escitalopram) increase serotonin levels in the brain by inhibiting its reuptake, which helps regulate mood and anxiety. They are widely used because they have a favorable side effect profile compared to other antidepressants. o SNRIs (e.g., venlafaxine, duloxetine) increase both serotonin and norepinephrine levels, targeting key neurotransmitters involved in mood regulation and the body's stress response. o Both classes of drugs are effective for anxiety disorders because they help balance neurotransmitter levels, which are often dysregulated in conditions like generalized anxiety disorder (GAD), panic disorder, and social anxiety disorder. They also help treat co-occurring conditions, such as depression, which commonly coexist with anxiety disorders Why do first line treatments for anxiety spectrum disorders also treat MDD? o First-line treatments for anxiety spectrum disorders, such as selective serotonin reuptake inhibitors (SSRIs) and serotonin-norepinephrine reuptake inhibitors (SNRIs), also treat major depressive disorder (MDD) because both conditions share common underlying neurobiological mechanisms, particularly involving serotonin and norepinephrine dysregulation. These medications help restore the balance of neurotransmitters in the brain, which can alleviate symptoms of both anxiety and depression. The overlap in their pathophysiology makes these treatments effective for both disorders. Explain seizure risk and benzodiazepines. o Benzodiazepines are commonly prescribed to treat anxiety, insomnia, and seizures due to their calming effect on the central nervous system. However, there is a risk of seizure under certain conditions: ▪ Withdrawal – Abrupt cessation or rapid dose reduction of benzodiazepines, especially after long-term use, can lead to withdrawal seizures. This is because benzodiazepines enhance GABA (an inhibitory neurotransmitter), and sudden removal disrupts the balance between inhibitory and excitatory signals in the brain, leading to hyperexcitability and seizures. ▪ Overuse or Toxicity – Excessive use or overdose of benzodiazepines can depress the central nervous system too much, but in rare cases, particularly with underlying conditions or when combined with other drugs (e.g., alcohol), it can paradoxically increase the risk of seizures. ▪ Pre-existing Seizure Disorders – In individuals with a history of epilepsy or seizure disorders, certain benzodiazepines might not be effective in controlling seizures, or may even trigger them if used improperly. While benzodiazepines themselves are generally used to manage acute seizures, especially in conditions like status epilepticus, proper dosage, and monitoring are critical to minimize the risk of seizures. Explain psychotropics for insomnia and daytime sleeping. o Psychotropics for insomnia include benzodiazepines, non-benzodiazepine hypnotics, antidepressants with sedative properties, and melatonin receptor agonists, all of which help induce sleep by affecting neurotransmitters or regulating the sleep-wake cycle. For daytime sleepiness (hypersomnia), stimulants and certain antidepressants are used to promote wakefulness by increasing norepinephrine and dopamine activity. These medications are typically used for short-term relief, with a focus on behavioral strategies for long-term management. Explain the long-term effects of insomnia. o long-term insomnia can lead to cognitive impairment, mental health issues like depression and anxiety, and increased risks of chronic diseases such as heart disease, diabetes, and stroke. It weakens the immune system, impairs physical functioning, and decreases quality of life due to fatigue and reduced social or work performance. Managing insomnia is essential to prevent these negative effects. Explain non-pharmacologic strategies for insomnia. o Non-pharmacological strategies for insomnia including CBT-I, sleep hygiene, relaxation techniques, exercise, light exposure therapy, dietary adjustments and behavioral strategies. These approaches help regulate sleep patterns, reduce anxiety and improve overall sleep quality without medication. Know FDA approval, mechanism of action, purpose, and side effects (SE) of the following psychotropics: clonazepam (Klonpin) o Yes; enhances the effect of GABA (brain’s primary inhibitor). Binds to GABA-A receptor – hyperpolarizing neurons, reducing their excitability and producing sedative, anxiolytic muscle relaxant an anticonvulsant effects. For Sz, panic disorder, Insomnia. SE – drowsiness, dizziness, fatigue, impaired coordination, respiratory depression, cognitive impairement, tolerance and addiction. temazepam (Restoril) o Yes; same as clonazepam. Z drugs purpose (think half-life) o Yes; Induce sleep, maintain sleep; SE – dizziness, headache, drowsiness, sleepwalking, memory impairment, hallucinaitons, dependence o Zolpidem (Ambien) – 2-3 hours o Zaleplon (Sonata) 1 hour o Eszopiclone (Lunesta) 6-9 hours modafinil (Provigil) o Approved for Narcolepsy, sleep apnea o Promotes wakefulness by stimulating dopamine, NE and histamine in the brain – possibly inihibiting dopamine reuptake. o SE: headaches, Nausea, dizziness, anxiety, insomnia, inc BP, Liver problins, Agression and Mania quetiapine (Seroquel) o approved for schizophrenia, BD, MDD; OFF USE for insomnia o blocks dopamine and serotonin receptors; sedative effects related to Histamine and Alpha-adrenergic receptor. o SE: Drowsiness, dizziness, weight gain, EPS, tardic dyskinesia, ortho HOTN trazadone (Dresyel) o approved for Depression; OFF USE for insomnia o serotonin antagonist and reuptake inhibitor. It blocks serotonin (5-HT2) receptors and inhibits serotonin reuptake. This leads to sedative effects, often used off-label for insomnia. o SE: Drowsiness, dry mouth, dizziness, headache, nausea, ortho HOTN, arrythimas, serotonergic drugs diphenhydramine (Benadryl) o Approved for allergic reactions; off USE for sleep aid o First-generation antihistamine that blocks H1 histamine receptors in the brain. Its sedating effect results from the inhibition of histamine- mediated wakefulness. o SE: Confusion, : Drowsiness, dry mouth, blurred vision, constipation, and urinary retention; Confusion (especially in elderly), memory impairment, tachycardia, severe allergic reaction (rare), and anticholinergic effects. Ramelteon (Rozerem) o Approved for Insomnia. o Melatonin receptor agonist that selectively binds to MT1 and MT2 melatonin receptors in the brain, regulating the sleep-wake cycle, particularly for sleep onset. o SE: Drowsiness, dizziness, fatigue, headache, and nausea; Endocrine effects (e.g., increased prolactin levels), allergic reactions, and sleep- driving behaviors. hydroxyzine pamoate (Vistaril) o Approved for Anxiety, allergies and sedation; off use for sleep o Antihistamine that blocks H1 histamine receptors and has sedative and anxiolytic properties. It is often used for anxiety and short-term insomnia. o SE: Drowsiness, dry mouth, dizziness, and headache; Confusion (especially in the elderly), sedation, tachycardia, and severe allergic reactions. buspirone (Buspar) o Approved GAD; off use in insomnia o Mechanism: A 5-HT1A partial agonist and dopamine D2 antagonist. It reduces serotonergic activity in the brain, providing anxiolytic effects without causing sedation, unlike benzodiazepines. o SE: Dizziness, nausea, headache, and nervousness; Serotonin syndrome (rare, especially if combined with other serotonergic drugs), tachycardia, and hypertension. prazosin (Minipress) o Approved HTN; off use for nightmares and sleep disturbance o Mechanism: Alpha-1 adrenergic antagonist that blocks norepinephrine receptors, leading to vasodilation and reduced blood pressure. Used off-label for nightmares in PTSD due to its effects on adrenergic pathways. o SE: Dizziness, drowsiness, headache, and orthostatic hypotension (especially with dose initiation); Syncope (fainting), tachycardia, and priapism (rare). Clonidine (Catapres) o Approved HTN and ADHD; off use for insomnia o Mechanism: Alpha-2 adrenergic agonist that reduces sympathetic nervous system activity, leading to sedation and reduced anxiety. It is often used for sleep disturbances related to withdrawal or anxiety. o SE: Sedation, dry mouth, dizziness, constipation, and fatigue; Severe hypotension, bradycardia, and rebound hypertension (if discontinued abruptly). Propranolol (Inderal) o HTN, Angina, Arrythmias and migraine prevention; off use for anxiety o Mechanism: Non-selective beta-blocker that blocks beta-1 and beta-2 adrenergic receptors, reducing heart rate and blood pressure. It also reduces symptoms of performance anxiety by counteracting the physical symptoms of anxiety (e.g., tachycardia, tremors). o SE: Fatigue, dizziness, cold hands and feet, nausea, and bradycardia; Bronchospasm (especially in asthmatic patients), heart block, hypotension, and depression Know the purpose and if there’s a deficit or surplus of gaba, glutamate, M1, serotonin, norepinephrine, dopamine, histamine, acetylcholine receptors regarding anxiety and insomnia (understand the neuropeptide orexin). Neurotransmitters and Their Role in Anxiety & Insomnia 1. GABA (Gamma-Aminobutyric Acid) a. Purpose: Primary inhibitory neurotransmitter; promotes relaxation, reduces anxiety, and induces sleep. b. Deficit: Increased anxiety, insomnia, hyperarousal, and risk of seizures. c. Surplus: Excessive sedation, drowsiness, cognitive impairment. 2. Glutamate a. Purpose: Primary excitatory neurotransmitter; involved in alertness, cognition, and stress response. b. Deficit: Cognitive dysfunction, decreased alertness. c. Surplus: Heightened anxiety, insomnia, excitotoxicity, and neurodegeneration. 3. M1 (Muscarinic Acetylcholine Receptors) a. Purpose: Regulate REM sleep, memory, and autonomic functions. b. Deficit: Disrupted REM sleep, cognitive impairment, drowsiness. c. Surplus: Overactivation may lead to excessive REM sleep, vivid dreams, or nightmares. 4. Serotonin (5-HT) a. Purpose: Modulates mood, anxiety, and sleep; precursor for melatonin (sleep hormone). b. Deficit: Increased anxiety, depression, insomnia, poor stress regulation. c. Surplus: Can cause excessive sedation, serotonin syndrome, and blunted emotions. 5. Norepinephrine (NE) a. Purpose: Regulates alertness, arousal, and stress response. b. Deficit: Fatigue, poor focus, depression, low energy. c. Surplus: Hyperarousal, anxiety, insomnia, racing thoughts. 6. Dopamine (DA) a. Purpose: Modulates mood, motivation, and wakefulness. b. Deficit: Low motivation, anhedonia, fatigue, poor attention. c. Surplus: Hyperactivity, agitation, insomnia, restlessness. 7. Histamine a. Purpose: Regulates wakefulness, arousal, and immune response. b. Deficit: Excessive sleepiness, cognitive dulling. c. Surplus: Increased wakefulness, insomnia, allergic responses. 8. Acetylcholine (ACh) a. Purpose: Involved in REM sleep, memory, learning, and autonomic nervous system regulation. b. Deficit: Memory impairment, reduced REM sleep, drowsiness. c. Surplus: Overstimulation, excessive REM sleep, vivid dreams. Summary Deficits in GABA and serotonin contribute to anxiety and insomnia. Excess in norepinephrine, glutamate, and histamine can worsen anxiety and disrupt sleep. Dopamine, acetylcholine, and M1 receptors play a complex role in sleep-wake balance. Neuropeptide Orexin (Hypocretin) Purpose: Regulates wakefulness, arousal, and appetite; helps maintain sleep-wake stability by preventing inappropriate transitions into sleep. Deficit: Associated with narcolepsy, excessive daytime sleepiness, and fragmented sleep. Surplus: Linked to insomnia, hyperarousal, and anxiety, as it promotes wakefulness and alertness. 💡 Clinical Relevance: Orexin antagonists (e.g., Suvorexant, Lemborexant) are used to treat insomnia by reducing wakefulness signals.