Bio Exam 2 Study Guide PDF
Document Details
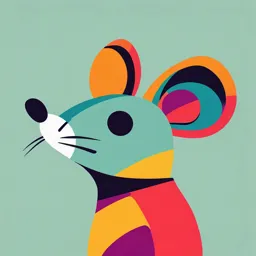
Uploaded by TranquilPeninsula
Mesa Community College
Kylee McKinney
Tags
Summary
This study guide covers the topics of diffusion, osmosis, and selective permeability for a Bio exam. The guide provides definitions, diagrams, and explanations of these concepts..
Full Transcript
BIO EXAM 2 Study guide By: Kylee McKinney The movement of particles (atoms, ions or molecules) from a region in which they are in higher concentration to DIFFUSION regions of lower concentration Extracellular fluid having a...
BIO EXAM 2 Study guide By: Kylee McKinney The movement of particles (atoms, ions or molecules) from a region in which they are in higher concentration to DIFFUSION regions of lower concentration Extracellular fluid having a Hypertonic higher osmolarity than the Water moves across a membrane cell’s cytoplasm. Water leaves from areas of lower solute OSMOSIS concentration to areas of higher the cell. CELL SHRINKS solute concentration. The extracellular fluid has a lower osmolarity than the fluid inside the Hypotonic cell. Water enters the cell. CELL EXPANDS Isotonic The extra cellular fluid has the same osmolarity as the cell. Factors that 1. Extent of concentration gradient Affect 2. Mass of molecules diffusing Diffusion 3. Temperature 4. Solvent density 5. Solubility SELECTIVE PERMEABILITY 6. Surface area and plasma membrane thickness Plasma membranes allow SOME 7. Distance travelled substances to pass through but not all. Defines the cell PLASMA MEMBRANE Outlines its borders Determines the nature of its interaction with its environment. FLUID MOSAIC MODEL Describes the plasma membrane structure as a mosaic of components - phospholipids, cholesterol, proteins, and carbs - that gives the membrane fluid character. AMPHIPATHIC Dual-loving. meaning a molecule that has PHOSPHOLIPID BILAYER both hydrophilic (water-attracting) and a fundamental structure that forms the cell hydrophobic (water-repelling) parts membrane in living organisms. It consists of two layers of phospholipid molecules, each composed of a hydrophilic (water-attracting) “head” and two hydrophobic (water- repelling) “tails.” MEMBRANE STRUCTURE A hydrophilic head that is attracted to water. TYPES Hydrophobic tails that repel water. 1. Diffusion 2. Facilitated diffusion This dual nature allows phospholipids to form the bilayer structure of the 3. Osmosis plasma membrane, with the hydrophilic heads facing the aqueous environments inside and outside the cell, while the hydrophobic tails face each other, forming the interior of the membrane. This property is essential for the membrane’s function as a selective barrier. PASSIVE TRANSPORT Passive transport is the movement of substances across a cell membrane without the need for energy input from the cell. It occurs down a concentration gradient, meaning substances move from an area of higher concentration to an area of lower concentration until equilibrium is reached. The process relies on the natural kinetic energy of molecules. FACILITATED DIFFUSION Larger or polar molecules (such as glucose or ions) require the help of membrane proteins (like channels or carriers) to move across the membrane, still without energy. AQUAPORIN a type of integral membrane protein that forms channels in the cell membrane to facilitate the transport of water molecules in and out of the cell. These channels are highly selective, allowing only GATED PROTEIN CHANNEL water to pass through while preventing the passage of ions or other small molecules. Aquaporins play a crucial role in maintaining A gated protein channel is a type of membrane water balance in cells and are essential in processes such as osmosis, protein that opens or closes in response to specific where water moves in response to concentration gradients. stimuli, allowing or preventing the passage of ions or molecules across the cell membrane. These channels are highly selective, usually permitting only certain ions CARRIER PROTEIN (such as sodium, potassium, calcium, or chloride) or A channel protein is a type of membrane protein that molecules to pass through when open. forms a passageway or pore in the cell membrane, allowing specific ions or molecules to move across it. ACTIVE TRANSPORT process by which cells move molecules or ions across the cell membrane against their concentration gradient, from an area of lower concentration to an area of higher ATP - adenosine triphosphate concentration. primary energy carrier in cells. It stores and provides energy for many biological processes, such as muscle contraction, nerve PRIMARY ACTIVE TRANSPORT impulse transmission, and chemical reactions. type of active transport where energy is directly used from ATP to move molecules or ions across a cell membrane against their concentration gradient (from lower to higher concentration). This process requires membrane proteins, often called pumps, which hydrolyze ATP to obtain the necessary energy. SECONDARY ACTIVE TRANSPORT Does not require ATP directly. Instead it is the movement due to the electrochemical gradient that is established by the primary active transport that is used. Carrier Proteins in Active Transport Uniporter Carries one specific ion or molecule Carries two different ions or molecules, both in Symporter the SAME direction Antiporter Carries two different ions or molecules but in different directions. SODIUM-POTASSIUM PUMP NA+/K+ Found in animal cells. Main function is to maintain the proper balance of Sodium and potassium ions inside and outside the cel. This is essential for various cellular processes like nerve impulse transmission, muscle contraction and maintaining cell volume. Drive secondary active transport - the sodium gradient created by the pump powers the secondary system. HOW IT WORKS: 1. Binding of sodium ions: The pump binds three sodium ions (Na⁺) from ELECTROCHEMICAL GRADIENT the inside of the cell. the combination of two forces that drive the 2. ATP hydrolysis: The pump then hydrolyzes one molecule of ATP, movement of ions across a cell membrane: breaking it down into ADP and inorganic phosphate. This provides the energy 1. Chemical gradient (concentration gradient): This required for the pump to change its shape. is the difference in the concentration of a specific ion 3. Sodium ions released: Using the energy from ATP, the pump changes (like sodium, Na⁺, or potassium, K⁺) across the its conformation and moves the three sodium ions out of the cell, against membrane. Ions tend to move from areas of high their concentration gradient (since Na⁺ is typically higher outside the cell). concentration to areas of low concentration, which is 4. Binding of potassium ions: In its new shape, the pump binds two the chemical driving force. 2. Electrical gradient (membrane potential): This potassium ions (K⁺) from outside the cell. refers to the difference in charge (voltage) across 5. Return to original shape: The pump releases the phosphate group, the cell membrane. Since ions are charged particles, causing it to return to its original shape, which transports the two they are influenced by the electrical potential across potassium ions into the cell, against their concentration gradient (since K⁺ is the membrane. Positively charged ions (like Na⁺ or higher inside the cell). K⁺) are attracted to negatively charged areas, and 6. Cycle repeats: The pump is now ready to bind sodium ions again, vice versa for negatively charged ions. and the process continues. 3 TYPES ENDOCYTOSIS 1. PHAGOCYTOSIS cellular process by which a cell takes in materials from 2. PINOCYTOSIS its external environment by engulfing them with its 3. RECEPTOR-MEDIATED ENDOCYTOSIS plasma membrane. This process involves the cell membrane folding around the substance to form a vesicle, which is then brought into the cell. Endocytosis is essential for the uptake of large molecules, particles, or even other cells that cannot pass through the cell membrane by passive transport. PHAGOCYTOSIS “CELL EATING” PINOCYTOSIS “CELL DRINKING” In this process, the cell engulfs large particles, such as bacteria or This type of endocytosis involves the intake of debris. The membrane surrounds the particle, forming a vesicle liquids and dissolved solutes. The cell membrane known as a phagosome, which is then internalized and typically forms small vesicles that capture extracellular digested by lysosomes. fluid and bring it into the cell. RECEPTOR-MEDIATED ENDOCYTOSIS This is a selective form of endocytosis where specific molecules, such as hormones or nutrients, bind to receptors on the cell surface. Once bound, the membrane invaginates and forms a vesicle that carries the bound molecules into the cell. This process allows the cell to take in specific substances in larger amounts. Exocytosis process by which cells expel materials from the cell into the external environment. In this process, vesicles containing substances (such as proteins, waste products, or neurotransmitters) fuse with the plasma membrane, releasing their contents outside the cell. Exocytosis is the reverse of endocytosis, where substances are brought into the cell. Steps in Exocytosis: 1. Vesicle formation: Inside the cell, materials are packaged into vesicles, typically formed by the Golgi apparatus or endosomes. 2. Vesicle transport: The vesicle is transported toward the plasma membrane. 3. Membrane fusion: The vesicle membrane fuses with the plasma membrane. 4. Release of contents: Once the vesicle merges with the membrane, its contents are released into the extracellular space. 1. Catabolism: The process of breaking down complex molecules METABOLISM into simpler ones, releasing energy in the process. For example, all the chemical reactions that occur within a cell during cellular respiration, glucose is broken down into carbon to maintain life. These reactions are responsible for dioxide and water, releasing energy stored in ATP (adenosine converting nutrients into energy, building cellular triphosphate), which the cell uses for various functions. structures, and breaking down waste products. Metabolism can be divided into two main categories: 2. Anabolism: The process of building up complex molecules from simpler ones. This requires energy and is essential for growth, repair, and the synthesis of essential molecules like proteins, nucleic acids, and lipids. An example is the synthesis of proteins from amino acids. ACTIVATION ENERGY The minimum amount of energy required to start a chemical reaction. It represents the energy barrier that must be overcome for reactants to be converted into products. In both biological and chemical reactions, activation energy is necessary to break the initial bonds between atoms in the reactants and form new ones in the products. Enzyme a biological catalyst that speeds up chemical reactions in living organisms without being consumed in the process. Enzymes are AcTIVE SITE typically proteins (though some RNA molecules can act as The specific region on the enzyme where the substrate enzymes, called ribozymes) that facilitate various metabolic binds. It is the part of the enzyme that directly reactions necessary for life, such as digestion, energy production, interacts with the substrate and facilitates the chemical DNA replication, and more. reaction. The active site has a unique shape and chemical HOW IT WORKS environment that fits the substrate like a “lock and 1. Substrate binding: The substrate binds to the key,” ensuring that only the correct substrate can bind and be catalyzed. active site of the enzyme, forming an enzyme-substrate complex. SUBSTRATE 2. Catalysis: Once bound, the enzyme facilitates the The specific reactant molecule that an enzyme acts conversion of the substrate into products by lowering the upon in a chemical reaction. During an enzymatic activation energy required for the reaction. reaction, the substrate binds to the enzyme’s active 3. Product release: After the reaction occurs, the site, forming an enzyme-substrate complex, which allows the enzyme to catalyze the conversion of the products are released from the active site, and the enzyme substrate into products. is free to catalyze another reaction. ENZYME INHIBITOR a molecule that binds to an enzyme and decreases its activity, either by blocking the enzyme’s active site or by altering its shape. Enzyme inhibitors prevent the enzyme from catalyzing its reaction with the substrate, effectively slowing down or stopping the reaction. CELLULAR RESPIRATION 1. GLYCOLYSIS 3 MAIN TYPES a biochemical process in which cells convert glucose and 2. CITRIC ACID CYCLE oxygen into energy (in the form of ATP), carbon dioxide, 3. ELECTRON TRANSPORT CHAIN AND and water. This process is essential for producing the OXIDATIVE PHOSPHORYLATION energy required for various cellular activities, such as growth, repair, and maintenance of cellular functions. f C hao + 6 O To 6 CO2 oct + 6 H O +f ATP Glucose Oxygen Carbon dioxide Water Energy OXIDATION Loss of electrons Loss of energy Gain of electrons REDUCTION Gain in energy and H+ GLYCOLYSIS ANAEROBIC AEROBIC first stage of cellular respiration, where one glucose molecule (a Does NOT use oxygen USES Oxygen six-carbon sugar) is broken down into two molecules of pyruvate (a three-carbon compound). It occurs in the cytoplasm and does not require oxygen, making it an anaerobic process. Glycolysis consists of ten steps, divided into two phases: the ENERGY ENERGY REQUIRING PHASE REQUIRING phase and the ENERGY RELEASING phase. STEPS 1-5 In this phase, energy is produced in In this phase, the cell uses energy in the form of ENERGY RELEASING PHASE the form of ATP and NADH. ATP to prepare glucose for breakdown STEPS 6-10 Step 1: Phosphorylation of Glucose Enzyme: Hexokinase Step 6: Oxidation and Phosphorylation of G3P Process: A phosphate group from ATP is added to glucose, forming glucose-6-phosphate. Enzyme: Glyceraldehyde-3-phosphate dehydrogenase Energy Use: 1 ATP is used. Process: Each G3P is oxidized, transferring electrons to NAD⁺ to form NADH. A phosphate group is also added Step 2: Isomerization of Glucose-6-Phosphate to G3P, forming 1,3-bisphosphoglycerate. Energy Production: 2 NADH molecules are produced (one for each G3P). Enzyme: Phosphoglucose isomerase Step 7: Transfer of Phosphate to ADP Process: Glucose-6-phosphate is rearranged into fructose-6-phosphate. Enzyme: Phosphoglycerate kinase Step 3: Phosphorylation of Fructose-6-Phosphate Process: A phosphate group from 1,3-bisphosphoglycerate is transferred to ADP, forming ATP and 3- Enzyme: Phosphofructokinase phosphoglycerate. Process: Another phosphate group from ATP is added to fructose-6-phosphate, forming Energy Production: 2 ATP molecules are produced (one per G3P). fructose-1,6-bisphosphate. Step 8: Isomerization of 3-Phosphoglycerat Energy Use: 1 ATP is used. Enzyme: Phosphoglycerate mutase Step 4: Cleavage of Fructose-1,6-Bisphosphate Process: 3-phosphoglycerate is rearranged into 2-phosphoglycerate. Enzyme: Aldolase Step 9: Dehydration of 2-Phosphoglycerate Process: Fructose-1,6-bisphosphate is split into two three-carbon molecules: Enzyme: Enolase glyceraldehyde-3-phosphate (G3P) and dihydroxyacetone phosphate (DHAP). Step 5: Isomerization of DHAP Process: Water is removed from 2-phosphoglycerate, forming phosphoenolpyruvate (PEP). Enzyme: Triose phosphate isomerase Step 10: Transfer of Phosphate from PEP to ADP Process: DHAP is converted into G3P, so now there are two molecules of G3P ready to enter Enzyme: Pyruvate kinase the energy payoff phase. Process: The phosphate group from PEP is transferred to ADP, forming ATP and pyruvate. Energy Production: 2 ATP molecules are produced (one per G3P). GLYCOLYSIS SUMMARY Glucose is split into two molecules of pyruvate. Energy use: 2 ATP are consumed during the energy investment phase. Energy production: 4 ATP and 2 NADH are produced in the energy payoff phase. Net gain: 2 ATP (since 4 ATP are produced, but 2 are used) and 2 NADH. CITRIC ACID CYCLE C the second stage of cellular respiration, where the breakdown of glucose continues. Here are the simplified key points: 1. Starts with Acetyl-CoA: The cycle begins when acetyl-CoA (from glucose breakdown) combines with a molecule called oxaloacetate to form citrate. 2. Produces Energy Carriers: During the cycle, energy is captured by producing NADH and FADH₂, which are used later to make a lot of ATP. 3. Releases Carbon Dioxide: Two molecules of CO₂ are released as waste during the process. 4. Makes a Small Amount of ATP: One molecule of ATP (or GTP) is made directly in the cycle. 5. Cycle Repeats: Oxaloacetate is regenerated at the end, so the cycle can start again with a new acetyl-CoA. Outputs per cycle: 3 NADH 1 FADH₂ 1 ATP 2 CO₂ In short, the citric acid cycle breaks down acetyl-CoA to release energy carriers (NADH, FADH₂), a little ATP, and carbon dioxide. These carriers then go on to produce a lot of ATP in the next stage (electron transport chain).