Respiratory System Study Guide PDF
Document Details
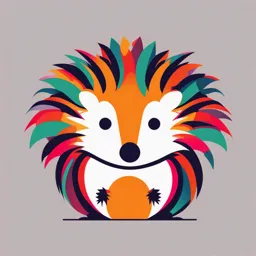
Uploaded by MajesticNovaculite
Tags
Related
Summary
This study guide provides an overview of the respiratory system. It covers various aspects of its structure, function, and processes. The guide also explains the interaction between the components of the system.
Full Transcript
Study Guide- Chapter 23- Respiratory System State the functions of the respiratory system. 1. Gas exchange: The respiratory system facilitates the exchange of oxygen and carbon dioxide between the body and the environment through the process of respiration. 2. Breathing: The respiratory system contr...
Study Guide- Chapter 23- Respiratory System State the functions of the respiratory system. 1. Gas exchange: The respiratory system facilitates the exchange of oxygen and carbon dioxide between the body and the environment through the process of respiration. 2. Breathing: The respiratory system controls the intake of oxygen-rich air (inhalation) and the release of carbon dioxide-rich air (exhalation) to maintain proper levels of oxygen in the blood. 3. Filtration and humidification: The respiratory system filters out airborne particles, such as dust and allergens, and adds moisture to the inhaled air to prevent drying out of delicate lung tissues. 4. Regulation of pH: By controlling the elimination of carbon dioxide from the body, the respiratory system helps regulate the acid-base balance (pH) in the bloodstream. 5. Voice production: The vocal cords within the larynx are responsible for producing sound during speech or singing by vibrating as air passes through them. 6. Sense of smell: The nasal cavity is lined with olfactory receptors that allow us to detect various scents and contribute to our sense of smell. 7. Immune defense: The respiratory system contains specialized cells and mechanisms that help defend against pathogens and foreign substances entering through inhaled air. 8. Regulation of body temperature: During exhalation, warm air is expelled from the body, helping to regulate overall body temperature. 9. Metabolic functions: Through respiration, the respiratory system plays a role in metabolism by providing oxygen needed for cellular energy production (aerobic respiration). 10. Removal of waste products: Along with carbon dioxide, other waste products such as volatile compounds can be eliminated from the body through exhalation. Distinguish between the structural organization and the functional organization of the respiratory system Structural organization of the respiratory system: - Consists of the nasal cavity, pharynx, larynx, trachea, bronchi, and lungs. - The nasal cavity is lined with ciliated epithelium and contains mucus-secreting cells that help filter and humidify inhaled air. - The pharynx serves as a common passage for both air and food. - The larynx houses the vocal cords and plays a role in producing sounds. - The trachea is a tube that connects the larynx to the bronchi and is supported by cartilage rings to maintain its shape. - The bronchi further divide into smaller tubes called bronchioles, which eventually lead to clusters of tiny air sacs called alveoli in the lungs. Functional organization of the respiratory system: - Responsible for oxygen intake and carbon dioxide removal from the body. - Inhalation brings fresh air into the respiratory tract, where it gets filtered, moistened, and warmed before reaching the lungs. - Oxygen diffuses across the thin walls of alveoli into capillaries to be transported by red blood cells to tissues throughout the body. - Carbon dioxide produced during cellular respiration diffuses out of tissues into capillaries, then travels back to the lungs through blood circulation. - Exhalation removes stale air rich in carbon dioxide from the respiratory tract. Describe the structure of the mucous membrane that lines the respiratory tract and the structural changes observed along its length. - The mucous membrane that lines the respiratory tract consists of multiple layers. - The outermost layer is composed of epithelial cells, which provide a protective barrier. - Beneath the epithelium, there is a layer of connective tissue called the lamina propria, which contains blood vessels, nerves, and immune cells. - Within the lamina propria, there are also specialized structures called goblet cells that produce mucus to help trap and remove foreign particles from the airway. - Along the length of the respiratory tract, structural changes can be observed. For example: - In the upper respiratory tract (nose and throat), the mucous membrane may have cilia or hair-like projections that help move mucus and trapped particles out of the airway. - In the lower respiratory tract (trachea, bronchi, and lungs), the mucous membrane becomes thinner and smoother with fewer cilia as it reaches deeper into the lungs. Explain the function of mucus produced by the mucosa - Mucus produced by the mucosa functions as a protective barrier in various body systems, including the respiratory, digestive, and reproductive systems. - It helps to trap and remove foreign particles, such as dust, pathogens, and allergens from entering and damaging the underlying tissues. - The mucus also moisturizes and lubricates the surfaces it covers, preventing dryness and friction-related damage. - Additionally, mucus contains antimicrobial substances that help to inhibit the growth of bacteria and other harmful microorganisms. Describe the structure and function of the nose. - The nose is a prominent facial feature that consists of cartilage, bone, and soft tissue. - It serves as the primary organ of smell, allowing us to detect and distinguish various odors in our environment. - The nose also plays a crucial role in the respiratory system by filtering, warming, and humidifying the air we breathe before it reaches the lungs. - Inside the nose, there are hair-like structures called cilia that help trap dust particles and other foreign substances, preventing them from entering the respiratory system. - The nasal cavity is lined with mucus-producing cells that moisten the air and help remove any harmful bacteria or viruses present in the inhaled air. Provide a general description of the structure and function of the nasal cavity - The nasal cavity is the hollow space located behind the nose and above the mouth. - It is lined with a mucous membrane that helps to moisten and filter the air we breathe. - The nasal cavity contains structures such as turbinates (bony projections) and cilia (tiny hair-like structures), which help to increase the surface area for filtering and warming incoming air. - It also houses olfactory receptors, responsible for our sense of smell. - The nasal cavity serves as a passage for air to enter into the respiratory system, while also playing a role in speech resonance. Describe the structure and function of the four paired paranasal sinuses - The four paired paranasal sinuses are the frontal sinuses, maxillary sinuses, ethmoid sinuses, and sphenoid sinuses. - The frontal sinuses are located above the eyes in the forehead region. They help to reduce the weight of the skull and provide resonance for the voice. - The maxillary sinuses are located on each side of the nose, within the cheekbones. They serve to warm and humidify inhaled air, as well as enhance voice quality. - The ethmoid sinuses are located between the eyes, behind the bridge of the nose. They play a role in filtering and moistening air, as well as contributing to facial shape and structure. - The sphenoid sinuses are located deep within the skull, behind the eyes. They assist in reducing skull weight and providing resonance for speech. Compare the three regions of the pharynx, and describe their associated structures. 1. Nasopharynx: - Located above the soft palate and behind the nasal cavity. - Associated with the following structures: - Pharyngeal tonsils (adenoids): Lymphoid tissue located in the posterior wall of the nasopharynx that helps protect against infections. - Eustachian tubes: Connects the middle ear to the nasopharynx, helping to equalize pressure between the two areas. - Opening of auditory tube: Allows passage of air from nasopharynx to middle ear. 2. Oropharynx: - Situated behind the oral cavity, extending from the soft palate to the epiglottis. - Associated with several important structures: - Palatine tonsils: Located on both sides of the oropharynx; part of the immune system's defense against pathogens entering through the mouth. - Lingual tonsils: Found at the base of the tongue; also contribute to immune response against invading microorganisms. - Epiglottis: A flap-like structure made of elastic cartilage that prevents food and liquids from entering the trachea during swallowing. 3. Laryngopharynx: - The lowest part of the pharynx, connecting it to both the esophagus and larynx. - Associated with vital structures including: - Vocal cords: Located within the larynx (voice box), they play a crucial role in speech production by vibrating as air passes over them. - Esophageal opening: Marks where food enters the esophagus for digestion after passing through the pharynx. Describe the general functions and structure of the larynx. - The larynx is a structure located in the throat that plays a vital role in speech production and protecting the airway during swallowing. - It houses the vocal cords, which are responsible for producing sound. When air passes through the vocal cords, they vibrate, creating various pitches and tones. - The larynx also contains muscles that control the tension and position of the vocal cords, allowing for modulation of pitch and volume. - In addition to its role in speech production, the larynx acts as a valve to prevent food and liquid from entering the airway during swallowing. This is achieved by closure of the epiglottis, a flap-like structure that covers the top of the larynx. - The structure of the larynx consists of cartilage frameworks connected by ligaments and muscles. These components provide stability and flexibility to allow for proper vocal cord movement. Explain how the larynx functions in sound production - The larynx, also known as the voice box, plays a crucial role in sound production. - It contains vocal cords that vibrate when air passes through them, producing sound waves. - The muscles of the larynx adjust the tension and position of the vocal cords, allowing for different pitch and volume levels. - The shape of the larynx and its surrounding structures help to modify the quality and resonance of the produced sound. Describe the structure of the trachea - The trachea, also known as the windpipe, is a tube-like structure that connects the larynx (voice box) to the bronchi of the lungs. - It is composed of a series of C-shaped rings made of hyaline cartilage. There are approximately 16 to 20 tracheal cartilages in total, stacked on top of each other. These rings provide structural support and prevent collapse of the trachea during respiration. - The tracheal rings are incomplete at the posterior side, where they are connected by smooth muscle and connective tissue. This allows flexibility for swallowing and movement of adjacent structures. - The inner lining of the trachea is lined with pseudostratified ciliated columnar epithelium, which contains goblet cells that secrete mucus. The cilia move in coordinated motions to help propel trapped particles and mucus out of the airway. - Surrounding the outer layer of the trachea is adventitia, a fibrous connective tissue that provides additional support and anchorage to surrounding structures. Explain the structure and function of the tracheal cartilages - The tracheal cartilages are C-shaped rings of hyaline cartilage that form the structure of the trachea, also known as the windpipe. - There are approximately 16 to 20 tracheal cartilages in total, stacked on top of each other. - The shape of the tracheal cartilages allows for flexibility and expansion of the trachea during breathing while providing support and preventing collapse. - The open ends of the C-shaped rings face posteriorly towards the esophagus, allowing for its expansion during swallowing. - The gaps between adjacent tracheal cartilages are filled with smooth muscle fibers and connective tissue, forming the trachealis muscle. - The function of the tracheal cartilages is to maintain an open airway by preventing narrowing or collapse of the trachea. This ensures a continuous flow of air into and out of the lungs during respiration. - Additionally, they provide protection to the delicate structures within the respiratory system, such as the bronchi and alveoli, from external compression or trauma. Describe the structural subdivisions of the bronchial tree. - Trachea: The bronchial tree begins with the trachea, a large tube made up of cartilage rings and smooth muscle. It connects the larynx to the main bronchi. - Main Bronchi: The trachea divides into two main bronchi, also known as primary bronchi. There is one main bronchus for each lung. - Lobar Bronchi: Each main bronchus further divides into secondary bronchi, also called lobar bronchi. These supply air to specific lobes of the lungs (three on the right side and two on the left). - Segmental Bronchi: Within each lobe, the lobar bronchi divide into smaller segmental bronchi. These branch off into various segments within the lobes, supplying air to specific regions of the lungs. - Bronchioles: The segmental bronchi continue to divide into smaller tubes called bronchioles. These are less than 1 mm in diameter and lack cartilage rings but have smooth muscle walls. - Terminal Bronchioles: The smallest subdivisions of the bronchial tree are terminal bronchioles. They lead directly to respiratory bronchioles. - Respiratory Bronchioles: Beyond terminal bronchioles, respiratory bronchioles emerge. They contain alveoli sacs where gas exchange occurs between air and blood vessels. Explain the processes of bronchoconstriction and bronchodilation Bronchoconstriction: - Triggered by various stimuli such as allergens, irritants, or exercise. - Smooth muscle surrounding the airways contracts, causing them to narrow. - This narrowing reduces the diameter of the bronchioles and restricts airflow. - Inflammatory mediators, such as histamine and leukotrienes, play a role in initiating bronchoconstriction. - Constriction of bronchial smooth muscles can lead to symptoms like wheezing, coughing, and shortness of breath. - Commonly observed in conditions like asthma and allergic reactions. Bronchodilation: - Mediated by relaxation of smooth muscles surrounding the airways. - Relaxation occurs through activation of beta-adrenergic receptors on the smooth muscle cells. - Stimulation of these receptors triggers the release of cyclic adenosine monophosphate (cAMP), which leads to muscle relaxation. - Additionally, other mediators like nitric oxide also contribute to bronchodilation by relaxing the smooth muscles. - The widening of the bronchioles increases airflow and improves respiratory function. - Medications like beta2 agonists (e.g., albuterol) are commonly used to induce bronchodilation in conditions like asthma. Describe the structure and function of the components of the respiratory zone. - The respiratory zone is the site of actual gas exchange in the lungs. - It consists of the respiratory bronchioles, alveolar ducts, and alveoli. - Respiratory bronchioles are small tubes that branch off from the terminal bronchioles and contain scattered alveoli. - Alveolar ducts are thin-walled passages that connect respiratory bronchioles to the alveoli. - Alveoli are tiny, air-filled sacs surrounded by a dense network of capillaries. - The structure of the alveoli allows for efficient gas exchange between oxygen inhaled into the lungs and carbon dioxide produced as waste by cells. - Oxygen diffuses across the thin walls of the alveoli and into the surrounding capillaries where it binds to hemoglobin in red blood cells. Carbon dioxide, on the other hand, diffuses out of the capillaries into the alveoli to be exhaled. - The large surface area provided by numerous alveoli allows for a high rate of gas exchange to meet the body's oxygen demands. List three types of cells found in alveoli, and describe the function of each. 1. Type I alveolar cells: - Function: These cells are responsible for the main function of gas exchange in the lungs. They are extremely thin and allow for efficient diffusion of oxygen from the air into the bloodstream, as well as removal of carbon dioxide from the blood into the air. 2. Type II alveolar cells (also known as septal or surfactant-producing cells): - Function: These cells produce a substance called surfactant, which helps to reduce surface tension within the alveoli. By reducing surface tension, surfactant prevents the collapse of alveoli during exhalation and facilitates lung expansion during inhalation, promoting effective breathing. 3. Alveolar macrophages (dust cells): - Function: These immune cells reside in the alveoli and play a crucial role in defending the lungs against foreign particles, such as dust, bacteria, and viruses. They engulf and remove these particles through phagocytosis, helping to maintain the overall health and integrity of the respiratory system. Explain the structure of the respiratory membrane. - The respiratory membrane is composed of three layers: the alveolar epithelium, the basement membrane, and the capillary endothelium. - The alveolar epithelium consists of type I and type II alveolar cells. Type I cells are thin and flat, allowing for efficient gas exchange, while type II cells secrete surfactant to reduce surface tension in the alveoli. - The basement membrane is a thin layer of connective tissue that provides support and acts as a barrier between the alveolar epithelium and capillary endothelium. - The capillary endothelium is made up of a single layer of squamous cells, allowing for easy diffusion of gases between the blood and alveoli. - The distance between the air in the alveoli and the blood in the capillaries is extremely small, approximately 0.5 micrometers or less, ensuring rapid gas exchange. - "The respiratory membrane has a large surface area due to numerous alveoli present in the lungs, which enhances efficient gas exchange." (Source: National Heart, Lung, and Blood Institute) - Oxygen diffuses from the alveoli into the capillaries through the respiratory membrane, while carbon dioxide moves in the opposite direction. Describe the location and general structure of the lungs. - The lungs are located in the thoracic cavity, on either side of the heart. - They are enclosed and protected by the rib cage. - Each lung is divided into lobes: the right lung has three lobes (upper, middle, and lower), while the left lung has two lobes (upper and lower). - The surface of each lung is covered by a thin membrane called the visceral pleura. - Within each lung, there are multiple smaller divisions known as bronchopulmonary segments. - The lungs receive oxygenated blood from the pulmonary arteries and exchange gases (oxygen and carbon dioxide) with the surrounding capillaries. Compare and contrast the right versus left lung Right Lung: - Larger and heavier than the left lung - Divided into three lobes: upper, middle, and lower - Contains a larger bronchus compared to the left lung - Accommodates three secondary bronchi, one for each lobe - Has a shorter distance between the right main bronchus and carina (where the trachea divides) - More prone to aspiration due to its more vertical orientation Left Lung: - Smaller and lighter than the right lung - Divided into two lobes: upper and lower - Contains a smaller bronchus compared to the right lung - Accommodates two secondary bronchi, one for each lobe - Has a longer distance between the left main bronchus and carina (where the trachea divides) - Houses the cardiac notch, which allows space for the heart Distinguish between the two types of blood circulation through the lungs 1. Pulmonary circulation: This type of blood circulation refers to the flow of deoxygenated blood from the heart to the lungs and back to the heart again. - Deoxygenated blood is pumped from the right ventricle of the heart through the pulmonary artery. - The pulmonary artery branches into smaller vessels called arterioles, which eventually lead to capillaries in the lungs. - In the capillaries, carbon dioxide (CO2) diffuses out of the blood and oxygen (O2) binds with hemoglobin in red blood cells. - Oxygenated blood then returns to the heart through pulmonary veins. 2. Bronchial circulation: Unlike pulmonary circulation, bronchial circulation involves a separate network of blood vessels that supply oxygen-rich blood to lung tissues themselves. - Bronchial arteries arise from different sources such as systemic circulation or directly from the aorta. - These arteries carry oxygenated blood to nourish lung tissue, including bronchi, bronchioles, and connective tissues. - Some of this oxygenated blood mixes with deoxygenated blood from pulmonary veins before entering lung parenchyma. - Venous blood drains into bronchial veins and ultimately joins systemic venous circulation. Describe the innervation of lung structures by the autonomic nervous system 1. Parasympathetic innervation: The parasympathetic nervous system, specifically the vagus nerve (cranial nerve X), provides extensive innervation to the lungs. 2. Sympathetic innervation: The sympathetic nervous system also plays a role in innervating lung structures. 3. Bronchial smooth muscle: Both sympathetic and parasympathetic divisions of the autonomic nervous system control bronchial smooth muscle tone. 4. Bronchial glands: Parasympathetic nerves stimulate secretion from bronchial glands, contributing to airway lubrication. 5. Pulmonary blood vessels: Both sympathetic and parasympathetic nerves influence vascular tone in pulmonary blood vessels. 6. Pulmonary arteries and veins: Sympathetic nerves regulate the diameter of pulmonary arteries and veins for controlling blood flow. Describe the pleural membranes and pleural cavity - The pleural membranes are thin, double-layered sheets of tissue that line the thoracic cavity and cover the lungs. - They consist of two layers: the visceral pleura and the parietal pleura. - The visceral pleura is the inner layer that directly covers the surface of the lungs. - The parietal pleura is the outer layer that lines the walls of the thoracic cavity. - Between these two layers is a potential space called the pleural cavity. - The pleural cavity contains a small amount of fluid, known as pleural fluid, which acts as a lubricant to reduce friction during breathing movements. - The pleural membranes and cavity play a crucial role in maintaining lung function by creating a mechanical connection between the lungs and chest wall. Explain the function of serous fluid in the pleural cavity. - Serous fluid is a clear, watery fluid that is found in the pleural cavity. - It functions to lubricate and reduce friction between the two layers of the pleura - the visceral pleura (outer layer) and the parietal pleura (inner layer). - The serous fluid allows for smooth movement of the lungs during breathing, preventing them from sticking to the chest wall. - It also helps to maintain the integrity and stability of the pleural cavity by creating a cohesive environment for lung expansion and contraction. - Additionally, serous fluid acts as a protective barrier against infection or inflammation within the pleural space. Explain the anatomic properties that keep lungs inflated 1. Elasticity of lung tissue: The lungs are made up of highly elastic tissue that allows them to expand and contract easily during breathing. This elasticity helps maintain inflation by allowing the lungs to recoil after each breath, preventing collapse. 2. Pleural pressure: The pleura is a thin membrane that covers the surface of the lungs and lines the inside of the chest cavity. It creates a negative pressure between the lung surface and the chest wall, known as pleural pressure, which keeps the lungs inflated by opposing their natural tendency to collapse. 3. Surfactant production: The alveoli (small air sacs in the lungs) are lined with cells called type II pneumocytes, which produce surfactant. Surfactant is a substance that reduces surface tension within the alveoli, preventing them from collapsing and facilitating gas exchange. This property helps keep the lungs inflated. 4. Interdependence between lung tissues: Adjacent alveoli within the lungs share walls, creating an interdependence among them. This interdependence prevents individual alveoli from collapsing by exerting outward forces on each other when one tries to deflate. 5. Intercostal muscles and diaphragm: The intercostal muscles between the ribs and the diaphragm play a crucial role in maintaining lung inflation. During inhalation, these muscles contract, expanding the chest cavity and pulling on the pleura, thus increasing lung volume and promoting inflation. Give an overview of the process of pulmonary ventilation Pulmonary ventilation, also known as breathing, is the process of moving air in and out of the lungs. It involves two main phases: inspiration (inhalation) and expiration (exhalation). - During inspiration: - The diaphragm contracts and flattens, while the intercostal muscles between the ribs contract, expanding the chest cavity vertically and laterally. - This creates a negative pressure within the thoracic cavity, causing air to rush into the lungs through the airways. During expiration: - The diaphragm relaxes, returning to its dome shape, and the intercostal muscles relax. - This reduces the volume of the chest cavity, increasing the pressure inside the lungs. - As a result, air is forced out of the lungs back through the airways and out of the body. - The rate and depth of pulmonary ventilation are regulated by respiratory centers in the brainstem that respond to changes in blood chemistry (such as carbon dioxide levels) and oxygen demand in the body. Explain how pressure gradients are established and result in pulmonary ventilation. - Pressure gradients are established in the respiratory system through changes in the volume of the thoracic cavity and the resulting changes in air pressure. - During inspiration, the diaphragm contracts and moves downward, increasing the volume of the thoracic cavity. This decrease in intra-alveolar pressure creates a pressure gradient between the atmosphere and alveoli. - In response to this pressure gradient, air flows from an area of higher pressure (atmosphere) to an area of lower pressure (alveoli). This is known as inhalation or inspiration. - During expiration, the diaphragm relaxes and moves upward, decreasing the volume of the thoracic cavity. This increase in intra-alveolar pressure establishes a new pressure gradient between the alveoli and atmosphere. - As a result, air flows from an area of higher pressure (alveoli) to an area of lower pressure (atmosphere), causing exhalation or expiration. State the relationship between pressure and volume as described by Boyle's law. Boyle's law states that the pressure of a gas is inversely proportional to its volume, assuming temperature and amount of gas remain constant. - "The volume of a given mass of gas is inversely proportional to its pressure, provided the temperature remains constant." (Boyle's Law) - As the volume of a gas decreases, the pressure exerted by the gas increases proportionally. - Similarly, as the volume of a gas increases, the pressure exerted by the gas decreases proportionally. - This relationship can be expressed mathematically as P₁V₁ = P₂V₂, where P represents pressure and V represents volume. Distinguish between quiet and forced breathing. - Forced breathing requires active engagement of respiratory muscles such as the diaphragm, intercostal muscles, and abdominal muscles to expand the chest cavity more forcefully during inhalation and contract it more vigorously during exhalation. - Quiet breathing is typically quiet and calm, with minimal sound produced during inhalation and exhalation. Describe the anatomic structures involved in regulating breathing - Respiratory Center: The primary regulatory center for breathing is located in the brainstem, specifically in the medulla oblongata and pons. These structures play a crucial role in generating and coordinating the rhythmic pattern of breathing. - Medullary Respiratory Centers: Within the medulla, there are two main groups of neurons involved in regulating breathing. The dorsal respiratory group (DRG) primarily controls inspiration, while the ventral respiratory group (VRG) is responsible for both inspiration and expiration. - Pontine Respiratory Centers: In the pons, there are two additional centers called the pneumotaxic center and apneustic center, which help fine-tune and modulate the rate and depth of respiration generated by the medullary centers. They contribute to smooth transitions between inhalation and exhalation. - Phrenic Nerve: The phrenic nerve originates from the cervical spine (C3-C5) and innervates the diaphragm muscle—the primary muscle responsible for inspiration. Stimulation of this nerve causes contraction of the diaphragm, leading to inhalation. - Intercostal Nerves: Intercostal nerves emerge from each thoracic spinal level and supply motor fibers to the intercostal muscles located between the ribs. These muscles assist with breathing during both inspiration and expiration by expanding or contracting the ribcage. - Diaphragm: the diaphragm is a large dome-shaped muscle that separates the thoracic cavity from the abdominal cavity. Contraction of the diaphragm increases its volume, causing a decrease in intra-thoracic pressure and facilitating inhalation. Explain the physiologic events associated with controlling quiet breathing. Inspiration- initiated when the inspiratory neurons of the VRG within the medullary respiratory center spontaneously depolarize or are "turned on." Expiration- VRG is inhibited or "turned off." 2 seconds of inspiration followed by 3 seconds of expiration Explain the different reflexes that alter breathing rate and depth. 1. Chemoreflexes:Chemoreceptors in the carotid bodies and medulla monitor the levels of carbon dioxide (CO2), oxygen (O2), and pH in the blood, and trigger changes in breathing rate and depth accordingly." 2. Hering-Breuer reflex: Stretch receptors located in the lungs send signals to the brainstem when lung inflation reaches a certain threshold, leading to inhibition of inspiration and prolongation of expiration, thus regulating breathing rate and depth 3. Baroreceptor reflex:Baroreceptors in the walls of major blood vessels detect changes in blood pressure, which can indirectly affect breathing rate by modulating sympathetic and parasympathetic activity. 4. Pain reflexes: Stimulation of pain receptors can elicit a protective response that includes rapid shallow breathing or breath-holding, depending on the nature and location of pain stimuli. 5. Emotional reflexes: Strong emotions such as fear, anger, or excitement can activate neural pathways that alter breathing rate and depth, leading to either hyperventilation or gasping. 6. Exercise-induced reflexes: During physical exertion, signals from motor centers in the brain stimulate increased respiratory drive via both cortical and subcortical pathways, resulting in higher breathing rates and deeper breaths. Distinguish between nervous system control of structures of the respiratory system and nervous system control of structures involved in breathing. Nervous system control of structures of the respiratory system: - The nervous system controls the muscles involved in respiration, such as the diaphragm and intercostal muscles. - These muscles are responsible for expanding and contracting the lungs to facilitate inhalation and exhalation. - Nerves from the brain and spinal cord send signals to these muscles, initiating their contraction or relaxation. Nervous system control of structures involved in breathing: - Breathing is regulated by a specialized area in the brain called the medulla oblongata. - The medulla oblongata receives sensory information from receptors monitoring carbon dioxide levels in the blood. - It then sends nerve impulses to stimulate the appropriate muscles for inhalation or exhalation. - This regulation ensures that oxygen intake matches metabolic needs and maintains proper blood pH levels. Define airflow - Airflow refers to the movement of air in and out of the respiratory system. - It is necessary for the exchange of oxygen and carbon dioxide between the body and the environment. - The process starts with inhalation, where air is drawn into the lungs through the nose or mouth. - The diaphragm contracts and the chest expands, creating a negative pressure that pulls air into the lungs. - During exhalation, the diaphragm relaxes and the chest cavity decreases in size, forcing air out of the lungs. - The airflow is regulated by various structures such as the nasal passages, trachea, bronchi, and bronchioles. - These structures help filter, warm, and moisten incoming air before it reaches the lungs. - Efficient airflow is crucial for proper gas exchange and maintaining respiratory function. Explain how pressure gradients and resistance determine airflow. Airflow increases because a steeper pressure gradient is established between atmospheric pressure and intrapulmonary pressure. Airflow is always opposed by resistance and can be altered in 3 ways: decrease elasticity of chest wall and lungs, change in bronchiole diameter or size of passageway, collapse of alveoli. Distinguish between pulmonary ventilation and alveolar ventilation, and discuss the significance of each. Pulmonary ventilation: - Refers to the total movement of air into and out of the lungs during breathing. - It involves both inhalation and exhalation. - Pulmonary ventilation is measured by tidal volume (the amount of air inspired or expired with each breath) multiplied by respiratory rate (number of breaths per minute). - Significance: Pulmonary ventilation helps in maintaining a constant level of oxygen and carbon dioxide in the alveoli, ensuring efficient gas exchange. Alveolar ventilation: - Refers to the amount of fresh air that reaches the alveoli for gas exchange. - It excludes dead space ventilation, which is the portion of inspired air that does not reach the alveoli. - Alveolar ventilation is calculated by subtracting dead space volume from tidal volume, then multiplying it by respiratory rate. - Significance: Alveolar ventilation plays a crucial role in determining the partial pressure gradients of oxygen and carbon dioxide between the alveoli and capillaries, facilitating effective gas exchange. It helps maintain optimal levels of oxygen for cellular respiration and removes excess carbon dioxide, preventing its accumulation in the body. Explain the relationship between anatomic dead space and physiologic dead space the anatomic dead space- collective space, where there is no exchange of respiratory gases physiologic dead space- The difference in volume of air available for gas exchange, which is the normal anatomic dead space plus any loss of alveoli. The anatomic dead space is equivalent to the physiologic dead space in a healthy individual, because the usual loss of alveoli should be minimal. Define the four different respiratory volume measurements. 1. Tidal volume (TV) is the amount of air inhaled or exhaled per breath during quiet breathing. 2. Inspiratory reserve volume (IRV) is the amount of air that can be forcibly inhaled beyond the tidal volume (after a normal inspiration). IRV is a measure of lung compliance. 3. Expiratory reserve volume (ERV) is the amount that can be forcibly exhaled beyond the tidal volume (after a normal expiration). ERV is a measure of lung and chest wall elasticity. 4. residual volume (RV) is the amount of air left in the lungs even after the most forceful expiration. Explain the four respiratory capacities that are calculated from the volume measurements. 1. Inspiratory capacity (IC) is the sum of the tidal volume plus the inspiratory reserve volume. 2.Functional residual capacity (FRC) is the sum of the expiratory reserve volume plus the residual volume. It is the volume of air that is normally left in the lungs after a quiet expiration. 3. Vital capacity (VC) is the sum of the tidal volume plus both the inspiratory reserve volume and expiratory reserve volume. is significant because it is a measure of the total amount of air that a person can exchange through forced breathing. 4. total lung capacity (TLC) is the sum of all the volumes, including the residual volume, and is the maximum volume of air that the lungs can hold. Give the meaning of forced expiratory volume (FEV) and maximum voluntary ventilation (MMV) Forced expiratory volume (FEV) is the percentage of the vital capacity that can be expelled in a specific period of time. Maximum voluntary ventilation (MVV) is the greatest amount of air that can be taken into, and then expelled from, the lungs in 1 minute. Both the FEV and MVV amounts are timed and reflect rates of air movement Define partial pressure and the movement of gases relative to a partial pressure gradient Partial Pressure is the pressure exerted by each gas within a mixture of gases. nitrogen (78.6%), oxygen (20.9%), carbon dioxide (0.04%), and water vapor (0.46%). Dalton's law, which states that the total pressure in a mixture of gases is equal to the sum of all of the individual partial pressures. Describe the partial pressures that are relevant to gas exchange Partial pressure refers to the pressure exerted by a specific gas in a mixture of gases. - In gas exchange, the relevant partial pressures are those of oxygen (O2) and carbon dioxide (CO2). - The partial pressure of oxygen (PO2) is higher in the alveoli of the lungs compared to that in the capillaries surrounding them. This gradient allows for diffusion of oxygen into the bloodstream. - On the other hand, the partial pressure of carbon dioxide (PCO2) is higher in the capillaries compared to that in the alveoli. This concentration gradient facilitates the diffusion of carbon dioxide out of the bloodstream and into the alveoli for exhalation. - According to Boyle's Law, gases flow from areas of high pressure to areas of low pressure. Therefore, maintaining appropriate partial pressures is crucial for efficient gas exchange. Explain the laws that govern gas solubility. Henry's Law: at a given temp, the solubility of a gas in liquid is dependent upon the partial pressure of the gas in the air and solubility coefficient of the gas in the liquid Describe alveolar gas exchange and the partial pressure gradients responsible. Alveolar gas exchange refers to the process of oxygen and carbon dioxide diffusion between the alveoli in the lungs and the surrounding blood vessels. - Oxygen moves from the alveoli into the bloodstream, while carbon dioxide moves from the bloodstream into the alveoli for elimination. - Partial pressure gradients play a crucial role in driving this exchange. According to Boyle's law, gases move from an area of high partial pressure to an area of low partial pressure. - The partial pressure gradient for oxygen is established by its higher concentration in the alveoli (partial pressure around 100 mmHg) compared to that in the pulmonary capillaries (partial pressure around 40 mmHg). This difference drives oxygen diffusion into the bloodstream. - Similarly, a partial pressure gradient for carbon dioxide is created due to its higher concentration in the pulmonary capillaries (partial pressure around 45 mmHg) compared to that in the alveoli (partial pressure around 40 mmHg). This allows carbon dioxide to diffuse out of the bloodstream and into the alveoli for elimination. Name the two anatomic features of the respiratory membrane that contribute to efficient alveolar gas exchange 1. Thinness: The respiratory membrane is composed of a thin layer of alveolar epithelium, capillary endothelium, and their shared basement membrane. This thinness allows for rapid diffusion of gases across the membrane, minimizing the distance that oxygen and carbon dioxide need to travel between the alveoli and blood vessels. 2. Large Surface Area: The respiratory membrane consists of millions of tiny air sacs called alveoli, which provide a large surface area for gas exchange. This increased surface area maximizes contact between inhaled oxygen and capillary blood, increasing the efficiency of gas exchange by facilitating more rapid diffusion. Explain ventilation-perfusion coupling and how it maximizes alveolar gas exchange Certain physiological adjustments are responsible for increasing gas exchange at the alveoli. Maximum gas exchange is possible when there is contraction and relaxation of the arteries (which carry blood to pulmonary capillaries) and smooth muscle of the bronchiole. This innate ability of the arteries to control blood flow and the ability of the bronchioles to control airflow simultaneously known as ventilation-perfusion coupling. Explain the partial pressure gradients between systemic cells and the blood in capillaries - Oxygen: The partial pressure of oxygen (PO2) is higher in the systemic cells compared to the blood capillaries. This creates a gradient that drives oxygen from the cells into the capillaries. - Carbon dioxide: The partial pressure of carbon dioxide (PCO2) is lower in the systemic cells than in the blood capillaries. This concentration gradient allows carbon dioxide to diffuse out of the cells and into the capillaries. - Nutrients: Various nutrients such as glucose, amino acids, and fatty acids have higher concentrations within systemic cells compared to the blood capillaries. This concentration difference leads to their movement from cells into capillaries. - Waste products: Metabolic waste products like urea and lactic acid have higher concentrations in systemic cells compared to the blood capillaries. Consequently, these waste products move from cells into the capillaries due to their concentration gradient. Explain why hemoglobin is essential to oxygen transport Hemoglobin is a protein found in red blood cells that binds to oxygen molecules. - It has a high affinity for oxygen, allowing it to efficiently transport and deliver oxygen throughout the body. - Hemoglobin can bind up to four oxygen molecules at a time, increasing its capacity for oxygen transport. - The binding of oxygen to hemoglobin occurs in the lungs, where oxygen is taken up from the air we breathe. - Once bound, hemoglobin carries the oxygen-rich blood from the lungs to tissues and organs via the circulatory system. - In tissues with low oxygen levels, such as muscles during exercise or organs like the brain, hemoglobin releases its bound oxygen molecules. - This release of oxygen allows tissues and organs to utilize the vital gas for energy production and cellular respiration. - Without hemoglobin, efficient delivery of oxygen to tissues would be compromised, leading to hypoxia (oxygen deprivation) and potentially severe health consequences. Describe the three ways carbon dioxide is transported in the blood. 1. Dissolved form: Carbon dioxide can dissolve directly into the blood plasma as a gas, forming carbonic acid (H2CO3). 2. Carbamino compounds: Carbon dioxide can combine with the globin part of hemoglobin to form carbamino compounds. 3. Bicarbonate ions: The majority of carbon dioxide is transported in the blood as bicarbonate ions (HCO3-) through a series of reactions known as the bicarbonate buffer system Explain the conversion of CO₂ to and from HCO₃⁻ within erythrocytes 1. In erythrocytes, carbon dioxide (CO₂) is converted to bicarbonate ions (HCO₃⁻) through the enzyme carbonic anhydrase. 2. Carbonic anhydrase facilitates the rapid conversion of CO₂ and water (H₂O) into carbonic acid (H₂CO₃). This reaction occurs in the red blood cells. 3. The carbonic acid (H₂CO₃) formed by carbonic anhydrase rapidly dissociates into a hydrogen ion (H+) and a bicarbonate ion (HCO₃⁻). 4. The released H+ ions bind to hemoglobin within the erythrocyte, which helps maintain the pH balance by preventing excessive buildup of acidity. 5. Simultaneously, chloride ions (Cl⁻) from plasma enter the erythrocytes to replace the lost negative charge due to HCO₃⁻ formation. This process is known as chloride shift or Hamburger phenomenon. Name the three substances carried by hemoglobin 1. Oxygen: Hemoglobin binds to oxygen in the lungs and transports it throughout the body, delivering it to tissues and organs for cellular respiration. This process is crucial for sustaining life. "Hemoglobin carries oxygen from the lungs to the tissues." (American Society of Hematology) 2. Carbon dioxide: Hemoglobin also carries carbon dioxide, a waste product of cellular metabolism, away from tissues and back to the lungs for exhalation. This helps maintain proper pH balance in the body. "Carbon dioxide produced by cells diffuses into capillaries and binds to hemoglobin, which then carries it back to the lungs." (MedlinePlus) 3. Nitric oxide: In addition to oxygen and carbon dioxide, hemoglobin can carry nitric oxide molecules. Nitric oxide plays a vital role in regulating blood vessel function, promoting vasodilation and maintaining blood pressure within normal ranges. Hemoglobin acts as a reservoir of nitric oxide that can be released when needed to regulate vascular tone. Explain the significance of the oxygen-hemoglobin saturation curve for both alveolar and systemic gas exchange The oxygen-hemoglobin saturation curve refers to the amount of oxygen bound to hemoglobin. the higher the partial pressure of oxygen, the higher the hemoglobin saturation Explain how hyperventilation and hypoventilation influence the chemical composition of blood Hyper: Breathing rate or depth increased above body's demand-- due to panic or ascending to high altitude. PO2 levels increase and partial pressure gradients between alveoli and blood increases for both gases. 1) Additional oxygen does not enter blood because hemoglobin is generally 98% saturated 2) Additional CO2 leaving blood to enter alveoli, therefore blood PCO2 decreases below normal = hypocapnia. Causing vasoconstriction and decrease oxygen delivery to the brain. May also decrease H+ in blood. Symptoms: faint, numb, tingling, cramps and severe cases = death. Hypo: Breathing slow (Bradypnea) or shallow (hypopnea). Can be caused by airway obstruction, pneumonia, brainstem injury, obesity, or any interference with pulmonary ventilation or alveolar gas exchange. Oxygen levels decrease and carbon dioxide levels increase in alveoli. Lower amounts of oxygen diffuse from alveoli into blood and blood PO2 decreases (hypoxemia). Lower amounts of CO2 diffuse from blood into alveoli, blood PCO2 increases (Hypercapnia) and may decrease pH (acidosis). Symptoms: lethargy, headache, polycythemia, and cyanotic tissues, severe cases cause death) Describe how breathing rate and depth affect venous return of blood and lymph The rate and depth of breathing influences the blood levels of O2 and CO2, and H+ and venous return of blood and lymph due to changes in compression within the thoracic cavity that occur during breathing. Explain the changes in breathing that accompany exercise - Increased breathing rate: During exercise, the breathing rate increases significantly to meet the increased oxygen demand of the muscles. This allows for a greater intake of oxygen and removal of carbon dioxide from the body. - Deepened breaths: With exercise, the depth of each breath also tends to increase. This is because deeper breaths allow for a larger volume of air to be inhaled and exhaled, facilitating efficient gas exchange in the lungs. - More use of diaphragm: During exercise, there is an increased reliance on the diaphragm for breathing. The diaphragm, which is a dome-shaped muscle located below the lungs, contracts more forcefully during exercise to help draw in more air into the lungs. - Increased tidal volume: Tidal volume refers to the amount of air that is inspired or expired with each breath. During exercise, there is often an increase in tidal volume as a result of deeper and more forceful breaths. This enables a higher intake of oxygen and removal of carbon dioxide. - Enhanced efficiency of respiratory muscles: Regular exercise can lead to improved strength and endurance of respiratory muscles such as the diaphragm and intercostal muscles. This allows for better control over breathing patterns during physical activity.