Structure and Function of DNA PDF
Document Details
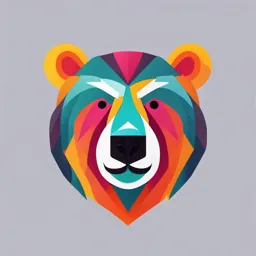
Uploaded by SharperSet3975
Islamia University of Bahawalpur
Eric J. Simon, Jean L. Dickey
Tags
Summary
This document is about the structure and function of DNA. It discusses the molecular basis of inheritance, and covers the molecular components as well as the function of DNA. It gives a summary on the processes involved in molecular biology.
Full Transcript
10 The Structure and Function of DNA Why Molecular Biology Matters A single molecular “typo” in DNA can result in a life- threatening disease....
10 The Structure and Function of DNA Why Molecular Biology Matters A single molecular “typo” in DNA can result in a life- threatening disease. Because all life on Earth shares a universal genetic code, your DNA could be used to genetically modify a monkey. Mad cow ▶ disease is caused by an abnormal molecule of protein. ◀ Enzymes help maintain the integrity of your DNA to greater than 99.999% accuracy. 204 CHAPTER CONTENTS CHAPTER THREAD DNA: Structure and Replication 206 The Deadliest Virus Information Flow: From DNA to RNA to Protein 210 BIOLOGY AND SOCIETY The First 21st-Century Pandemic 205 Viruses and Other Noncellular Infectious Agents 220 THE PROCESS OF SCIENCE Do Flu Vaccines Protect the Elderly? 224 EVOLUTION CONNECTION Emerging Viruses 226 The Deadliest Virus BIOLOGY AND SOCIETY The First 21st-Century Pandemic In 2009, a cluster of unusual flu cases was reported in and around Mexico City. Despite a near total shutdown of the city, the new flu virus, called 2009 H1N1, quickly spread to California and Texas. This strain was originally misnamed the “swine flu”; in fact, pigs had little impact on the spread of this virus, which, like most flu viruses, is passed from person to person through respiratory fluids. Nonetheless, the new strain received heavy media attention and caused considerable worry among the general public. In June 2009, the World Health Organization (WHO) declared H1N1 to be the first influenza pandemic (global epidemic) of the 21st century; the last flu pandemic was in 1968. In response, the WHO unveiled a comprehensive effort to contain H1N1, including increased surveillance, development of rapid testing procedures, the issuance of travel warnings, recommendations for increased sanitation (hand wash- ing, etc.), and the production, stockpiling, and distribution of antiviral drugs. By 2010, cases of H1N1 had been confirmed in 214 countries. Scientists soon determined that H1N1 was a hybrid flu strain, cre- ated when a previously known flu virus (which itself originated through a combination of viruses from birds, swine, and people) mixed with The H1N1 influenza virus. Citizens of Russia try to pro- an Asian swine flu virus. This novel combination of genes produced tect themselves from H1N1 infection in late fall 2009. some unusual features in the H1N1 strain. Most significantly, it infected young, healthy people, whereas the flu usually affects the elderly or people who are already sick. Many countries participated in the WHO-coordinated response, including widespread distribution of a new vaccine. As a result, the virus was contained, and WHO declared the pandemic over in August 2010. The WHO confirmed that this virus killed about 18,000 people, and estimates of the total number of unreported deaths topped 250,000. You may wonder, What’s the big deal about the flu? The flu is not merely a seasonal inconvenience. In fact, the influenza virus may be the deadliest pathogen known to science. In a typical year in the United States, more than 20,000 people die from influenza infection. And that is considered a good year. Once ev- ery few decades, a new flu strain explodes on the scene, causing pandemics and widespread death. H1N1 pales in comparison to the deadliest outbreak: the pandemic of 1918–1919, which in just 18 months killed 40 million people worldwide—that’s more people than have died of AIDS since it was discovered more than 30 years ago. Given the nature of the threat, it’s no surprise that health workers remain vigilant, always aware that a new and deadly flu virus may appear at any time. The flu virus, like all viruses, consists of a relatively simple structure of nucleic acid (RNA in this case) and protein. Combating any virus requires a detailed understanding of life at the molecular level. In this chapter, we will explore the structure of DNA, how it replicates and mutates, and how it controls the cell by directing the synthesis of RNA and protein. 205 DNA: Structure and Replication CHAPTER 10 THE STRUCTURE AND FUNCTION OF DNA DNA was known to be a chemical component of cells generation to generation. The race was on to discover the by the late 1800s, but Gregor Mendel and other early ge- link between the structure and function of this important neticists did their work without any knowledge of DNA’s molecule. We will describe that momentous discovery role in heredity. By the late 1930s, experimental studies shortly. First, let’s review the underlying chemical struc- had convinced most biologists that one specific kind of ture of DNA and its chemical cousin RNA. molecule, rather than a complex chemical mixture, is the basis of inheritance. Attention focused on chromo- somes, which were already known to carry genes. By DNA and RNA Structure the 1940s, scientists knew that chromosomes consist of Both DNA and RNA are nucleic acids, which consist of two types of chemicals: DNA and protein. And by the long chains (polymers) of chemical units (monomers) early 1950s, a series of discoveries had convinced the called nucleotides. (For an in-depth refresher, see scientific world that DNA was the molecule that acts Figures 3.21–3.25.) A diagram of a nucleotide polymer, as the hereditary material. This breakthrough ushered or polynucleotide, is shown in Figure 10.1. Polynucleo- in the field of molecular biology, the study of heredity tides can be very long and may have any sequence of at the molecular level. the four different types of nucleotides (abbreviated A, What came next was one of the most celebrated quests C, T, and G), so a tremendous variety of polynucleotide in the history of science: the effort to figure out the chains is possible. structure of DNA. A good deal was already known about Nucleotides are joined together by covalent bonds DNA. Scientists had identified all its atoms and knew between the sugar of one nucleotide and the phos- how they were bonded to one another. What was not phate of the next. This results in a repeating pattern of understood was the specific three-dimensional ar- sugar-phosphate-sugar-phosphate, which is known as a rangement of atoms that gives DNA its unique sugar-phosphate backbone. The nitrogenous bases are properties—the capacity to store genetic arranged like ribs that project from this backbone. You A T information, copy it, and pass it from can think of a polynucleotide as a long ladder split in C G T A Phosphate group Nitrogenous base C G T A A A G C Sugar G A T G C C C O T A DNA nucleotide T A C H H3C C N O C G C C T A T O P O CH2 H N O T Thymine (T) DNA double helix O– O Phosphate C H H C group H C C H G G ▶ Figure 10.1 The O H chemical structure of a Sugar DNA polynucleotide. A (deoxyribose) molecule of DNA contains two polynucleotides, each a G DNA nucleotide G chain of nucleotides. Each nucleotide consists of a nitrogenous base, a sugar (blue), and a phosphate group (gold). Polynucleotide Sugar-phosphate backbone 206 half longwise, with rungs that come in four colors. The The bases can be divided into two types. Thymine (T) DNA: STRUCTURE AND REPLICATION sugars and phosphates make up the side of the ladder, and cytosine (C) are single-ring structures. Adenine (A) with the sugars acting as the half-rungs. and guanine (G) are larger, double-ring structures. In- Moving from left to right across Figure 10.1, we can stead of thymine, RNA has a similar base called zoom in to see that each nucleotide consists of three uracil (U). And RNA con- components: a nitrogenous base, a sugar (blue), and a tains a slightly different sugar Cytosine phosphate group (gold). Examining a single nucleotide than DNA (ribose instead of even more closely, we see the chemical structure of its deoxyribose, accounting for the Uracil three components. The phosphate group, with a phos- names RNA vs. DNA). Other than Adenine phorus atom (P) at its center, is the source of the acid in that, RNA and DNA polynucle- nucleic acid. Each phosphate has a negative charge on otides have the same chemi- Guanine one of its oxygen atoms. The sugar has five carbon atoms cal structure. Figure 10.2 is (shown in red): four in its ring and one extending above a computer graphic of a piece the ring. The ring also includes an oxygen atom. The of RNA polynucleotide sugar is called deoxyribose because, compared with the about 20 nucleo- CHECKPOINT sugar ribose, it is missing an oxygen atom. The full name tides long. Compare and contrast the for DNA is deoxyribonucleic acid, with nucleic referring Phosphate chemical components of to DNA’s location in the nuclei of eukaryotic cells. The DNA and RNA. nitrogenous base (thymine, in our example) has a ring C, but DNA has T and RNA has U. Sugar of nitrogen and carbon atoms with various chemical and DNA have the bases A, G, and ▶ Figure 10.2 An RNA (ribose) in DNA, it is deoxyribose. Both RNA groups attached. Nitrogenous bases are basic (having a polynucleotide. The yellow group). In RNA, the sugar is ribose; used for the phosphorus atoms high pH, the opposite of acidic), hence their name. nitrogenous base + a phosphate and the blue of the sugar of nucleotides (a sugar + a The four nucleotides found in DNA differ only in atoms make it easy to spot the Answer: Both are polymers their nitrogenous bases (see Figure 3.23 for a review). sugar-phosphate backbone. Watson and Crick’s Discovery of the Double Helix The celebrated partnership that solved the puzzle of Figure 10.3 Discoverers of the double helix. DNA structure began soon after a 23-year-old newly minted American Ph.D. named James D. Watson jour- neyed to Cambridge University in England. There, a more senior scientist, Francis Crick, was studying pro- tein structure with a technique called X-ray crystallogra- phy. While visiting the laboratory of Maurice Wilkins at King’s College in London, Watson saw an X-ray image of DNA produced by Wilkins’s colleague Rosalind Frank- lin. The data produced by Franklin turned out to be the key to the puzzle. A careful study of the image enabled Watson to figure out that the basic shape of DNA is a helix (spiral) with a uniform diameter. The thickness of the helix suggested that it was made up of two poly- nucleotide strands—in other words, a double helix. But how were the nucleotides arranged in the double helix? Using wire models, Watson and Crick began trying to construct a double helix that conformed to all known data about DNA (Figure 10.3). Watson placed the back- James Watson (left) and Francis Crick. The discoverers of Rosalind Franklin Using X-rays, Franklin bones on the outside of the model, forcing the nitrog- the structure of DNA are shown in 1953 with their model of generated some of the key data that provided enous bases to swivel to the interior of the molecule. the double helix. insight into the structure of DNA. As he did this, it occurred to him that the four kinds of bases must pair in a specific way. This idea of specific base pairing was a flash of inspiration that enabled Watson and Crick to solve the DNA puzzle. 207 CHAPTER 10 At first, Watson imagined that the bases paired like Each base has protruding chemical groups that can best THE STRUCTURE AND FUNCTION OF DNA with like—for example, A with A, C with C. But that kind form hydrogen bonds with just one appropriate partner. of pairing did not fit with the fact that the DNA mol- This would be like having four colors of snap-together ecule has a uniform diameter. An AA pair (made of two puzzle pieces and realizing that only certain colors can double-ringed bases) would be almost twice as wide as a snap together (e.g., red can only snap together with blue). CC pair (made of two single-ringed bases), resulting in an Similarly, adenine can best form hydrogen bonds with uneven molecule. It soon became apparent that a double- thymine, and guanine with cytosine. In the biologist’s ringed base on one strand must always be paired with a shorthand: A pairs with T, and G pairs with C. A is also single-ringed base on the opposite strand. Moreover, Wat- said to be “complementary” to T, and G to C. son and Crick realized that the chemical structure of each If you imagine a polynucleotide strand as a half ladder, kind of base dictated the pairings even more specifically. then you can picture the model of the DNA double helix ▶ Figure 10.4 A proposed by Watson and Crick as a full ladder twisted into rope-ladder model a spiral (Figure 10.4). Figure 10.5 shows three more de- of a double helix. tailed representations of the double helix. The ribbonlike The ropes at the sides represent the sugar- diagram in Figure 10.5a symbolizes the bases with shapes phosphate backbones. that emphasize their complementarity. Figure 10.5b is a Each rung stands more chemically precise version showing only four base for a pair of bases pairs, with the helix untwisted and the individual hydro- connected by hydrogen bonds. gen bonds specified by dashed lines. Figure 10.5c is a computer model showing part of a double helix in detail. Although the base-pairing rules dictate the side-by- side combinations of bases that form the rungs of the double helix, they place no restrictions on the sequence of nucleotides along the length of a DNA strand. In fact, the sequence of bases can vary in countless ways. Twist In 1953, Watson and Crick rocked the scientific world Figure 10.5 Three representations of DNA. with a succinct paper proposing their molecular model for Hydrogen bond O OH A T P T OH – O O C G A H2 C O O CH2 T A O O– P O O O O P C C G O– O G H2 C O T A O CH2 O O– G C P O O O O G P T O– O A H2C O CH2 O A T O O– G C P O O O O T A C P C O– O G G H2C O O CH2 O O– C G OH P OH O T A (a) Ribbon model. The sugar-phosphate (b) Atomic model. In this more chemically detailed structure, you (c) Computer model. Each atom is shown backbones are blue ribbons, and the bases can see the individual hydrogen bonds (dashed lines). You can also as a sphere, creating a space-filling model. are complementary shapes in shades of see that the strands run in opposite directions: Notice that the sugars green and orange. on the two strands are upside down with respect to each other. 208 DNA. Few milestones in the history of biology base-pairs with its complement on the tem- DNA: STRUCTURE AND Enzymes help REPLICATION have had as broad an impact as their double plate strand, a DNA polymerase adds it helix, with its A-T and C-G base pairing. In maintain the to the end of the growing daughter 1962, Watson, Crick, and Wilkins received integrity of your strand. The process is both fast the Nobel Prize for their work. (Franklin DNA to greater (a rate of 50 nucleotides per sec- than 99.999% T A Parental (old) deserved a share of the prize, but she had ond is typical) and amazingly accuracy. C G DNA molecule died from cancer in 1958, and Nobel prizes are accurate, with fewer than one in A T never granted posthumously.) a billion bases incorrectly paired. In A T In their 1953 paper, Watson and Crick wrote addition to their roles in DNA replication, that the structure they proposed “immediately suggests DNA polymerases and some of the associated G C a possible copying mechanism for the genetic material.” proteins can repair DNA that has been dam- A T In other words, the structure of DNA points toward a aged by toxic chemicals or high-energy ra- A T molecular explanation for life’s unique properties of re- diation, such as X-rays and ultraviolet light. T A production and inheritance. Understanding how the ar- DNA replication begins on a double Daughter C G rangement of parts within DNA affects it actions within a helix at specific sites, called origins (new) strand C G T cell is an excellent example of biology’s important theme of replication. Replication then A of the relationship of structure to function, as we see next. proceeds in both directions, C C Parental G G creating what are called (old) strand G C T Structure/ G replication “bubbles” Function DNA Replication A T C C T A (Figure 10.7). The parental G G A A T Every cell contains a DNA “cookbook” that provides DNA strands open C C A G complete information on how to make and maintain up as daughter T T A that cell. When a cell reproduces, it must duplicate this strands elongate A T T A G information, providing one copy to the new offspring on both sides of Daughter DNA A C molecules T cell while keeping one copy for itself. Thus, each cell each bubble. The DNA (double helices) must have a means of copying the DNA instructions. In molecule of a typical a clear demonstration of how the structure of a biologi- eukaryotic chromosome cal system can provide insight into its function, Watson has many origins where replication can start simultane- Figure 10.6 DNA replication. Replication and Crick’s model of DNA suggests that each DNA ously, shortening the total time needed for the process. results in two daughter DNA strand serves as a mold, or template, to guide reproduc- Eventually, all the bubbles merge, yielding two completed molecules, each consisting tion of the other strand. If you know the sequence of double-stranded daughter DNA molecules. of one old strand and one bases in one strand of the double helix, you can very DNA replication ensures that all the body cells in a new strand. The parental DNA untwists as its strands separate, easily determine the sequence of bases in the other multicellular organism carry the same genetic informa- and the daughter DNA rewinds strand by applying the base-pairing rules: A pairs with T tion. It is also the means by which genetic information is as it forms. (and T with A), and G pairs with C (and C with G). For passed along to offspring. example, if one polynucleotide has the sequence AGTC, then the complementary polynucleotide in that DNA Figure 10.7 Multiple “bubbles” in replicating DNA. molecule must have the sequence TCAG. Origin of Origin of Figure 10.6 shows how this model can account for the replication replication direct copying of a piece of DNA. The two strands of pa- Parental strands rental DNA separate, and each becomes a template for the CHECKPOINT assembly of a complementary strand from a supply of free 1. How does nucleotides. The nucleotides are lined up one at a time Origin of complementary base replication pairing make DNA along the template strand in accordance with the base- Parental strand replication possible? pairing rules. Enzymes link the nucleotides to form new Daughter strand 2. What enzymes connect DNA strands. The completed new molecules, identical to nucleotides together the parental molecule, are known as daughter DNA mol- during DNA replication? ecules (no gender should be inferred from this name). polymerases The process of DNA replication requires the co- complementary strands. 2. DNA Bubble specific base pairing into new operation of more than a dozen enzymes and other nucleotides can be arranged by proteins. The enzymes that make the covalent bonds serves as a template on which of the double helix separate, each between the nucleotides of a new DNA strand are Two daughter DNA molecules Answers: 1. When the two strands called DNA polymerases. As an incoming nucleotide 209 CHAPTER 10 THE STRUCTURE AND FUNCTION OF DNA Information Flow From DNA to RNA to Protein Now that we’ve seen how the structure of DNA allows chemical reactions that are necessary for life. it to be copied, let’s explore how DNA provides instruc- DNA specifies the synthesis of proteins. However, a tions to a cell and to an organism as a whole. gene does not build a protein directly. Instead, DNA dis- patches instructions in the form of RNA, which in turn programs protein synthesis. This fundamental concept How an Organism’s Genotype in biology is summarized in Figure 10.8. The molecular “chain of command” is from DNA in the nucleus (purple Determines Its Phenotype area in the figure) to RNA to protein synthesis in the We can now define genotype and phenotype with cytoplasm (blue area). The two stages are transcription, CHECKPOINT regard to the structure and function of DNA. An the transfer of genetic information from DNA into an What are transcription organism’s genotype, its genetic makeup, is the heritable RNA molecule, and translation, the transfer of the and translation? the synthesis of a polypeptide. information contained in the sequence of nucleotide information from RNA into a polypeptide (protein information in an RNA molecule for bases in its DNA. The phenotype, the organism’s physical strand). The relationship between genes and proteins is traits, arises from the actions of a wide variety of thus one of information flow: The function of a DNA RNA. Translation is the use of the of genetic information from DNA to Answer: Transcription is the transfer proteins. For example, structural proteins help make up gene is to dictate the production of a polypeptide. the body of an organism, and enzymes catalyze the Figure 10.8 The flow of genetic information in a eukaryotic cell. A sequence of nucleotides in the DNA is transcribed into a molecule of RNA in the cell’s nucleus. The RNA travels to the cytoplasm, where it is translated into the specific amino acid sequence of a protein. DNA TRANSCRIPTION Nucleus RNA Cytoplasm TRANSLATION Protein 210 From Nucleotides to Amino a polypeptide? Keep in mind that there are only four different kinds of nucleotides in DNA (A, G, C, T) and FROM DNA TO RNA TO PROTEIN Acids: An Overview RNA (A, G, C, U). During translation, these four must somehow specify 20 amino acids. If each nucleotide base Genetic information in DNA is transcribed into RNA coded for one amino acid, only 4 of the 20 amino acids and then translated into polypeptides, which then fold could be accounted for. In fact, triplets of bases are the into proteins. But how do these processes occur? Tran- smallest “words” of uniform length that can specify all scription and translation are linguistic terms, and it is the amino acids. There can be 64 (that is, 43) possible useful to think of nucleic acids and proteins as having code words of this type—more than enough to specify languages. To understand how genetic information the 20 amino acids. Indeed, there are enough triplets to passes from genotype to phenotype, we need to see how allow more than one coding for each amino acid. For the chemical language of DNA is translated into the dif- example, the base triplets AAA and AAG both code for ferent chemical language of proteins. the same amino acid. What exactly is the language of nucleic acids? Both Experiments have verified that the flow of informa- DNA and RNA are polymers made of nucleotide mono- tion from gene to protein is based on a triplet code. mers strung together in specific sequences that convey The genetic instructions for the amino acid sequence information, much as specific sequences of letters con- of a polypeptide chain are written in DNA and RNA as vey information in English. In DNA, the monomers a series of three-base words called codons. Three-base are the four types of nucleotides, which differ in their codons in the DNA are transcribed into complemen- CHECKPOINT nitrogenous bases (A, T, C, and G). The same is true for tary three-base codons in the RNA, and then the RNA RNA, although it has the base U instead of T. codons are translated into amino acids that form a How many nucleotides are necessary to code The language of DNA is written as a linear sequence polypeptide. As summarized in Figure 10.9, one DNA for a polypeptide that is of nucleotide bases, such as the blue sequence you see codon (three nucleotides) → one RNA codon (three 100 amino acids long? on the enlarged DNA strand in Figure 10.9. Every gene nucleotides) → one amino acid. Next we turn to the Answer: 300 is made up of a specific sequence of bases, with special codons themselves. sequences marking the beginning and the end. A typi- cal gene is a few thousand nucleotides in length. Figure 10.9 Transcription of DNA and translation When a segment of DNA is tran- of codons. This figure focuses on a small region of one of the scribed, the result is an RNA molecule. The genes carried by a DNA molecule. The enlarged segment from process is called transcription because the one strand of gene 3 shows its specific sequence of bases. nucleic acid language of DNA has simply been The red strand and purple chain represent the results of transcription and translation, respectively. rewritten (transcribed) as a sequence of bases of Gene 1 RNA; the language is still that of nucleic acids. The nucleotide bases of the RNA molecule are comple- mentary to those on the DNA strand. As you will soon Gene 2 see, this is because the RNA was synthesized using the DNA molecule DNA as a template. Translation is the conversion of the nucleic acid lan- Gene 3 guage to the polypeptide language. Like nucleic acids, polypeptides are straight polymers, but the monomers that make them up—the letters of the polypep- DNA strand tide alphabet—are the 20 amino acids common A A A C C G G C A A A A to all organisms (represented as purple shapes in TRANSCRIPTION Figure 10.9). The sequence of nucleotides of the RNA molecule dictates the sequence of amino ac- U U U G G C C G U U U U ids of the polypeptide. But remember, RNA is only RNA a messenger; the genetic information that dictates the amino acid sequence originates in DNA. TRANSLATION Codon What are the rules for translating the RNA message into a polypeptide? In other words, Polypeptide what is the correspondence between the nucleo- tides of an RNA molecule and the amino acids of Amino acid 211 CHAPTER 10 THE STRUCTURE AND The Genetic Code Figure 10.11 A pig expressing a foreign gene. The glowing porker in the middle was created when researchers incorporated a jelly (jellyfish) gene for a FUNCTION OF DNA protein called green fluorescent protein (GFP) into the DNA of a standard pig. The genetic code is the set of rules that convert a nucleotide sequence in RNA to an amino acid sequence. As Figure 10.10 shows, 61 of the Because all life on Earth 64 triplets code for amino acids. shares a universal genetic The triplet AUG has a dual code, your DNA could be used to genetically function: It codes for the amino modify a monkey. acid methionine (abbreviated Met) and can also provide a signal for the start of a polypeptide chain. Three codons (UAA, UAG, and UGA) do not designate amino acids. They are the stop codons that instruct the ribosomes to end the polypeptide. Notice in Figure 10.10 that a given RNA triplet always specifies a given amino acid. For example, although codons UUU and UUC both specify phenylalanine (Phe), neither of them ever represents any other amino acid. The codons in occur in a linear order along the DNA and RNA, with the figure are the triplets found in RNA. They have a no gaps separating the codons. straightforward, complementary relationship to the The genetic code is nearly universal, shared by codons in DNA. The nucleotides making up the codons organisms from the simplest bacteria to the most complex plants and animals. Second base of RNA codon The universality of the genetic U C A G vocabulary suggests that it UUU UCU UAU UGU U Phenylalanine Tyrosine Cysteine arose very early in evolution UUC (Phe) UCC UAC (Tyr) UGC (Cys) C and was passed on over the U Serine UUA Leucine UCA (Ser) UAA Stop UGA Stop A eons to all the organisms liv- UUG (Leu) UCG UAG Stop UGG Tryptophan (Trp) G ing on Earth today. In fact, such universality is the key to CUU CCU CAU CGU U Histidine modern DNA technologies. CUC CCC CAC (His) CGC C Because diverse organisms Third base of RNA codon First base of RNA codon C Leucine Proline Arginine CUA (Leu) CCA (Pro) CAA Glutamine CGA (Arg) A share a common genetic code, CUG CCG CAG (Gln) CGG G it is possible to program one species to produce a protein AUU ACU AAU AGU U Asparagine Serine from another species by trans- AUC Isoleucine ACC AAC (Asn) AGC (Ser) C A (Ile) Threonine planting DNA (Figure 10.11). AUA ACA (Thr) AAA Lysine AGA Arginine A This allows scientists to mix AUG Met or start ACG AAG (Lys) AGG (Arg) G and match genes from various species—a procedure with GUU GCU GAU GGU U Aspartic many useful genetic engineer- CHECKPOINT GUC GCC GAC acid (Asp) GGC C G Valine Alanine Glycine ing applications in agriculture, GUA (Val) GCA (Ala) GAA GGA (Gly) A medicine, and research (see An RNA molecule contains Glutamic the nucleotide sequence GUG GCG GAG acid (Glu) GGG G Chapter 12 for further discus- CCAUUUACG. Using sion of genetic engineering). Figure 10.10, translate Figure 10.10 The dictionary of the genetic code, listed by RNA codons. Practice using Besides having practical this sequence into the this dictionary by finding the codon UGG. (It is the only codon for the amino acid tryptophan, corresponding amino acid purposes, a shared genetic Trp.) Notice that the codon AUG (highlighted in green) not only stands for the amino acid sequence. methionine (Met), but also functions as a signal to “start” translating the RNA at that place. vocabulary also reminds us of Answer: Pro-Phe-Thr Three of the 64 codons (highlighted in red) function as “stop” signals that mark the end of a the evolutionary kinship that genetic message, but do not encode any amino acids. connects all life on Earth. 212 Transcription: From RNA polymerase to the promoter and the start of RNA synthesis. For any gene, the promoter dictates which of FROM DNA TO RNA TO PROTEIN DNA to RNA the two DNA strands is to be transcribed (the particular strand varies from gene to gene). Let’s look more closely at transcrip- tion, the transfer of genetic informa- RNA Elongation tion from DNA to RNA. If you think During the second phase of transcription, elongation, of your DNA as a cookbook, then the RNA grows longer. As RNA synthesis continues, the transcription is the process of copy- RNA strand peels away from its DNA template, allowing ing one recipe onto an index card (a the two separated DNA strands to come back together in molecule of RNA) for immediate use. Figure 10.12a is a the region already transcribed. close-up view of this process. As with DNA replication, the two DNA strands must first separate at the place Termination of Transcription where the process will start. In transcription, however, In the third phase, termination, the RNA polymerase only one of the DNA strands serves as a template for reaches a special sequence of bases in the DNA template the newly forming RNA molecule; the other strand is called a terminator. This sequence signals the end of the unused. The nucleotides that make up the new RNA gene. At this point, the polymerase molecule detaches CHECKPOINT molecule take their place one at a time along the DNA from the RNA molecule and the gene, and the DNA How does RNA polymerase template strand by forming hydrogen bonds with the strands rejoin. “know” where to start nucleotide bases there. Notice that the RNA nucleotides In addition to producing RNA that encodes amino transcribing a gene? follow the usual base-pairing rules, except that U, rather acid sequences, transcription makes two other kinds of sequence. than T, pairs with A. The RNA nucleotides are linked by promoter, a specific nucleotide RNA that are involved in building polypeptides. We dis- Answer: It recognizes the gene’s the transcription enzyme RNA polymerase. cuss these kinds of RNA a little later. Figure 10.12b is an overview of the transcription of an entire gene. Special sequences of DNA nucleotides RNA polymerase tell the RNA polymerase where to start and where to stop the transcribing process. DNA of gene Initiation of Transcription The “start transcribing” signal is a nucleotide sequence Promoter DNA called a promoter, which is located in the DNA at the 1 Initiation Terminator DNA beginning of the gene. A promoter is a specific place where RNA polymerase attaches. The first phase of transcription, called initiation, is the attachment of RNA 2 Elongation Figure 10.12 Transcription. RNA nucleotides RNA polymerase A T C C A A T U 3 Termination C T Growing T RNA G U G A A U C C A C C A A T A G G T T Newly made Completed RNA RNA Direction of transcription Template strand of DNA RNA polymerase (a) A close-up view of transcription. As RNA nucleotides base- (b) Transcription of a gene. The transcription of an entire gene pair one by one with DNA bases on one DNA strand (called the occurs in three phases: initiation, elongation, and termination of the template strand), the enzyme RNA polymerase (orange) links the RNA. The section of DNA where the RNA polymerase starts is called RNA nucleotides into an RNA chain. the promoter; the place where it stops is called the terminator. 213 CHAPTER 10 THE STRUCTURE AND The Processing Exon Int ron Intron Exon FUNCTION OF DNA Exon of Eukaryotic RNA DNA Transcription In the cells of prokaryotes, which lack nuclei, the RNA Cap Addition of cap and tail RNA transcribed from a gene immediately functions as transcript messenger RNA (mRNA), the molecule that is trans- with cap and tail lated into protein. But this is not the case in eukaryotic Introns removed Tail cells. The eukaryotic cell not only localizes transcription in the nucleus but also modifies, or processes, the RNA transcripts there before they move to the cytoplasm for Exons spliced together translation by the ribosomes. One kind of RNA processing is the addition of extra mRNA nucleotides to the ends of the RNA transcript. These additions, called the cap and tail, protect the RNA from Coding sequence attack by cellular enzymes and help ribosomes recognize Nucleus the RNA as mRNA. Another type of RNA processing is made necessary in eukaryotes by noncoding stretches of nucleotides Cytoplasm that interrupt the nucleotides that actually code for amino acids. It is as if nonsense words were randomly interspersed within a recipe that you copied. Most genes of plants and animals, it turns out, include such internal noncoding regions, which are called Figure 10.13 The production of messenger RNA introns. The coding regions—the parts of a gene (mRNA) in a eukaryotic cell. Note that the molecule of that are expressed—are called exons. As Figure 10.13 mRNA that leaves the nucleus is substantially different from the molecule of RNA that was first transcribed from the gene. In illustrates, both exons and introns are transcribed the cytoplasm, the coding sequence of the final mRNA will be from DNA into RNA. However, before the RNA translated. CHECKPOINT leaves the nucleus, the introns are removed, and the Why is a final mRNA often exons are joined to produce an mRNA molecule with polypeptides. This is accomplished by varying the shorter than the DNA gene a continuous coding sequence. This process is called exons that are included in the final mRNA. that coded for it? removed from the RNA RNA splicing. RNA splicing is believed to play a With capping, tailing, and splicing completed, Answer: because introns are significant role in humans in allowing our approxi- the “final draft” of eukaryotic mRNA is ready for mately 21,000 genes to produce many thousands more translation. Translation: enzymes and sources of chemical energy, such as ATP. In addition, translation requires two other important The Players components: ribosomes and a kind of RNA called transfer RNA. As we have already discussed, transla- tion is a conversion between differ- Transfer RNA (tRNA) ent languages—from the nucleic acid Translation of any language into another requires an in- language to the protein language—and terpreter, someone or something that can recognize the it involves more elaborate machinery words of one language and convert them to the other. than transcription. Translation of the genetic message carried in mRNA into the amino acid language of proteins also requires an Messenger RNA (mRNA) interpreter. To convert the three-letter words (codons) The first important ingredient required for translation of nucleic acids to the amino acid words of proteins, a is the mRNA produced by transcription. Once it is pres- cell uses a molecular interpreter, a type of RNA called ent, the machinery used to translate mRNA requires transfer RNA (tRNA), depicted in Figure 10.14. 214 Figure 10.14 The structure of tRNA. At one end of the Ribosomes FROM DNA TO RNA tRNA is the site where an amino acid will attach (purple), and TO PROTEIN Ribosomes are the organelles in the cytoplasm that coor- at the other end is the three-nucleotide anticodon where the mRNA will attach (light green). dinate the functioning of mRNA and tRNA and actually make polypeptides. As you can see in Figure 10.15a, a Amino acid attachment site ribosome consists of two subunits. Each subunit is made up of proteins and a considerable amount of yet another kind of RNA, ribosomal RNA (rRNA). A fully assem- bled ribosome has a binding site for mRNA on its small subunit and binding sites for tRNA on its large subunit. Figure 10.15b shows how two tRNA molecules get to- Hydrogen bond gether with an mRNA molecule on a ribosome. One of the tRNA binding sites, the P site, holds the tRNA car- rying the growing polypeptide chain, while another, the CHECKPOINT RNA polynucleotide chain A site, holds a tRNA carrying the next amino acid to be What is an anticodon? added to the chain. The anticodon on each tRNA base- polypeptide. pairs with a codon on the mRNA. The subunits of the key step in translating mRNA to a pairing of anticodon to codon is a ribosome act like a vise, holding the tRNA and mRNA tary codon in the mRNA. The base molecules close together. The ribosome can then con- couples the tRNA to a complemen- Anticodon triplet of a tRNA molecule that tRNA nect the amino acid from the tRNA in the A site to the Answer: An anticodon is the base tRNA polynucleotide (simplified growing polypeptide. (ribbon model) representation) Figure 10.15 The ribosome. tRNA binding sites P site A site A cell that is producing proteins has in its cytoplasm a supply of amino acids. But amino acids themselves cannot recognize the codons arranged in sequence along Large messenger RNA. It is up to the cell’s molecular interpret- subunit mRNA Ribosome ers, tRNA molecules, to match amino acids to the appro- binding site priate codons to form the new polypeptide. To perform Small subunit this task, tRNA molecules must carry out two distinct functions: (1) pick up the appropriate amino acids and (a) A simplified diagram of a ribosome. Notice the two (2) recognize the appropriate codons in the mRNA. The subunits and sites where mRNA and tRNA molecules bind. unique structure of tRNA molecules enables them to perform both tasks. As shown on the left in Figure 10.14, a tRNA mol- Next amino acid ecule is made of a single strand of RNA—one poly- to be added to polypeptide nucleotide chain—consisting of about 80 nucleotides. Growing The chain twists and folds upon itself, forming several polypeptide double-stranded regions in which short stretches of RNA base-pair with other stretches. At one end of the folded molecule is a special triplet of bases called an tRNA anticodon. The anticodon triplet is complementary to a codon triplet on mRNA. During translation, the antico- mRNA don on the tRNA recognizes a particular codon on the mRNA by using base-pairing rules. At the other end of the tRNA molecule is a site where one specific kind of Codons amino acid attaches. Although all tRNA molecules are (b) The ”players“ of translation. When functioning in polypeptide synthesis, a ribosome holds one molecule similar, there are slightly different versions of tRNA for of mRNA and two molecules of tRNA. The growing poly- each amino acid. peptide is attached to one of the tRNAs. 215 CHAPTER 10 THE STRUCTURE AND Translation: The Process Elongation Once initiation is complete, amino acids are added one FUNCTION OF DNA Translation is divided into the same three phases as by one to the first amino acid. Each addition occurs in transcription: initiation, elongation, and termination. the three-step elongation process shown in Figure 10.18. Figure 10.16 A molecule The anticodon of an incoming tRNA molecule, car- Initiation rying its amino acid, pairs with the mRNA codon in of mRNA. This first phase brings together the mRNA, the first the A site of the ribosome. The polypeptide leaves Cap amino acid with its attached tRNA, and the two sub- the tRNA in the P site and attaches to the amino acid units of a ribosome. An mRNA molecule, even after on the tRNA in the A site. The ribosome creates a new Start of genetic message splicing, is longer than the genetic message it carries peptide bond. Now the chain has one more amino acid. (Figure 10.16). Nucleotide sequences at either end of the The P site tRNA now leaves the ribosome, and the molecule (pink) are not part of the message, but along ribosome moves the remaining tRNA, carrying the with the cap and tail in eukaryotes, they help the mRNA growing polypeptide, to the P site. The mRNA and bind to the ribosome. The initiation process determines tRNA move as a unit. This movement brings into the A exactly where translation will begin so that the mRNA site the next mRNA codon to be translated, and the pro- codons will be translated into the correct sequence of cess can start again with step 1. amino acids. Initiation occurs in two steps, as shown in Figure 10.17. An mRNA molecule binds to a small ribosomal subunit. A special initiator tRNA then binds Termination to the start codon, where translation is to begin on the Elongation continues until a stop codon reaches the ri- End mRNA. The initiator tRNA carries the amino acid me- bosome’s A site. Stop codons—UAA, UAG, and UGA— thionine (Met); its anticodon, UAC, binds to the start do not code for amino acids but instead tell translation codon, AUG. A large ribosomal subunit binds to the to stop. The completed polypeptide, typically several Tail small one, creating a functional ribosome. The initiator hundred amino acids long, is freed, and the ribosome tRNA fits into the P site on the ribosome. splits back into its subunits. Figure 10.17 The initiation of translation. Amino acid Met Polypeptide Large ribosomal subunit tRNA Initiator tRNA U C A P site A site A G P site U mRNA Anticodon mRNA A site 1 2 Start codon Small Codons ribosomal 1 Codon recognition subunit ELONGATION New peptide bond CHECKPOINT Which of the following does not participate directly mRNA in translation: ribosomes, ▶ Figure 10.18 movement transfer RNA, messenger The elongation of 2 Peptide bond formation RNA, DNA? a polypeptide. The Answer: DNA dashed red arrows 3 Translocation indicate movement. 216 Review: broadly, the way the genotype produces the phenotype. The flow of information originates with the specific FROM DNA TO RNA TO PROTEIN DNA → RNA → Protein sequence of nucleotides in a DNA gene. The gene dic- tates the transcription of a complementary sequence of Figure 10.19 reviews the flow of genetic information nucleotides in mRNA. In turn, the information within CHECKPOINT in the cell, from DNA to RNA to protein. In eukary- the mRNA specifies the sequence of amino acids in a 1. Transcription is the syn- otic cells, transcription (DNA → RNA) occurs in the polypeptide. Finally, the proteins that form from the thesis of _________, nucleus, and the RNA is processed before it enters the polypeptides determine the appearance and capabilities using _________ as a cytoplasm. Translation (RNA → protein) is rapid; a sin- of the cell and organism. template. gle ribosome can make an average-sized polypeptide in For decades, the DNA → RNA → protein pathway 2. Translation is the synthe- sis of _________, with less than a minute. As it is made, a polypeptide coils and was believed to be the sole means by which genetic in- one _________ deter- folds, assuming its final three-dimensional shape. formation controls traits. In recent years, however, this mining each amino acid What is the overall significance of transcription and notion has been challenged by discoveries that point to in the sequence. translation? These are the processes whereby genes more complex roles for RNA. (We will explore some of 3. Which organelle control the structures and activities of cells—or, more these special properties of RNA in Chapter 11.) coordinates translation? (polypeptides); codon 3. ribosomes Answers: 1. mRNA; DNA 2. protein Figure 10.19 A summary of transcription and translation. This figure summarizes the main stages in the flow of genetic information from DNA to protein in a eukaryotic cell. RNA polymerase Nucleus 1 Transcription: RNA is made on a DNA template. DNA mRNA Nucleus pore Intron 2 RNA processing: The RNA transcript is Anticodon spliced and modified to produce mRNA,