Molecular Biology Combined (Prof Gwira) PDF
Document Details
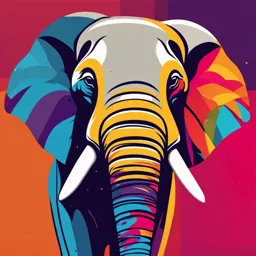
Uploaded by RewardingGarnet855
University of Ghana
2025
Prof Gwira
Tags
Summary
This document provides a summary of molecular biology concepts with focus on explaining "from DNA to protein". It also includes the genetic code and its interpretation. Details the process from DNA to the formation of amino acids, and also explains the concepts of codons, mRNA, and tRNA.
Full Transcript
BCMB 319: Molecular Biology From RNA to Protein January 20, 2025 DNA DNA RNA Protein Gene 1 Gene 3 From DNA to Protein DNA molecule Gene 2 DNA str...
BCMB 319: Molecular Biology From RNA to Protein January 20, 2025 DNA DNA RNA Protein Gene 1 Gene 3 From DNA to Protein DNA molecule Gene 2 DNA strand TRANSCRIPTION RNA Codon TRANSLATION Polypeptide Amino acid From DNA to Protein DNA is in the language of nucleotides RNA is in the language of nucleotides Polypeptides are in the language of amino acids Converting DNA to RNA is the process of transcription. Where does transcription take place? Summary of gene expression in eukaryotes 7. Degraded protein Three major types of RNA are transcribed mRNA (messenger RNA) - encodes genetic information from DNA & carries it into the cytoplasm rRNA (ribosomal RNA) - associates with proteins to form ribosomes tRNA (transfer RNA) - transports specific amino acids to ribosome during protein synthesis (translation). From DNA to Protein Virtually all organisms share the same genetic code! Just like we have 26 letters in the alphabet to construct words, the alphabet of DNA has 4 letters to use to construct polypeptides DNA template C A G T A A G C C RNA strand G U C A U U C G G So how is the code used to construct polypeptides? mRNA (messenger RNA) mRNA (messenger RNA) - encodes genetic information from DNA & carries it into the cytoplasm 5’ 3’ codon Each three consecutive mRNA bases forms a genetic code word (codon) that codes for a particular amino acid. The genetic code Deciphering the genetic code required determining how 4 nucleotides (A, T, G, C) could encode more than 20 amino acids. Francis Crick and Sydney Brenner determined that the DNA is read in sets of 3 nucleotides for each amino acid. 11 The genetic code Codon: set of 3 nucleotides that specifies a particular amino acid Reading frame: the series of nucleotides read in sets of 3 (codon) – only 1 reading frame is correct for encoding the correct sequence of amino acids 12 The genetic code The language of amino acids is based on codons 1 codon = 3 mRNA nucleotides 1 codon = 1 amino acid A UA U A U G C C C G C How many codons are in this sequence of mRNA? The genetic code The genetic code is a set of rules defining how the four-letter code of DNA is translated into the 20-letter code of amino acids, which are the building blocks of proteins. The genetic code is a set of three-letter combinations of nucleotides called codons, each of which corresponds to a specific amino acid or stop signal. The concept of codons was first described by Francis Crick and his colleagues in 1961. During the same year, Marshall Nirenberg and Heinrich Matthaei performed experiments that began deciphering the genetic code. They showed that the RNA sequence UUU specifically coded for the amino acid phenylalanine. Following this discovery, Nirenberg, Philip Leder, and Gobind Khorana identified the rest of the genetic code and fully described each three-letter codon and its corresponding amino acid. 14 The genetic code RNA molecules of only 1 nucleotide and of specific 3 base sequences were used to determine the amino acid encoded by each codon. The amino acids encoded by all 64 possible codons were determined. 15 Degeneracy of the genetic code Because there are 43=64 possible combinations of triplet codons, most amino acids are specified by more than one codon (degeneracy) 61 codons specify amino acids. Three do not (stop or termination codons). Termination codons tell ribosomes where to end translation of the mRNA. Most commonly, the AUG codon (specifying methionine) serves as the start codon and tells the ribosome where to begin translation. How to read the Triplet Code There are three potential reading frames in all mRNAs. However, only one reading frame is used for translation, and is selected based on the frame in which the AUG start codon appears. Triplet codons are read in a non- overlapping, comma-less manner. Rarely are mRNAs read in more than one frame. Likewise, frame- shifting is very uncommon. Features of the genetic code It’s degenerate - meaning that multiple codons can code for the same amino acid. For example, the amino acid serine, is coded by four different codons: UCU, UCC, UCA, and UCG. It’s universal - the codon CCA codes for proline in humans, in chickens, in bacteria, and so on. It is universal to all life on Earth except in a few cases (mitochondria). It’s nonoverlapping - codons are translated as consecutive nucleotide triplets, Codons do not overlap. The genetic code Using this chart, you can determine which amino acid the codon “codes” for! Which amino acid is encoded in the codon CAC? The genetic code Find the second letter of the codon CAC Find the first letter of the codon CAC Find the third letter of the codon CAC The genetic code CAC codes for the amino acid histidine (his) The genetic code What does the mRNA codon UAC code for? Tyr or tyrosine The genetic code Notice there is one start codon AUG. Transcription begins at that codon! The genetic code Notice there are three stop codons. Transcription stops when these codons are encountered. Key Points Properties of the Genetic Code The genetic code is composed of nucleotide triplets. The genetic code is nonoverlapping. (?) The genetic code is comma-free. (?) The genetic code is degenerate. (yes) The genetic code is ordered. (5’ to 3’) The genetic code contains start and stop codons. (yes) The genetic code is nearly universal. YES :) Key Points Genetic code: the sequence of nitrogenous bases in mRNA molecule which encloses information for the synthesis of protein molecules Codon: set of 3 nucleotides that specifies/codes for a particular amino acid Reading frame: the series of nucleotides read in sets of 3 (codon) – only 1 reading frame is correct for encoding the correct sequence of amino acids 26 Key Points Stop codons: 3 codons (UUA, UGA, UAG) in the genetic code used to terminate translation Start codon: the codon (AUG) used to signify the start of translation The remainder of the code is degenerate meaning that some amino acids are specified by more than one codon. 27 Key Points Each of the 20 amino acids in proteins is specified by one or more nucleotide triplets in mRNA. (20 amino acids refers to what is attached to the tRNAs!) Of the 64 possible triplets, given the four bases in mRNA, 61 specify amino acids and 3 signal chain termination. (have no tRNAs!) Key Points The code is nonoverlapping, with each nucleotide part of a single codon, degenerate, with most amino acids specified by two to four codons, and ordered, with similar amino acids specified by related codons. The genetic code is nearly universal; with minor exceptions, the 64 triplets have the same meaning in all organisms. Protein Synthesis (Activation and Initiation) Protein Structure Proteins are complex macromolecules composed of 20 (?) different amino acids Protein Structure Proteins are made of polypeptides. A polypeptide is a long chain of amino acids. Amino acids have a free amino group, a free carboxyl group, and a side group (R). Amino acids Peptide Bonds Amino acids are joined by peptide bonds. The carboxyl group of one amino acid is covalently attached to the amino group of the next amino acid. Protein Synthesis: Translation The genetic information in mRNA molecules is translated into the amino acid sequences of polypeptides according to the specifications of the genetic code. Translation/Protein synthesis The translation system consists of five major components: Messenger RNA: mRNA is needed to provide the coding sequence of bases that determines the amino acid sequence in the resulting polypeptide chain Ribosomes are particles on which protein synthesis takes place Transfer RNA: tRNA is a small adaptor molecule that translates codons into amino acid Aminoacyl-tRNA synthetases: set of molecules catalyzes the attachment of a particular amino acid to its corresponding tRNA molecule Initiation, elongation, and termination factors Ribosomes 38 Transfer RNA (tRNA) Are adaptors and consist of about 80 nucleotides in length. Molecular Biology of the cell 5th edition tRNAs are covalently modified before they exit from the nucleus Like most other eucaryotic RNAs, tRNAs are covalently modified before they are allowed to exit from the nucleus. Eucaryotic tRNAs are synthesized by RNA polymerase III. Both bacterial and eucaryotic tRNAs are typically synthesized as larger precursor tRNAs, which are then trimmed to produce the mature tRNA. some tRNA precursors (from both bacteria and eucaryotes) contain introns that must be spliced out. All tRNAs are modified chemically—nearly 1 in 10 nucleotides in each mature tRNA molecule is an altered version of a standard G, U, C, or A ribonucleotide. Over 50 different types of tRNA modifications are known Wobble base-pairing between codons and anticodons If the nucleotide listed in the first column is present at the third, or wobble, position of the codon, it can base-pair with any of the nucleotides listed in the second column. Thus, for example, when inosine (I) is present in the wobble position of the tRNA anticodon, the tRNA can recognize any one of three different codons in bacteria and either of two codons in eucaryotes. The inosine in tRNAs is formed from the deamination of, a chemical modification that takes place after the tRNA has been synthesized. (Adapted from C. Guthrie and J. Abelson, in The Molecular Biology of the Yeast Saccharomyces: Metabolism and Gene Expression, pp. 487–528. Cold Spring Harbor, New York: Cold Spring Harbor Laboratory Press, 1982.) Molecular Biology of the cell 5th edition Wobble base-pairing between codons and anticodons The nonstandard base pairs, including those made with inosine, are generally weaker than conventional base pairs. Note that codon–anticodon base pairing is more stringent at positions 1 and 2 of the codon: here only conventional base pairs are permitted. The differences in wobble base-pairing interactions between bacteria and eucaryotes presumably result from subtle structural differences between bacterial and eucaryotic ribosomes, the molecular machines that perform protein synthesis. (Adapted from C. Guthrie and J. Abelson, in The Molecular Biology of the Yeast Saccharomyces: Metabolism and Gene Expression, pp. 487–528. Cold Spring Harbor, New York: Cold Spring Harbor Laboratory Press, 1982.) Molecular Biology of the cell 5th edition Specific enzymes couple each amino acid to its appropriate tRNA molecule Recognition and attachment of the correct amino acid depends on enzymes called aminoacyl-tRNA synthetases, which covalently couple each amino acid to its appropriate set of tRNA molecules Most cells have a different synthetase enzyme for each amino acid (that is, 20 synthetases in all); one attaches glycine to all tRNAs that recognize codons for glycine, another attaches alanine to all tRNAs that recognize codons for alanine, and so on. Molecular Biology of the cell 5th edition Specific enzymes couple each amino acid to its appropriate tRNA molecule Many bacteria, however, have fewer than 20 synthetases, and the same synthetase enzyme is responsible for coupling more than one amino acid to the appropriate tRNAs. In these cases, a single synthetase places the identical amino acid on two different types of tRNAs, only one of which has an anticodon that matches the amino acid. A second enzyme then chemically modifies each “incorrectly” attached amino acid so that it now corresponds to the anticodon displayed by its covalently linked tRNA. Molecular Biology of the cell 5th edition The genetic code is translated by means of two adaptors that act one after another The first adaptor is the aminoacyl-tRNA synthetase, which couples a particular amino acid to its corresponding tRNA The second adaptor is the tRNA molecule itself, whose anticodon forms base pairs with the appropriate codon on the mRNA An error in either step would cause the wrong amino acid to be incorporated into a protein chain Molecular Biology of the cell 5th edition Amino acids are added to the C-terminal end of a growing polypeptide chain The fundamental reaction of protein synthesis is the formation of a peptide bond between the carboxyl group at the end of a growing polypeptide chain and a free amino group on an incoming amino acid. Consequently, a protein is synthesized stepwise from its N-terminal end to its C-terminal end. Throughout the entire process the growing carboxyl end of the polypeptide chain remains activated by its covalent attachment to a tRNA molecule (forming a peptidyl-tRNA). Molecular Biology of the cell 5th edition January 21, 2025 The RNA message is decoded in ribosomes The synthesis of proteins is guided by information carried by mRNA molecules. To maintain the correct reading frame and to ensure accuracy (about 1 mistake every 10,000 amino acids), protein synthesis is performed in the ribosome, a complex catalytic machine made from more than 50 different proteins (the ribosomal proteins) and several RNA molecules, the ribosomal RNAs (rRNAs). Molecular Biology of the cell 5th edition The RNA message is decoded in ribosomes A typical eukaryotic cell contains millions of ribosomes in its cytoplasm. Eukaryotic ribosome subunits are assembled at the nucleolus, when newly transcribed and modified rRNAs associate with ribosomal proteins, which have been transported into the nucleus after their synthesis in the cytoplasm Molecular Biology of the cell 5th edition Ribosomes Eukaryotic and prokaryotic ribosomes have similar designs and functions. Both are composed of one large and one small subunit that fit together to form a complete ribosome with a mass of several million daltons. The small subunit provides the framework on which the tRNAs can be accurately matched to the codons of the mRNA. The large subunit catalyzes the formation of the peptide bonds that link the amino acids together into a polypeptide chain 50 Ribosomes When not actively synthesizing proteins, the two subunits of the ribosome are separate. They join together on an mRNA molecule, usually near its 5’ end, to initiate the synthesis of a protein. The mRNA is then pulled through the ribosome; as its codons enter the core of the ribosome, The mRNA nucleotide sequence is translated into an amino acid sequence using the tRNAs as adaptors to add each amino acid in the correct sequence to the end of the growing polypeptide chain. 51 The RNA message is decoded in ribosomes They join together on an mRNA molecule, usually near its 5’ end, to initiate the synthesis of a protein. The mRNA is then pulled through the ribosome; as its codons enter the core of the ribosome, the mRNA nucleotide sequence is translated into an amino acid sequence using the tRNAs as adaptors to add each amino acid in the correct sequence to the end of the growing polypeptide chain. When a stop codon is encountered, the ribosome releases the finished protein, and its two subunits separate again. These subunits can then be used to start the synthesis of another protein on another mRNA molecule. Molecular Biology of the cell 5th edition The RNA-binding sites in the ribosome A ribosome contains four binding sites for RNA molecules: one is for the mRNA and three (called the A-site, the P-site, and the E-site) are for tRNAs A tRNA molecule is held tightly at the A- and P- sites only if its anticodon forms base pairs with a complementary codon (allowing for wobble) on the mRNA molecule that is threaded through the ribosome The A- and P-sites are close enough together for their two tRNA molecules to be forced to form base pairs with adjacent codons on the mRNA molecule. This feature of the ribosome maintains the correct reading frame on the mRNA. Molecular Biology of the cell 5th edition Translating an mRNA molecule Once protein synthesis has been initiated, each new amino acid is added to the elongating chain in a cycle of reactions containing four major steps: tRNA binding, peptide bond formation, large subunit and small subunit translocation. As a result of the two translocation steps, the entire ribosome moves three nucleotides along the mRNA and is positioned to start the next cycle. Translating an mRNA molecule In step 1, a tRNA (aminoacyl-tRNA molecule ) carrying the next amino acid in the chain binds to the ribosomal A-site by forming base pairs with the mRNA codon positioned there, so that the P-site and the A-site contain adjacent bound tRNAs. In step 2, the carboxyl end of the polypeptide chain is released from the tRNA at the P-site (by breakage of the high-energy bond between the tRNA and its amino acid) and joined to the free amino group of the amino acid linked to the tRNA at the A-site, forming a new peptide bond. This central reaction of protein synthesis is catalyzed by a peptidyl transferase contained in the large ribosomal subunit. Molecular Biology of the cell 5th edition Translating an mRNA molecule In step 3, the large subunit moves relative to the mRNA held by the small subunit, thereby shifting the acceptor stems of the two tRNAs to the E- and P sites of the large subunit. In step 4, another series of conformational changes moves the small subunit and its bound mRNA exactly three nucleotides, resetting the ribosome so it is ready to receive the next aminoacyl-tRNA. Step 1 is then repeated with a new incoming aminoacyl-tRNA, and so on. This four-step cycle is repeated each time an amino acid is added to the polypeptide chain, as the chain grows from its amino to its carboxyl end. Molecular Biology of the cell 5th edition Elongation factors drive translation forward and improve its accuracy Two elongation factors EF-Tu and EF-G, drive translation in the forward direction. Each hydrolyzing GTP to GDP and undergoing conformational changes in the process. Coupling the GTP hydrolysis- driven changes in the elongation factors to transitions between different states of the ribosome speeds up protein synthesis enormously. EF-Tu also provides two opportunities for proofreading of the codon–anticodon match. In this way, incorrectly paired tRNAs are selectively rejected, and the accuracy of translation is improved. Molecular Biology of the cell 5th edition Elongation factors drive translation forward and improve its accuracy Under some conditions in vitro, ribosomes can be forced to synthesize proteins without the aid of these elongation factors and GTP hydrolysis, but this synthesis is very slow, inefficient, and inaccurate. There are structural changes that almost certainly involve RNA structure rearrangements in the ribosome core. The cycles of elongation factor association, GTP hydrolysis, and dissociation ensure that all such changes occur in the “forward” direction so that translation can proceed efficiently Molecular Biology of the cell 5th edition Nucleotide sequences in mRNA signal where to start protein synthesis Initiation and elongation The translation of an mRNA begins with the codon AUG, and a special tRNA is required to start translation. This initiator tRNA always carries the amino acid methionine (in bacteria, a modified form of methionine—formylmethionine— is used), with the result that all newly made proteins have methionine as the first amino acid at their N-terminus, the end of a protein that is synthesized first. This methionine is usually removed later by a specific protease. The initiator tRNA can be specially recognized by initiation factors because it has a nucleotide sequence distinct from that of the tRNA that normally carries methionine Molecular Biology of the cell 5th edition Nucleotide sequences in mRNA signal where to start protein synthesis Initiation Step 1 - In eukaryotes, the initiator tRNA–methionine complex (Met–tRNAi) is first loaded into the small ribosomal subunit along with additional proteins called eukaryotic initiation factors, or eIFs Step 2 - the small ribosomal subunit binds to the 5’ end of an mRNA molecule, which is recognized by virtue of its 5’ cap and its two bound initiation factors, eIF4E (which directly binds the cap) and eIF4G Molecular Biology of the cell 5th edition Nucleotide sequences in mRNA signal where to start protein synthesis Step 3 - The small ribosomal subunit then moves forward (5’ to 3’) along the mRNA, searching for the first AUG. Additional initiation factors that act as ATP-powered helicases facilitate the ribosome’s movement through RNA secondary structure. Step 4 - In 90% of mRNAs, translation begins at the first AUG encountered by the small subunit. At this point, the initiation factors dissociate, allowing the large ribosomal subunit to assemble with the complex and complete the ribosome. The initiator tRNA is still bound to the P-site, leaving the Asite vacant. Protein synthesis is therefore ready to begin Molecular Biology of the cell 5th edition Nucleotide sequences in mRNA signal where to start protein synthesis The process translation require many translation initiation factors. Efficient translation initiation also requires the poly-A tail of the mRNA bound by poly-A-binding proteins which, in turn, interact with eIF4G. In this way, the translation apparatus ascertains that both ends of the mRNA are intact before initiating protein synthesis. Although only one GTP hydrolysis event is shown in the figure, a second is known to occur just before the large and small ribosomal subunits join. Molecular Biology of the cell 5th edition Nucleotide sequences in mRNA signal where to start protein synthesis Of all the aminoacyl-tRNAs in the cell, only the methionine- charged initiator tRNA is capable of tightly binding the small ribosome subunit without the complete ribosome being present and it binds directly to the P-site Molecular Biology of the cell 5th edition Translation initiation in prokaryotes The mechanism for selecting a start codon in bacteria is different. Bacterial mRNAs have no 5’ caps to signal the ribosome where to begin searching for the start of translation. Instead, each bacterial mRNA contains a specific ribosome binding site (called the Shine–Dalgarno sequence, named after its discoverers) that is located a few nucleotides upstream of the AUG at which translation is to begin. This nucleotide sequence, with the consensus 5’-AGGAGGU-3’, forms base pairs with the 16S rRNA of the small ribosomal subunit to position the initiating AUG codon in the ribosome. A set of translation initiation factors orchestrates this interaction, as well as the subsequent assembly of the large ribosomal subunit to complete the ribosome. Molecular Biology of the cell 5th edition Translation initiation in prokaryotes Unlike a eukaryotic ribosome, a bacterial ribosome can therefore readily assemble directly on a start codon that lies in the interior of an mRNA molecule, so long as a ribosome-binding site precedes it by several nucleotides. As a result, bacterial mRNAs are often polycistronic—that is, they encode several different proteins, each of which is translated from the same mRNA molecule. In contrast, a eucaryotic mRNA generally encodes only a single protein. Molecular Biology of the cell 5th edition Stop codons mark the end of translation Termination The end of the protein-coding message is signaled by the presence of one of three stop codons (UAA, UAG, or UGA). These are not recognized by a tRNA and do not specify an amino acid, but instead signal to the ribosome to stop translation. Step 1 - Proteins known as release factors bind to any ribosome with a stop codon positioned in the A site, forcing the peptidyl transferase in the ribosome to catalyze the addition of a water molecule instead of an amino acid to the peptidyl-tRNA. Molecular Biology of the cell 5th edition Stop codons mark the end of translation Step 2 - This reaction frees the carboxyl end of the growing polypeptide chain from its attachment to a tRNA molecule, and since only this attachment normally holds the growing polypeptide to the ribosome, the completed protein chain is immediately released into the cytoplasm. Step 3 - The ribosome then releases the mRNA and separates into the large and small subunits, which can assemble on this or another mRNA molecule to begin a new round of protein synthesis. Molecular Biology of the cell 5th edition The structure of a human translation release factor (eRF1) and its resemblance to a tRNA molecule eRF1 tRNA Molecular Biology of the cell 5th edition Proteins are made on polyribosomes The synthesis of most protein molecules takes between 20 seconds and several minutes. During this very short period, however, it is usual for multiple initiations to take place on each mRNA molecule being translated. As soon as the preceding ribosome has translated enough of the nucleotide sequence to move out of the way, the 5’ end of the mRNA is threaded into a new ribosome. Molecular Biology of the cell 5th edition Proteins are made on polyribosomes The mRNA molecules being translated are therefore usually found in the form of polyribosomes (or polysomes): large cytoplasmic assemblies made up of several ribosomes spaced as close as 80 nucleotides apart along a single mRNA molecule. These multiple initiations allow the cell to make many more protein molecules in a given time than would be possible if each had to be completed before the next could start. Molecular Biology of the cell 5th edition There are minor variations in the standard genetic code Incorporation of selenocysteine into a growing polypeptide chain Molecular Biology of the cell 5th edition There are minor variations in the standard genetic code Candida albicans, for example translates the codon CUG as serine, whereas nearly all other organisms translate it as leucine. Mitochondria (which have their own genomes and encode much of their translational apparatus) often deviate from the standard code. For example, in mammalian mitochondria AUA is translated as methionine, whereas in the cytosol of the cell it is translated as isoleucine. This type of deviation in the genetic code is “hardwired” into the organisms or the organelles in which it occurs. Molecular Biology of the cell 5th edition There are minor variations in the standard genetic code Translation recoding In this case, other nucleotide sequence information present in an mRNA can change the meaning of the genetic code at a particular site in the mRNA molecule. The standard code allows cells to manufacture proteins using only 20 amino acids. However, bacteria, archaea, and eukaryotes have available to them a twenty-first amino acid that can be incorporated directly into a growing polypeptide chain through translation recoding Selenocysteine, which is essential for the efficient function of a variety of enzymes, contains a selenium atom in place of the sulfur atom of cysteine. Molecular Biology of the cell 5th edition There are minor variations in the standard genetic code Translation recoding Selenocysteine is enzymatically produced from a serine attached to a special tRNA molecule that base-pairs with the UGA codon, a codon normally used to signal a translation stop. The mRNAs for proteins in which selenocysteine is to be inserted at a UGA codon carry an additional nucleotide sequence in the mRNA nearby that causes this recoding event Molecular Biology of the cell 5th edition There are minor variations in the standard genetic code Translational frameshifting This process allows more than one protein to be synthesized from a single mRNA. For example - Retroviruses, members of a large group of eukaryotic- infecting pathogens, commonly use translational frameshifting to make both the capsid proteins (Gag proteins) and the viral reverse transcriptase and integrase (Pol proteins) from the same RNA transcript. Molecular Biology of the cell 5th edition There are minor variations in the standard genetic code Translational frameshifting The virus needs many more copies of the Gag proteins than it does of the Pol proteins. This quantitative adjustment is achieved by encoding the Pol genes just after the Gag genes but in a different reading frame. Small amounts of the Pol gene products are made because, on occasion, an upstream translational frameshift allows the Gag protein stop codon to be bypassed. This frameshift occurs at a particular codon in the mRNA and requires a specific recoding signal, which seems to be a structural feature of the RNA sequence downstream of this site. Molecular Biology of the cell 5th edition Inhibitors of prokaryotic protein synthesis are useful as antibiotics Molecular Biology of the cell 5th edition Factors/Players in eukaryotic protein synthesis Summary mRNA – Codons for amino acids tRNA – Anto codons for codons of mRNA tRNA synthetases – Charging of tRNA Ribosomes – Act as ribozymes/site for trnaslation and peptidyl Transferase activity Initiation factors Elongation factors Termination factors January 27, 2025 Polysomes and Rapid Ribosome Recycling Increase the Efficiency of Translation Translation of a single eukaryotic mRNA molecule to yield a typical- sized protein takes 30–60 seconds Two phenomena significantly increase the overall rate at which cells can synthesize a protein: ---the simultaneous translation of a single mRNA molecule by multiple ribosomes (Polysomes) ---rapid recycling of ribosomal subunits after they disengage from the 3’ end of an mRNA. Model of protein synthesis on circular polysomes and recycling of ribosomal subunits Multiple copies of a cytosolic protein found in all eukaryotic cells, poly(A)-binding protein (PABPI), can interact with both an mRNA poly(A) tail and the 4G subunit of yeast eIF4. Moreover, the 4E subunit of yeast eIF4 binds to the 5’ end of an mRNA. As a result of these interactions, the two ends of an mRNA molecule can be bridged by the intervening proteins, forming a “circular” mRNA (Figure 4-30). Polysomes Key concepts to remember……… Of the two methionine tRNAs found in all cells, only one (tRNAi Met) functions in initiation of translation. Each stage of translation—initiation, chain elongation, and termination— requires specific protein factors including GTP-binding proteins that hydrolyze their bound GTP to GDP when a step has been completed successfully. During initiation, the ribosomal subunits assemble near the translation start site in an mRNA molecule with the tRNA carrying the amino-terminal methionine (Met-tRNAiMet) base-paired with the start codon Key concepts to remember……… Chain elongation entails a repetitive four-step cycle: Loose binding of an incoming aminoacyl-tRNA to the A site on the ribosome; Tight binding of the correct aminoacyl-tRNA to the A site accompanied by release of the previously used tRNA from the E site; Transfer of the growing peptidyl chain to the incoming amino acid catalyzed by large rRNA; Translocation of the ribosome to the next codon, thereby moving the peptidyl-tRNA in the A site to the P site and the now unacylated tRNA in the P site to the E site Key concepts to remember……… In each cycle of chain elongation, the ribosome undergoes two conformational changes monitored by GTP-binding proteins. The first permits tight binding of the incoming aminoacyl-tRNA to the A site and ejection of a tRNA from the E site, and the second leads to translocation. Termination of translation is carried out by two types of termination factors: those that recognize stop codons and those that promote hydrolysis of peptidyl-tRNA The efficiency of protein synthesis is increased by the simultaneous translation of a single mRNA by multiple ribosomes. In eukaryotic cells, protein-mediated interactions bring the two ends of a polyribosome close together, thereby promoting the rapid recycling of ribosomal subunits, which further increases the efficiency of protein synthesis Some proteins begin to fold while still being synthesized Translation of an mRNA sequence into an amino acid sequence on the ribosome is not the end of the process of forming a protein. To function, the completed polypeptide chain must fold correctly into its three-dimensional conformation, bind any cofactors required, and assemble with its partner protein chains (if any). Noncovalent bond formation drives these changes. however, many proteins also require covalent modifications of selected amino acids. Although the most frequent modifications are protein glycosylation and protein phosphorylation, more than 100 different types of covalent modifications are known Molecular Biology of the cell 5th edition Molecular chaperones help guide the folding of most proteins For most proteins, they are met at the ribosome by a special class of proteins called molecular chaperones. Molecular chaperones are useful for cells because there are many different paths that can be taken to convert an unfolded or partially folded protein to its final compact conformation. For many proteins, some of the intermediates formed along the way would aggregate and be left as off-pathway dead ends without the intervention of a chaperone. Molecular Biology of the cell 5th edition Molecular chaperones help guide the folding of most proteins Many molecular chaperones are called heat-shock proteins (designated Hsp), because they are synthesized in dramatically increased amounts after a brief exposure of cells to an elevated temperature (for example, 42°C for cells that normally live at 37°C). There are several major families of eukaryotic molecular chaperones, including the Hsp60 and Hsp70 proteins. Different family members function in different organelles. For example, mitochondria contain their own Hsp60 and Hsp70 molecules that are distinct from those that function in the cytosol; and a special Hsp70 (called BIP) helps to fold proteins in the endoplasmic reticulum. Molecular Biology of the cell 5th edition Molecular chaperones help guide the folding of most proteins The Hsp60 and Hsp70 proteins each work with their own small set of associated proteins when they help other proteins to fold. Hsps share an affinity for the exposed hydrophobic patches on incompletely folded proteins, and they hydrolyze ATP, often binding and releasing their protein substrate with each cycle of ATP hydrolysis. In other respects, the two types of Hsp proteins function differently. Molecular Biology of the cell 5th edition Molecular chaperones help guide the folding of most proteins The Hsp70 machinery acts early in the life of many proteins, binding to a string of about seven hydrophobic amino acids before the protein leaves the ribosome Post-translational protein folding Molecular Biology of the cell 5th edition Molecular chaperones help guide the folding of most proteins Hsp60-like proteins form a large barrel-shaped structure that acts after a protein has been fully synthesized. This type of chaperone, sometimes called a chaperonin, forms an “isolation chamber” into which misfolded proteins are fed, preventing their aggregation and providing them with a favorable environment in which to attempt to refold Post-translational protein folding Molecular Biology of the cell 5th edition Co-translational protein folding A growing polypeptide chain is shown acquiring its secondary and tertiary structure as it emerges from a ribosome. The N-terminal domain folds first, while the C- terminal domain is still being synthesized Molecular Biology of the cell 5th edition Ubiquitin-mediated proteolytic pathway (a) Enzyme E1 is activated by attachment of a ubiquitin (Ub) molecule (step 1 ) and then transfers this Ub molecule to E2 (step 2 ). Ubiquitin ligase (E3) transfers the bound Ub molecule on E2 to the side-chain —NH2 of a lysine residue in a target protein (step 3 ). Additional Ub molecules are added to the target protein by repeating steps – , forming a polyubiquitin chain that directs the tagged protein to a proteasome (step 4 ). Within this large complex, the protein is cleaved into numerous small peptide fragments (step 5 ). (b) Computer-generated image reveals that a proteasome has a cylindrical structure with a cap at each end of a core region. Proteolysis of ubiquitin-tagged proteins occurs along the inner wall of the core. [Part (b) from W. Baumeister et al., 1998, Cell 92:357; courtesy of W. Baumeister.] Protein synthesis by free and membrane-bound ribosomes 15 Protein import by membrane- bound organelles ATP !!! 16 Molecular Biology of the Cell, 5th edition Molecular Cell Biology, Lodish 5th edition Overview of the secretory and endocytic pathways of protein sorting 1. Secretory pathway: Synthesis of proteins bearing an ER signal sequence is completed on the rough ER 2. the newly made polypeptide chains are inserted into the ER membrane or cross it into the lumen (Chapter 16). Some proteins (e.g., ER enzymes or structural proteins) remain within the ER. The remainder are packaged into transport vesicles 3. that bud from the ER and fuse together to form new cis- Golgi cisternae. Missorted ER-resident proteins and vesicle membrane proteins that need to be reused are retrieved to the ER by vesicles Molecular Cell Biology, Lodish 5th edition Overview of the secretory and endocytic pathways of protein sorting 4. that bud from the cis-Golgi and fuse with the ER. Each cis-Golgi cisterna, with its protein content, physically moves from the cis to the trans face of the Golgi complex 5. by a nonvesicular process called cisternal progression. Retrograde transport vesicles 6. move Golgi-resident proteins to the proper Golgi compartment. In all cells, certain soluble proteins move to the cell surface in transport vesicles Molecular Cell Biology, Lodish 5th edition Overview of the secretory and endocytic pathways of protein sorting 7. and are secreted continuously (constitutive secretion). In certain cell types, some soluble proteins are stored in secretory vesicles 8. and are released only after the cell receives an appropriate neural or hormonal signal (regulated secretion). Lysosome-destined membrane and soluble proteins, which are transported in vesicles that bud from the trans-Golgi 9. first move to the late endosome and then to the lysosome. Endocytic pathway: Membrane and soluble extracellular proteins taken up in vesicles that bud from the plasma membrane (also can move to the lysosome via the Endosome). Molecular Cell Biology, Lodish 5th edition Protein trafficking Signal sequences direct proteins to the correct cell ‘’address’’ ❖ These signal sequences are often found at the N-terminus ❖Signal peptidases remove the signal sequence from the finished protein once the sorting process is complete Molecular Biology of the Cell, 5th edition Signal sequences Molecular Biology of the Cell, 5th edition Molecular basis of gene mutations January 28, 2025 Mutation Changes in DNA that affect genetic information A change in the nucleotide sequence of a gene ◆ May occur at the molecular or chromosomal level ◆ The effect of mutations vary ◆ Mutations are important to evolution ◆ Mutant refers to an unusual phenotype Mutations Somatic Mutations ◆ Occur in cells of the body, excluding the germ cells ◆ Affects descendants of subsequent somatic cell But not transmitted to offspring Germ-line Mutations ◆Mutations that occur in the germ cells ◆Possibility of transmission to offspring Mutations in DNA can alter proteins ❑ Examples of mutations that cause disease: ◆ Beta globin gene ❖ Mutations can cause cells to sickle ❖ Sickle cell disease results from a base change in Beta Globin Gene ❖ Glutamic acid → Valine ◆ Collagen - deletions, insertions, RNA splicing mutations, and single-base substitutions more common disorders such as Nature. 2008 June 19; 453(7198): 998–999. doi:10.1038/453998a. osteoarthritis, osteoporosis, and aortic aneurysms ◆ Alzheimer’s disease – mutations in APP, APOE, PSEN1 and PSEN2 https://answers.childrenshospital.org/alzheimers- Mutations May occur spontaneously or by exposure to a radiation or chemicals A mutagen - an agent that causes a mutation Spontaneous mutation Not caused by exposure to known mutagen Errors in DNA replication Induced mutation Caused by mutagens, many are also carcinogens and cause cancer ◆ Radiations ❖ UV light: specific DNA base alterations ❖ X-rays: chromosome breaks and translocation natural or synthetic chemicals ◆ Site-directed mutagenesis - a method that is used to make specific and intentional mutating changes to the DNA sequence of a gene and any gene products Types of mutations A mistake that changes one base on a DNA molecule is called a point mutation. Two forms: – Transition: one pyrimidine (T or C) substituted for the other pyrimidine or one purine substituted for the other purine (A or G). – Transversion: purine substituted for pyrimidine or vice versa Point mutation ❑ A change of a single nucleotide ◆ Purine replaces purine A → G or G → A or ◆ Pyrimidine replaces pyrimidine C → T or T → C ◆Purine replaces pyrimidine or A or G → T or C ◆ Pyrimidine replaces purine T or C → A or G Types of mutations Not all mutations cause a change in amino acid coded for. These are called silent mutations. Mutations that do cause a change in amino acid are called replacement mutations. Not all mutations affect protein function ❑ Silent mutations ◆ Are mutations that do not alter the amino acid encoded Example: ❖ A mutation from AAA to AAG alters the DNA, the protein sequence remains unchanged ❖ AAA and AAG both encode for lysine ❖ These codons are called synonymous codons Missense Mutation A point mutation that changes the codon Causes a substitution of an amino acid Mutations may affect protein function severely or mildly CTC → CAC; Glutamic acid → Valine, results in sickle cell disease Nonsense mutation A point mutation changing a codon for an amino acid into a stop codon Creates truncated proteins that are often nonfunctional Some have dominant effects due to interference with normal functions Insertion or deletion mutations The genetic code is read in triplet nucleotides Addition or subtraction of a nucleotide not in multiples of three leads to a change in the reading frame Causes a frameshift and alters amino acids after mutation Addition or subtraction of nucleotides in multiples of three leads to addition or subtraction of entire amino acids Summary of mutations Mutation rates Some mutations cause readily identified phenotypic changes. E.g. Achrondoplastic dwarfism is a dominant disorder. An Achrondoplastic individual’s condition must be the result of a mutation, if his parents do not have the condition. Mutation rates Human estimate is 1.6 mutations/genome/generation. In Drosophila rate is only 0.14 m/g/g, but when corrected for number of cell divisions needed to produce sperm (400 in humans 25 in Drosophila) mutation rates per cell division are very similar. Mutation rates These rates are underestimates as they are based on loss-of-function mutations. Direct estimate of number of mutations of all kinds made for roundworm Caenorhabditis elegans by sequencing mitochondrial DNA. DNA repair mechanisms DNA Repair DNA repair is very important in a wide range of organisms. This is evident from the large investment that cells make in DNA repair enzymes. For example, analysis of the genomes of bacteria and yeasts has revealed that several percent of the coding capacity of these organisms is devoted solely to DNA repair functions. The importance of DNA repair is also demonstrated by the increased rate of mutation that follows the inactivation of a DNA repair gene. DNA Repair ❑ Excision Repair ◆ Damaged DNA is removed by excision of the bases ◆ Bases are replaced by a DNA polymerase ❑ Nucleotide excision repair ◆ Replaces up to 30 bases ◆ Used in repair of UVB and some carcinogens ❑ Base excision repair ◆ Replaces 1-5 bases ◆ Repairs oxidative damage DNA Repair ❑ Mismatch Repair ◆ Enzymes detect nucleotides that do not base pair in newly replicated DNA ◆ The incorrect base is excised and replaced ◆ Proofreading is the detection of mismatches DNA Repair ❑ Errors in DNA replication or damage to DNA create mutations ❑ Most errors and damage are repaired ❑ Type of repair depends upon the type of damage or error ❑ Different organisms vary in their ability to repair DNA Without DNA repair spontaneous DNA damage would rapidly change DNA sequences Although DNA is a highly stable material, as required for the storage of genetic information, it is a complex organic molecule that is susceptible, even under normal cell conditions, to spontaneous changes that would lead to mutations if left unrepaired For example, the DNA of each human cell loses about 5000 purine bases (adenine and guanine) every day because their N-glycosyl linkages to deoxyribose hydrolyze, a spontaneous reaction called depurination. Molecular Biology of the cell 5th edition Without DNA repair spontaneous DNA damage would rapidly change DNA sequences Similarly, a spontaneous deamination of cytosine to uracil in DNA occurs at a rate of about 100 bases per cell per day (Figure 5-45). DNA bases are also occasionally damaged by an encounter with reactive metabolites produced in the cell (including reactive forms of oxygen) or by exposure to chemicals in the environment. Molecular Biology of the cell 5th edition Without DNA repair spontaneous DNA damage would rapidly change DNA sequences Ultraviolet radiation from the sun can produce a covalent Iinkage between two adjacent pyrimidine bases in DNA to form, for example, thymine dimers (Figure 5-46). If left uncorrected when the DNA is replicated, most of these changes would be expected to lead either to the deletion of one or more base pairs or to a base-pair substitution in the daughter DNA chain (Figure 5-47). The mutations would then be propagated throughout subsequent cell generations. Such a high rate of random changes in the DNA sequence would have disastrous consequences for an organism. Molecular Biology of the cell 5th edition How chemical modifications of nucleotides produce mutation Deamination of cytosine Depurination can lead to the loss of a nucleotide pair mutated mutated Deaminated C Depurinated A A G has been an A-T nucleotide pair changed to an A has been deleted unchanged unchanged Molecular Biology of the cell 5th edition DNA damage can be removed by more than one pathway The two most common pathways are (1) base excision repair and (2) nucleotide excision repair. Base excision repair Nucleotide excision repair EXCISTON URACIL DNA NUCLEASE GLYCOSYLASE DNA AP ENDONUCLEASE END HELICASE PHOSPHODIESTERASE REMOVE SUGAR PHOSPHATE DNA helix with 12- Nucleotide gap DNA POLYMERASE PLUS DNA LIGASE Molecular Biology of the cell 5th edition Double-strand breaks are efficiently repaired An especially dangerous type of DNA damage occurs when both strands of the double helix are broken, leaving no intact template strand to enable accurate repair. Ionizing radiation, replication errors, oxidizing agents, and other metabolites produced in the cell cause breaks of this type There are two different ways to repair double-strand breaks (A) Nonhomologous end-joining (B) Homologous recombination alters the original DNA sequence More difficult to accomplish but this type of when repairing a broken chromosome. repair restores the original DNA sequence These alterations can be either deletions or short insertions Nonhomologous end-joining predominates in humans; homologous recombination is used only during and shortly after DNA replication (in S and G2 phases), when sister chromatids are available to serve as templates. Molecular Biology of the cell 5th edition