Sensory Systems - Human Physiology - PDF
Document Details
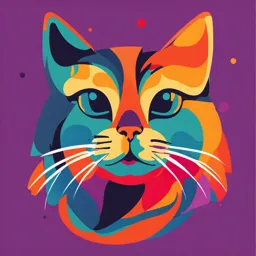
Uploaded by AgreeableSugilite5285
Utah Tech University
Glorimar Aponte-Kline
Tags
Summary
This presentation provides an overview of the sensory systems within the human body. It details the afferent division of the nervous system and explores the special senses including olfaction (smell), gustation (taste) and vision. The information covers anatomical structures, pathways, and transduction processes within the olfactory, gustatory, and visual systems.
Full Transcript
Advanced Human Physiology Chapters 9B Sensory Systems: Special Senses Lectures by Glorimar Aponte-Kline M.D. Utah Tech University Afferent Division of Nervous System Recall from...
Advanced Human Physiology Chapters 9B Sensory Systems: Special Senses Lectures by Glorimar Aponte-Kline M.D. Utah Tech University Afferent Division of Nervous System Recall from Chapter 7 that the afferent division of the neurons convey AP’s from receptor in the somatic or special senses to CNS. In this chapter we will be emphasizing on the Sensory Afferent Division of the Nervous system. This chapter discusses sensory information that reaches the conscious and subconscious level of perception. Recall: Stimulus Receptor Ascending signal/pathway Integrator Functional areas of the cerebral cortex: Special Senses Special Senses: The Olfactory System Olfactory Epithelium- occupies superior nasal concha - contains 3 types of cells (anatomical structure and function) 1) Olfactory receptor cells (sensory neurons) bipolar neurons: distal end is a single knob shaped terminal of the olfactory receptor cell; dendrite with olfactory cilia, that is embedded in the olfactory epithelium. The cilia contain olfactory receptor proteins (G Protein –linked receptor) that are embedded in mucus. Proximal end is a single olfactory receptor cell axon (CN I) that carries information to olfactory bulb which contain glomeruli and mitral cells where it synapses with secondary sensory neuron. 2) Supporting cells- are columnar epithelial cells of the mucus membrane lining the nose. Help detoxify chemicals. a) Basal cells – are stem cells that are located in olfactory epithelium between bases of supporting & olfactory receptor cells. Unlike other neurons, basal cells continue to divide to produce receptor cells and turnover about every two months. Chemoreception: Olfaction (Smell) Olfactory pathway: Anatomical structures and functions: 1. Olfactory Bulb- paired masses of gray mater located on underside of frontal lobe. It contains secondary sensory neurons. 2. Olfactory Tract- formed by axons of olfactory bulbs project to olfactory cortex. 3. Olfactory Cortex- located inferior and medial to surface of temporal lobe. It connects to limbic system, & cerebral cortex. Olfactory neurons show rapid adaptation- 50% in first second of exposure to a constant odor, but adapts very slowly thereafter. Chemoreception: Olfaction (smell) Olfaction pathway: (steps 1-6) Step 1: First, the odorant chemical molecules must dissolve in and penetrate mucus before binding to olfactory receptor protein in the plasma membrane of an olfactory cilium. Step 2: Binding of the odorant molecule to the olfactory receptor protein stimulates G protein Golf. Step 3: Golf activates adenyl cyclase to produce the second messenger cAMP. Step 4: cAMP opens cation channels that allow mainly Na+ and Ca+ ions to enter the cytosol. Step 5: The influx of Na+ and Ca+ causes a depolarizing receptor potential to form in the membrane of the olfactory receptor cell. Step 6: If the depolarization reaches Chemoreception: Olfaction (Smell) Steps 7- 10 in pathway : Step 7: The olfactory neurons synapse in the olfactory bulb, which contain glomeruli. Each glomeruli receives input from one type of olfactory receptor. Within glomeruli axons converge onto mitral cells –second order neurons. where integration and sorting of smells takes place to some degree. Step 8:Sensory neurons in the olfactory bulb conduct action potentials along mitral cell axons, which bundle together to form the olfactory tract. Step 9:Axons of olfactory tract carry this information to the olfactory cortex on the temporal lobe where conscious awareness of smell occurs. Step 10: then to the limbic system and the cerebral cortex. Where there is a link between smell, memory and emotions. The brain uses information coming from hundreds of different olfactory receptors to create perception of many different complex smells. Olfaction does NOT go first to the thalamus. Chemoreception: Gustation (taste) Taste is closely linked to olfaction. Taste receptors are non-neuronal epithelial cells with microvilli and these microvilli contain protein (chemical) receptor molecules in their membrane. There are five basic tastes: sour , sweet, bitter, S o Bitter S o salty and umami (MSG). u u r r Each taste cell is sensitive to only one taste. sweet These taste receptor cells are located mostly in the Salty taste buds, clustered on the surface of the tongue. Each taste bud is composed of 50-150 taste receptor cells (TRC’s), support cells and regenerative basal cells. Taste buds are innervated by CN’s VII (facial nerve) anterior 2/3 of tongue, IX (glossopharyngeal nerve) posterior 1/3 of tongue, and X (vagus nerve) innervates scattered taste buds on surface of throat and epiglottis. A gustatory epithelial cell lives only 10-12 days before it is replaced. Chemoreception: Gustation Anatomy and Function of Taste receptors Receptor mediated transduction-ligand binds with taste receptor cell Supporting cells- salty & sour triggered by influx of Na+ (Type II) ion channel or H+ ion Gustatory receptor cells (Type I) respond to presence of bitter, sweet & umami –G protein coupled receptor (GPCR) activate Gustducin Basal cells (stem cells)- are precursors of taste receptor cells. Taste Transduction: Salty & Sour Before the transduction pathway occurs, the taste chemical (tastant/ligand) dissolves in the saliva and mucus of mouth. The dissolved taste ligand then undergoes a transduction pathway. Transduction pathway for salty or sour occurs in the following way: Step 1: Na+ ion in a salty tastant or H+ ions in a sour tastant enter a gustatory receptor cell through Na+ or H+ channels. Step 2: The influx of Na+ or H+ ions into the cell causes a depolarization receptor potential. Step 3:The depolarization causes voltage-gated Ca+ channels in the plasma membrane to open, allowing Ca+ ions to flow into the cell. Step 4: Increase in intracellular Ca+ stimulates the synaptic vesicle to release NT into the synaptic cleft. Once the NT is released, it crosses the synaptic cleft and binds to and excites the first order neuron. NOTE: Unlike what is shown, an individual gustatory receptor cell responds to only one type of tastant. Each gustatory receptor cell has either ion channels or receptors for only one of the primary tastes. Taste Transduction: Sweet, Bitter, Umami Before the transduction pathway occurs, the taste chemical (tastant/ligand) dissolves in the saliva and mucus of mouth. The dissolved taste ligand then undergoes a transduction pathway. Transduction pathway for sweet, bitter and umani occurs in the following way: Step 1: A sweet, bitter, or umami tastant binds to a specific receptor on the plasma membrane that is coupled to a G-protein, Gustducin. Gustducin then activates the enzyme phospholipase C to produce the second messenger inositol triphosphate (IP3). Step 2: IP3 binds to and opens transient receptor potential (TRP) channels (TRPM5) that are present in the plasma membrane. Step 3: Once TRP channels open there is an influx of Na+ ions into the cell, resulting in formation of a depolarizing receptor potential. Step 4: The depolarization in turn causes voltage-gated Ca+ channels in plasma membrane to open, allowing Ca+ ions to enter the cell. Step 5: IP3 binds to and opens IP3 –gated Ca+ channels in the membrane of the endoplasmic reticulum (ER) releasing Ca + into the cytosol. Step 6: The increase in intracellular Ca + due to the opening of voltage- gated Ca+ channels and IP3 –gated Ca+ channels stimulates the synaptic vesicle to release NT into the synaptic cleft. Once the NT is released, it TRPMS = Transient Receptor Potential, Metastatin subfamily, crosses the synaptic cleft and binds to and excites the first order neuron. member 5 An individual gustatory receptor cell responds to only one type of tastant. Gustatory Pathway The last two steps of the Gustatory Pathway after transduction, are the following: Step 5/7: The gustatory neurons, that innervate the taste buds, have axons that run m through CN’s VII, IX, X and synapse at the gustatory nucleus of the medulla. Step 6/8: then the sensory information passes through the thalamus, to the gustatory cortex in the insula for central processing. General Pathway of light rays from the cornea of the eye to the cerebral cortex Cornea anterior chamber and aqueous humor pupil lens vitreous chamber and vitreous humor photo receptors in retina (rods & cones) optic nerve fibers optic chiasm optic tract lateral geniculate nucleus (body) of the thalamus visual cortex of the occipital lobe of the cerebral cortex Pathways for vision Optic Optic Optic Visual cortex Lateral geniculate Optic radiations Light passes through cornea pupil lens photo nerve chiasm tract (occipital lobe) body (thalamus) receptors in retina Collateral pathways leave Eye the thalamus and synapse in the midbrain to control collaterals constriction of the pupils. Light Midbrain Cranial nerve III controls pupillary constriction. Anatomy of the Eye: Accessory Structures Eye is protected in orbits by the bones of the skull Eyebrows & eyelashes – protect eyes from foreign objects, perspiration and sun rays. Upper and lower eyelids – shade eyes and protect eyes from foreign objects and excessive light. Lacrimal apparatus – group of glands, canals and ducts that produce and drain lacrimal fluid or tears (lysozyme). Washes exposed surface with tears. Extrinsic muscles- superior & inferior rectus and oblique muscles as well as lateral and medial rectus muscles. They are responsible for eye movements. Pupil is an opening in iris which can change diameter to let less or more light through. Iris is the colored ring of pigment containing circular and radial muscles. © 2016 Pearson Education, Inc. Anatomy of the Eye: Components Cornea– transparent tissue (extension of sclera), that causes light to refract a small amount. Sclera – “white” part of eye covers eyeball; gives shape to the eye. Choroid – lines inner surface of sclera, contains blood vessels, pigment melanin (absorbs light) Ciliary body – extension of the choroid, contains smooth muscle, secretes aqueous humor Zonular fibers (suspensory ligaments) – attached to lens, alters shape of the lens when viewing objects Iris – colored portion of eye Pupil – hole in the center of the iris Lens - transparent tissue with 2 convex surfaces that causes light to refract and is adjustable, so degree of refraction can be changed to focus light onto back of retina. Macula lutea – oval area in center of posterior retina. Responsible for central vision. Fovea – in center of macula lutea, area of highest visual acuity or resolution. Optic disc (blind spot) – circular area where optic nerve and blood vessels exit retina. It does not contain photoreceptors. Anterior Cavity – in front of the lens filled with aqueous humor covered by cornea. - replaced Posterior Cavity – behind the lens, larger vitreous chamber filled with vitreous humor – not replaced Canal of Schlemm – Opening where sclera and cornea meet. Drains aqueous humor. Responses of the pupil to light Pupil is an opening in iris which can change diameter to let less or more light through. Light enters the eye through pupil Size of the pupil modulates the amount of light that reaches photoreceptors Shape of lens focuses the light Iris is the colored ring of pigment containing pupillary smooth muscles: circular and radial muscles. Constrict (Circular muscle) in response to parasympathetic nervous system in bright light Dilate (radial muscle) in response to sympathetic nervous system in dim light Standard part of neurological examination is the Pupillary and Consensual reflexes. Pupillary reflex is a consensual reflex: Pupillary reflex; light shown in left eye causes pupil to constrict. Consensual reflex ; light shown in left eye causes right pupil to constrict. CNIII controls pupillary reflex. Organization of the retina Inner layer: Retina- converts light into AP’s A) Pigmented layer – contains melanin, helps absorb stray light rays. B) Neural layer – 3 layers that process visual signals. 1) Photoreceptor layer – consists of rods and cones that detect light and convert it into receptor potentials. 2) Bipolar cell layer- neurons that convey signals from photoreceptors to ganglion cells. 3) Ganglion cell layer- neurons receive signals from bipolar cells and generate AP’s. Axons give rise to optic nerve (CN II). Lens: Refraction of light rays The eye forms clear images of objects on retina via 3 processes: 1) The refraction or bending of light by the lens, and cornea 2) Accommodation 3) Constriction or narrowing of the pupil – covered previously Light entering eye is refracted, or bent. At the cornea and lens Refraction influenced by the angle at which light meets the lens Refraction depends on whether the surface of the lens is concave or convex. If parallel light rays strike a concave lens the light rays diverge If parallel light rays strike a convex lens (normal shape of cornea and lens) it causes the light rays to Lens: Accommodation Sympathetic stimulation Process by which the eye adjusts lens shape to keep objects in focus. Near point of accommodation is the closest distance at which the lens can focus an object During accommodation, the Parasympathetic stimulation lens flattens when we focus on a distant object; the lens becomes rounder to focus the image of nearby object on retina Refraction Abnormalities Emmetropic eye – normal eye, can refract light rays from an object 20 ft away so that a clear image is focused on the retina. Myopia (or near-sightedness) Focal point falls in front of the retina Eyeball too long, or lens more convex Can’t focus on far objects, but can focus on near objects. Hyperopia AKA Hypermetropia (or far- sightedness) Focal point falls behind the retina Eyeball too short, lens less convex Can’t focus on near object, but can focus on far objects. Astigmatism Usually caused by irregular curvature of lens or cornea, resulting in distorted images Photoreceptors: Rods & Cones Rods function well in low light and are used in night vision (black & white) Cones are responsible for high- acuity vision and color vision during the daytime Outer segment: light transduction. Contain discs of rods and cones Inner segment : contains cell nucleus and organelles. Synaptic terminal: is filled with synaptic vessels (with NT) and synapsis with bipolar cells. Photoreceptors Visual pigments convert light energy into a change in membrane potential Rods contain rhodopsin which absorbs most wavelengths of light. Cones contain three opsin pigments primary excited by red, green, and blue light which allows us to see color. Color-blindness – an inherited defect in one or more cone types thus, have difficulty distinguishing colors Photopigments: cyclical bleaching and regeneration opsi n Photopigment in rods and cones contain 2 parts: retinal (light absorbing portion of pigment; vitamin A derivative) and opsin (protein). Photopigments respond to light via the following cyclic process: 1) Isomerization- is the cis to trans conversion of retinal. In darkness retinal has bent shape= cis-retinal. When cis-retinal absorbs a photon of light, it straightens out = trans- retinal. 2) Bleaching – trans-retinal completely separates from opsin and becomes colorless. 3) Conversion – enzyme, retinal isomerase converts trans-retinal back to cis-retinal. 4) Regeneration – resynthesis of photopigment via binding of cis-retinal to opsin. Distribution of Photoreceptors in Retina Most light entering eye must pass through three neuron cell layers (ganglion cells, bipolar cells and photoreceptors) before striking the pigmented epithelium lining the Direction of visual processing back of the retina. The pigmented epithelium absorbs any light that was not absorbed by the pigments in the photoreceptors to be stimulated. An exception is the fovea, where the ganglion cells and bipolar cells have been pulled to the side and photoreceptors receive light directly. The fovea and macula immediately surrounding it are the sites of greatest visual acuity and the best color vision because only cones are there. Phototransduction in Rods Phototransduction is described for a rod, but similar in a cone. When a rod is in darkness and the photopigment (Rhodopsin) is not stimulated by light, cis-retinal binds tightly to opsin, high cGMP is produced which binds to and opens nonselective ion cation channels which allow the inflow of Na+ into cytosol = dark current, depolarizing the membrane to –40mV. This depolarization reaches the synaptic terminal and opens voltage gated Ca+ channels. Ca + influx triggers the exocytosis of the synaptic vessels containing neurotransmitter onto the bipolar cell. This means that the bipolar cells (get turned off) don’t excite the ganglion cells so no action potentials are sent to the Bipolar cell brain. Phototransduction in Rods When the right wavelength of light hits the retina and is absorbed by the photo- pigments, cis-retinal undergoes isomerization to trans-retinal and the photopigment is said to be bleached. The isomerization activates the G-protein, transducin, which activates cGMP phosphodiesterase. This causes the breakdown of triggers the cGMP which lowers the concentration of cGMP In the cytosol, resulting in reduction of open cGMP –gated channels thus, decreases in Na influx. This causes the membrane potential to drop to -65mv, thereby hyperpolarizing receptor potential. Hyperpolarizing receptor potential reaches the synaptic terminal causing a decrease in the number of voltage- gated Ca+ channels opening therefore, less Ca+ entry into cell which decreases the release of neurotransmitter from the synaptic vessels. This turns on bipolar cell and allows the brain to recognize that there is light entering the eyeball. Bipolar cell Dim light partially turns off NT release; brighter light completely shut down NT release. Signal Processing The ganglion cells send action potentials along their axons, bundled together as the optic nerve which leaves the eye through the optic disc (the optic disc or blind spot where there are no photoreceptors). The optic nerve sorts out visual fields first at the optic chiasm, so that right visual field information from both eyes all go the left side of the brain. Some axons go to midbrain for proprioception, but most go to lateral geniculate body in thalamus, and then to occipital cortex where sensory information is topographically organized (similar idea to sensory homunculus) Visual Field Defects Visual field defects of Left eye Right eye Defect: 1. Blindness of 1 ipsilateral eye 2 2. Bitemporal 3 hemianopsia 3. Homonymous hemianopsia General Pathway of sound vibrations from outer ear to cerebral cortex. Pinna External auditory canal tympanic membrane malleus incus stapes oval window cochlea endolymph/perilymph and receptors in the organ of Corti cochlear branch of the vestibulocochlear nerve auditory cortex of temporal lobe Auditory and Vestibular Systems: Ear Anatomy Auditory and Vestibular Systems: Ear Anatomy The EAR has three regions: external ear, middle ear, inner ear. 1. External ear: Pinna-acts to funnel sound waves into opening in ear canal Ear canal = external auditory meatus- conducts sound waves, and secretes earwax (cerumen) to trap debris or small insects. Tympanic membrane = ear drum -vibrates when sound waves hit and since the tympanic membrane is connected to the malleus, it will cause the ossicles in middle ear to also vibrate. Auditory and Vestibular Systems: Ear Anatomy 2. Middle ear: small air filled cavity located between the tympanic membrane and the inner ear. Oval window- connected to stapes and therefore vibrates when tympanic membrane vibrates Round Window- opening in vestibular apparatus that dissipates energy wave back to middle ear Ossicles (malleus, incus, stapes ) are the small bones and are connected in such as way to amplify sound waves. The vibrations of the tympanic membrane are increased in amplitude as they move through the ossicles and causes even greater vibration of the oval window. 2 muscles: Protect inner ear from loud sounds. Tensor tympani muscle -attached to malleus, limits movement of tympanic membrane. Stapedius muscle – attached to the stapes and dampens the movement of the stapes. The eustachian tube (auditory tube) is normally collapsed, but will open while yawning, chewing or swallowing to equilibrate the pressure in the middle ear with the atmospheric pressure Auditory and Vestibular Systems: Ear Anatomy 3. Inner ear (bony labyrinth) : contains two divisions: 1) Bony labyrinth 2) Membranous labyrinth Bony Labyrinth includes cavities: a) Cochlea-contains receptors for hearing ; snail shaped b) Vestibule - contain receptors for equilibrium & balance and Contains two sacs: (i) Utricle (ii) Saccule c) Semicircular canals – contain receptors for equilibrium & balance and contain semicircular ducts. - Bony labyrinth includes perilymph (fluid); similar to CSF Membranous labyrinth - includes a series of sacs and tubes that have same shape as bony labyrinth; contains endolymph Anatomy of the Cochlea Uncoiled, the cochlea has three parallel, The Cochlea fluid-filled chambers: (i) Scala Vestibuli - Vestibular duct- ends in oval window contains perilymph. (ii) Cochlear duct – Scala Media - between vestibular and tympanic duct contains to endolymph(similar to intracellular fluid) (iii) Scala Tympani- Tympanic duct – ends in round window contains perilymph. Vestibular duct and Tympanic duct connected by helicotrema at tip of Cochlea Anatomy of the Cochlea Cochlear duct contains: The Organ of Corti (spiral organ) -sense organ for hearing Hair cell receptors with stereocilia and support cells Sits on basilar membrane Partially covered by tectorial membrane (gelatinous), which bends stereocilia (non-neural receptor cells) Cochlear branch of vestibulocochlear nerve (CNVII) at basal end of hair cell. Sound Waves are Generated from a Vibrating Object Sound Waves: are alternating high and low pressure areas originating from a vibrating object and travel through the air. Sound waves can be described as to their pitch, or tone determined by the frequency of the sound waves, and their intensity (loudness), which is determined by their amplitude (size). The average human can hear frequencies between 20-20,000 Hertz (Hz) or number of waves/ second. Loudness measured in decibels (dB). Sounds becomes uncomfortable at about 120dB and painful at 140dB. Transmission of Sound Waves through the Ear Steps in sound transduction through the ear: 1) The pinna directs sound waves into the external auditory canal 2) When sound waves strike the tympanic membrane, the alternating high and low pressure of air causes the tympanic membrane to vibrate. 3) The vibration is then transferred to the bones of the middle ear, malleus, incus and stapes. Vestibular duct 4) The stapes is attached to the membrane of the oval window and pushes oval window in and out. Tympanic duct 5) The movement of stapes at oval window creates pressure waves in the perilymph, of the vestibular Vestibular duct duct, of the cochlea. As oval window bulges inward it pushes on perilymph of the scala vestibuli. 6) Pressure waves are transmitted from scala vestibuli to scala tympani. 7) Pressure waves cause vestibular membrane moves back and forth, creating pressure waves in endolymph inside cochlear duct. 8) The pressure wave in endolymph cause the basilar membrane to vibrate, which moves the hair cells of the organ of Corti against the tectorial membrane. This leads to bending of the hair cell stereocilia, resulting in the production of receptor potentials that leads to the generation of action Sound Transduction via Inner Hair cells Inner hair cells transduce mechanical vibrations into electrical signals. Hair cells are embedded in the tectorial membrane and contain stereocilium. Stereocilia are attached to each other by tip links which are connected to mechanically- gated cation channels that open and close. Hair cells move in response to sound waves causing the stereocilia to flex (bend). (a) When hair cell is at rest, the stereocilia are erect and cation channels are partially open. A few K+ enter cell, causing a weak depolarizing receptor potential which cause a few Ca2+ voltage- gated channels to open and a small amount of Ca2+ to enter the cell and triggers exocytosis of a small amount of neurotransmitter from the synaptic Sound Transduction via Inner Hair cells (b) When vibrations of the basilar membrane causes stereocilia to bend toward the highest stereocilia , tip links pop open mechanically gated cation channels. Large K+ influx depolarizes the cell. More voltage-gated Ca2+ channels open, causing exocytosis of more NT within synaptic vesicles to be released into synaptic cleft. NT binds to receptors on primary sensory neuron and generates an increase in firing frequency of AP. (c) When vibrations of the basilar membrane causes stereocilia to bend away from the highest stereocilia, the tip links relax and all cation channels close. K +and Ca2+ influx slows, membrane hyperpolarizes, less NT released, and sensory neuron firing decreases. (**Note: The hair cells also move in similar Pitch and Loudness Discrimination Pitch discrimination is primarily a function of which portion of the basilar membrane is displaced. Low frequency waves ( low pitch) reaches the region near the helicotrema, whereas high frequency waves ( high pitch) causes the basilar membrane close to the oval window to vibrate. Loudness is primarily a function of intensity (rate) of action potentials, which in turn reflects the degree of bending of the stereocilia of the hair cells. Auditory Pathway Cochlea (release of NT from hair cells of the organ of Corti) transforms sound waves into electrical signals (AP) and the first order auditory (sensory) neurons transfer this information to the cochlear nuclei in the medulla (brain) via cochlear branch of the vestibulocochlear nerve (axons of first order auditory neurons). Second order sensory (auditory) neurons - send information to nuclei in the midbrain. Some axons decussate and synapse in the inferior colliculus. Some have two branches one on ipsilateral and one on contralateral side and synapse in superior olivary nucleus of the pons. Third order auditory (sensory) neurons – send information via one ipsilateral and one contralateral axon, from superior olivary nucleus to the inferior colliculus or axon from inferior colliculus, on contralateral side, sends information to the medial geniculate nucleus of thalamus. Fourth order auditory (sensory) neurons – send information via one ipsilateral and one contralateral axon from inferior colliculus to medial geniculate nucleus of thalamus. Axons on contralateral side, send information to primary auditory cortex. Auditory Pathway AP’s arriving at primary auditory cortex allows you to perceive sound. Input about pitch (frequency) from each portion of the basilar membrane is conveyed to a different part of the primary auditory cortex. Loudness and duration are also perceived at primary auditory cortex. Auditory information from primary auditory cortex is conveyed to auditory association area. This area stores auditory memories and compares present and past experiences, allowing you to recognize a particular sound as speech, music or noise. Auditory Association Area: Sound Recognition a are on c iati sso yA d it or A u Deafness: Hearing Loss Conductive deafness: No transmission through either external or middle ear to cochlea. Causes: Otosclerosis, wax plug, infection, trauma to ear drum Rx: reconstruction of bones in middle ear Sensorineural deafness: Impaired transmission along auditory pathway from cochlea to cerebral cortex causes: damage to hair cells of cochlea (loud noise - younger people), damage to Cochlear branch of CNVII, or areas that process auditory input. Presbycusis- sensorineural hearing loss in the elderly (damage or loss of hair cells or degeneration of nerve pathway) Rx: hearing aids, cochlear implants Central Damage to neural pathway between ear and cerebral cortex or damage to cortex itself Causes stroke Uncommon Equilibrium (balance) Vestibular Apparatus – are receptor organs for equilibrium. They include: 1. Otolith organs - convey information about your head and body position with respect to gravity or acceleration a) Utricle -detects (forward and back) linear acceleration & deceleration in horizontal direction. It also responds to forward and back head tilts. b) Saccule -detects (up and down (gravity)) linear acceleration & deceleration that occurs in vertical direction. 2. Semicircular canals (3) - convey information about rotational movements of the head. - Superior, posterior and horizontal canals filled with endolymph Both vestibular apparatus and Semicircular canals contain receptors (hair cells) for equilibrium and balance. Equilibrium projects primarily to the cerebellum Otolithic Organs Detect linear acceleration or deceleration and head tilt. The vestibular apparatus contains: 1) Otolith Organs called utricle and saccule contain a. Macula attached to inner walls of otolithic organs and contain 1) sensory receptors(hair cells) & 2) supporting cells for linear acceleration and head tilt. The hair cells have graduated stereocilia plus a kinocilium (longest stereocilia) connected by tip links. The stereocilia are embedded in a gelatinous otolithic membrane which have otolith crystals on its surface. When the head tips forward, the otolith crystals (calcium carbonate and protein particles) move by gravity “downhill”, causing the gelatinous material to move, and distorting the hair cells. This stimulates sensory neurons to send action potentials along their axons, bundled together into the vestibular branch of the vestibulocochlear nerve (CNVII). Otolithic Organs: Head Tilt Maculae of utricle and saccule are perpendicular to each other. When head is upright: macula of utricle oriented horizontally, macula of saccule oriented vertically. When the head tips forward, the otolith crystals (calcium carbonate and protein particles) move by gravity “downhill”, causing the gelatinous material to move, and distorting the hair cells. This stimulates sensory neurons to send action potentials along their axons, bundled together into the vestibular branch of the vestibulocochlear nerve (CNVII). When body is moved forward or backward; linear acceleration or deceleration, the otolith crystals in the utricle move forward or back causing the gelatinous material to move, and distorting the hair cells. This stimulates sensory neurons to send action potentials along their axons, bundled together into the vestibular branch of the vestibulocochlear nerve (CNVII). When the body is moved up or down; vertical acceleration or deceleration, the otolith crystals in the saccule move up or down causing the gelatinous material to move, and distorting the hair cells. This stimulates sensory neurons to send action potentials along their axons, bundled together into the vestibular branch of the vestibulocochlear nerve (CNVII). Equilibrium Transduction: Distortion of the hair cells Sound and equilibrium transduction are similar with respect to sensory receptors (hair cells) kino kin Semicircular Duct Detects rotational acceleration and deceleration in all directions. Semicircular ducts lie at right angles to each other. Three semi-circular ducts of the vestibular apparatus contain: Ampulla- dilated portion of each duct Endolymph contains Cristae which have Supporting Endolymph cells and Hair cells (sensory receptors) for rotational acceleration. The hair cells have graduated stereocilia plus a kinocilium (longest stereocilia) connected by tip links. Each crista is bound to a cupula, a gelatinous structure, that extends the full width of the ampulla. Semicircular Duct As the head turns, the fluid within the semi-circular canal (endolymph) moves, and drags against the gelatinous cupula and the hair cells are bent in the opposite direction of the head, which stimulates sensory neurons to send action potentials Endolym ph along their axons, bundled together into the vestibular branch of the vestibulocochlear nerve (CNVII). Vertigo – dizziness, associated with nausea due to infection, strokes, tumors, drugs or other inner ear disorders. Motion Sickness- dizziness, nausea, weakness, and malaise due to a conflict among senses regarding motion. Experienced in a car, airplane, train, or amusement park ride. Meniere’s disease- fluctuating hearing loss, tinnitus, dizziness, and nausea due to excess endolymph within vestibular apparatus. Cause unknown. Equilibrium Pathway Utricle, saccule, and semicircular ducts (release NT from hair cells) transform linear and rotational movement into electrical signals (AP’s) and the first order vestibular (sensory) neurons transfer this information to the vestibular nuclei of the medulla and pons; the remaining axons enter and synapse in the cerebellum. Bidirectional pathways interconnect the cerebellum and vestibular nuclei. Second order vestibular (sensory) neurons: send descending information via the vestibulospinal tract to skeletal muscles to help maintain equilibrium. Vestibular nuclei integrate information from vestibular, visual, and somatic receptors and then send commands to nuclei that control the movement of the eyes to help focus on visual fields as well as the thalamus. Third order vestibular (sensory) neurons: sends information from the thalamus to the vestibular cortex in parietal lobe to provide conscious awareness of the position and movement of the head.