Growth and Development I (Sebentinha) Lecture Notes PDF
Document Details
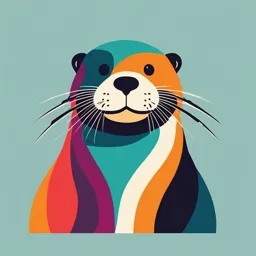
Uploaded by IngeniousSparrow594
UCP
2023
Marta Campos Ferreira de Brum Feijão
Tags
Summary
These lecture notes cover topics in growth and development, focusing on the molecular basis of cell growth, communication, and differentiation, along with principles of embryology. The notes are from academic year 2022/2023 and are designed for medical students.
Full Transcript
BLOCK 1.1 GROWTH AND DEVELOPMENT I Academic Year 2022/2023 Blockbook version Sebentinha vol.I - 13.0 st edition Marta Campos Ferreira de Brum Feijão October 2022...
BLOCK 1.1 GROWTH AND DEVELOPMENT I Academic Year 2022/2023 Blockbook version Sebentinha vol.I - 13.0 st edition Marta Campos Ferreira de Brum Feijão October 2022 000CODIGO000 SHAPING THE FUTURE OF MEDICAL EDUCATION 14/06/2021 0 FM.UCP.PT TABLE OF CONTENTS Lecture 2 - From DNA to proteins 1 1. Introduction 1 2. Nucleic acids (DNA and RNA) 1 2.1. The DNA molecule 1 2.2. the RNA molecule 2 3. DNA replication 3 4. Transcription 4 4.1. Steps 4 4.2. Silencer 5 4.3. TATA box 5 4.4. RNA polimerase 5 4.5. Transcription factors 6 5. RNA processing 7 5.1. Capping 7 5.2. Polyadenylation 7 5.3. Splicing 7 5.3.1. Alternative splicing 8 6. Exportation from the nucleus 8 7. Translation 9 7.1. Steps 9 7.1.1. Initiation process 9 7.1.2. Elongation process 10 7.1.3. Termination process 10 8. Transcription regulation 10 8.1. Regulation after transcription 11 8.2. microRNAs 11 8.3. RNA binding proteins 11 PBL 4 - How do cells communicate and develop? 12 1. Cell communication 12 2. Molecules involved in cell communication 12 2.1. Receptors and ligands 12 2.1.1. How different ligands and receptors lead to different responses 12 2.2. Target cells 16 II 1.1. Growth & Development - Block Notes 3. Outcomes of cell communication 17 4. Signals 18 4.1. Extra and intracellular signals and their outcomes 19 4.2. Signaling pathway stages: 19 Lecture 3 - a trip down the human cell 21 1. The cell structure 21 2. Plasma membrane 21 2.1. Structure and functional unit: the phospholipid bilayer 22 2.1.1. Phospholip phenomenons 23 2.2. Glycocalyx 24 2.2.1. Membrane proteins 24 2.3. Plasma membrane transport 25 2.3.1. Passive transport 25 2.3.2. Active transport 26 2.3.2.1. Channel proteins 26 2.3.2.2. ATP powered pumps 27 2.3.3. Endocytosis 27 2.3.4. Exocytosis 28 3. Cytoplasm & cytoskeleton 28 3.1. Cytoplasm 28 3.2. Cytoskeleton 28 3.3. Cell adhesions 29 4. Cell metabolism & Protein sorting 30 4.1. Endoplasmatic reticulum — smooth and rough 30 4.2. The signal hypothesis 30 4.3. Targeting proteins 31 4.4. Vesicular transport 31 4.4.1. Vesicular docking 33 4.5. The Golgi apparatus 34 4.5.1. Golgi apparatus — overview 34 4.5.2. Secretory pathways 34 4.5.2.1. Constitutive secretory pathway 34 4.5.2.2. Regulated secretory pathway 34 4.6. Lysosomes 36 4.7. Peroxisomes 36 5. Cell energetics 36 III 1.1. Growth & Development - Block Notes 5.1. The mitochondria 36 6. Cell regulation 37 6.1. Nucleus 37 6.2. Nucleolus 37 6.3. Nuclear transport 38 6.3.1. Nuclear import through the nuclear pore complex 38 6.3.2. Nuclear export 38 Lecture 5 - Gene mutations & Principles of tumor biology 40 1. Tumour vs cancer 40 1.1. Tumour (neoplasia) 40 1.2. Cancer 40 2. DNA damage 40 2.1. Types of DNA damage 40 2.1.1. Gene mutations 41 3. Carcinogenesis 41 3.1. Mutagens vs. carcinogens 42 3.1.1. Mutagens 42 3.1.2. Carcinogens 43 3.2. Multistep carcinogenesis 43 4. Cancer associated genes: oncogenes & tumour-suppressor genes 44 4.1. Oncogenes 44 4.2. Tumour-supressor genes 44 4.2.1. P53 gene 45 4.2.2. BRCA gene 45 4.3. The two-hit hypothesis (Knudson hypothesis) 46 Lecture 6- Hallmarks of cancer 47 1. A hallmark: framing the problem 47 2. The hallmarks of cancer: The new generation 47 3. Limitations 51 Lecture 7- Principles of embryology 52 1. Introduction 52 1.1. Clinical applications for the study of embryology 52 1.2. Human development timeline 52 2. Pregnancy tests 53 3. Gametes 54 3.1. Egg 54 IV 1.1. Growth & Development - Block Notes 3.1.1. Oogenesis 54 3.1. Spermatozoon 55 3.1.1. Spermatogenesis 55 4. Phases: from fertilisation to implantation 56 4.1. Fertilisation 56 4.2. Cleavage: division without growth 57 4.3. Compactation 57 4.4. Hatching 58 4.5. Implantation 58 4.6. Delamination 58 4.7. Gastrulation 59 4.7.1. Notochord 60 4.8. Neurolation 60 5. Cell potency 61 6. Tissues - Germ Cell Layers 62 6.1. Ectoderm 62 6.2. Mesoderm 62 6.3. Endoderm 63 Lecture 8- differentiation and patterning 64 1. Gene expression 64 1.1. Cell fate 64 1.2. Morphogens 65 2. Cell migration 65 3. Limb development 67 3.1. The organisation of the limb 67 3.2. Initiation of limb development 67 3.2.1. Hox genes 68 3.3. Further outgrowth of the upper limb 69 3.3.1. Proximal distal axis: role of AER- FGF 69 3.3.2. Cranial-caudal axis: role of ZPA-shh 71 3.3.3. Ventral-dorsal axis: role of Wnt-74 71 3.4. Cessation 72 4. Formation of digits 72 4.1. Intrinsic route of apoptosis 72 4.2. Extrinsic route of apoptosis 73 Lecture 9-Growth and Development 74 V 1.1. Growth & Development - Block Notes 1. Growth vs Development 74 2. Growth 74 2.1. Factors that influence growth 74 2.2. Stages of growth 75 2.2.1. Prenatal stage 75 2.2.2. Infancy stage 76 2.2.3. Childhood stage 76 2.2.4. Puberty stage 77 2.3. Growth charts and statistics 77 2.3.1. WHO growth charts – Multi-centre Growth Reference Study (2006) 78 2.4. Procedures for accurate measurements 79 3. Development 79 3.1. Models of development 80 3.1.1. Biopsychosocial models of development 80 3.1.2. Transactional model 81 4. Developmental theories 81 4.1. Psychoanalytic theories 81 4.2. Cognitive theories 82 4.3. Behavioural theory 82 4.4. Developmental domains 83 4.5. Developmental screening 84 4.5.1. Mary Sheridan’s scale 84 4.1. Socio-emotional development 87 4.1.1. Risk factors 88 4.1.2. Types of attachments 88 6. Puberty 89 6.1. Hormonal regulation 89 6.2. Physiology of the pubertal growth spurt 91 6.3. Puberty stages — Tanner scale 92 6.4. Physiological aspects of puberty 93 6.4.1. Menstrual cycle 93 6.4.2. Testosterone 97 6.4.3. Psychological aspects of puberty 98 Lecture 10 - Tissue repair and wound healing 100 1. Tissue repair 100 1.1. Tissue repair processes 100 VI 1.1. Growth & Development - Block Notes 2. Cell and tissue regeneration 101 2.1. Cell proliferation 101 2.1.1. Growth factors 102 3. Repair by connective tissue deposition (scar formation) 103 3.1. Angiogenesis 104 3.2. Deposition of connective tissue 104 3.3. Factors that influence tissue repair 106 4. Healing of skin wounds 106 4.1. Fibrosis in parenchymal organs 108 4.2. Defects in healing 108 4.2.1. Chronic wounds 108 4.2.2. Scarring defects 109 Lecture 11 - Biology of ageing 110 1. Ageing — definition and consequences to daily life 110 2. Theories of ageing 111 3. Hallmarks of ageing 112 VII 1.1. Growth & Development - Block Notes Sunday, 30 October 2022 Lecture 2 - From DNA to proteins 1. Introduction Gene is the segment of DNA that codes for a particular protein Transcription: DNA to RNA RNA processing : modification in the RNA Translation: RNA to protein 2 types of nucleic acids : desoxiribose, ribose (change according to the pentose) the big difference is the OH group (ribose has OH group at 2’, while the desoxiribose does not have anything) Heterocromatine: dense and inactive vs. Euchromatin: disperse and active 2. Nucleic acids (DNA and RNA) 2.1. The DNA molecule DNA is the blueprint for protein production and it is located inside each cell’s nucleus. It is organised into nucleosomes, that is, the DNA wrapped around 8 histone proteins and can be found in one of two states: - Heterochromatin — dense and in an inactive state — dark grey area - Euchromatin — disperse and in an active state —light grey area A Gene is a segment of the DNA that codes for a specific protein. The DNA molecule has a double helix structure and is made up of: a base (CUTPie);a phosphate group; and a pentose (either deoxydoribose or ribose). ‣ Complementary bases: A — T (2 hydrogen bonds); C — G (3 hydrogen bonds) ‣ Nucleotides: ↳ Pyrimidics : C, T (Single ring) ↳ Purines: A, G (double ring) 1 1.1. Growth & Development - Block Notes The DNA is a very organised molecule seeing as 2 strands coil around each other every 10 base points, this leads to the formation of major and minor groves which are larger or smaller spaces between the strands where proteins can bind to DNA in order to regulate its functions Central biology dogma - All cells express their genetic information this way, DNA-RNA-protein Telomeres - At the end of the lagging strand, enzyme telomerase extends the ends of the strand by adding multiple copies of the same short DNA sequence, thus preventing linear chromosomes from shortening during cell division. 2.2. the RNA molecule RNA stands for ribonucleic acid, and this name comes from the sugar that the molecule is made of, a ribose. Unlike the DNA molecule, RNA is a single-stranded molecule. The major difference between RNA and DNA is that, where in DNA you’d find a guanine, in RNA you can find a uracil. Types of RNA Messenger RNA Transfer RNA Ribosomal RNA mRNA tRNA rRNA Involved in protein Involved in protein Takes amonoacids to the synthesis. synthesis. ribosomes. It’s made from a DNA It serves as a link between Has a short RNA sequence. template during mRNA and the aminoacid It finds specific aminoacids. transcription. chain. Has a 3 letter coding Its main purpose is carrying Each time an aminoacid is sequence, corresponding to the information embedded in added to the chain, a a complementary sequence DNA to the cytoplasm so specific tRNA pairs with its in the mRNA chain — that it can be translated into complementary sequence anticodon a protein. on the mRNA molecule. 2 1.1. Growth & Development - Block Notes 3. DNA replication This replication is semi-conservative, that is because from a parent DNA single DNA strand another one arises by complementarity. Explicação interativa — https://www.labxchange.org/library/items/ lb:LabXchange:a21a9b48:lx_simulation:1 1. Initiation Pre replication complex seeks origin of replication, DNA helicases splits strands - Replication fork ‣ Single stranded DNA binding proteins improve stability of ione strands ‣ DNA topoisomerase prevents overwinding of later DNA 2. Elongation RNA primase creates multiple RNA primers → randomly binds → DNA polymerase adds complementary nucleotides in 3’ to 5’ direction ‣ Forms single leading strands ‣ Forms single lagging strand by attaching ( with DNA ligase multiple Okazaki fragments) 3. Termination DNA polymerase leaves strands at telomere (TTAAGGGG nucleotide sequences) Hayflick limit - maximum number of times a cell can be replicated ↳ Due to repeated shortening of telomeres during termination step 3 1.1. Growth & Development - Block Notes 4. Transcription It’s the first process in protein synthesis Happens in the nucleus 4.1. Steps The DNA sequence to which RNA polymerase binds to initiate transcription is called the promoter The process steps: 1. DNA unpacked from chromatin, undergoes dehelication 2. Promoter region identifies starting point for transcription 3. RNA polymerase shears hydrogen bonds between the 2 strands → transcription bubble 4. RNA polymerase follows template strand to assemble mRNA molecule (complementary strand to the template) 5. Hydrogen bonds form on nucleotides 6. Termination sequence contains two complementary sequences → mRNA binds within itself forming a hairpin loop 7. RNA polymerase detaches and DNA closes back up 4 1.1. Growth & Development - Block Notes 4.2. Silencer DNA sequence capable of binding transcription regulation factors, called the repressor. It induces a negative effect on the transcription of its particular gene. It can be located upstream, where it can help repress the transcription of the gene or some can be found downstream of a promoter located within the intron or exon of the gene itself. 4.3. TATA box This structure is a DNA sequence that indicates where a genetic sequence can be read or decoded. It is a type of promoter sequence, which specifies to other molecules where transcription begins. In fact, the TATA box is able to define the direction of transcription and also indicates the DNA strand to be read. The transcription factors can bind to the TATA box and recruit an enzyme called RNA polymerase. 4.4. RNA polimerase - The main enzyme responsible for RNA synthesis is RNA polymerase which catalyzes the polymerisation of ribonucleotide tryphosphates (NTPs) as directed by a DNA template - RNA polymerase does not require a preformed primer to initiate the synthesis of RNA. - RNA polymerase reads the template strand from 3’ to 5’ direction and the new strand is produced from 5’ to 3’ direction - Eukaryotic cells contain three distinct types of RNA polymerases is that transcribe different classes of genes ‣ RNA polymerase I is involved in transcription of mRNA ‣ RNA polymerase II also transcribes microRNA (miRNA), long noncoding RNA (lncRNA), there are critical regulators of gene expression ‣ tRNA and rRNA are transcribed by RNA polymerases I and III 5 1.1. Growth & Development - Block Notes 4.5. Transcription factors Many eukaryotic promotores contain a DNA sequence - TATA box Transcription factors are minimally required for eukaryotic RNA polymerase II to function 1. Binding of a general transcription factor called TFIID ‣ TFIID is composed of subunits such as the TATA binding proteins (TBP), and TBP-associated factors (TAFs) ‣ TBP is able to identify the TATA box so the whole structure of TFIID binds to it 2. Binding of TFIIB ‣ The binding of TFIID is followed by recruitment of a second general transcription factor 3. Binding of RNA polymerase II and TFIIF 4. Binding of TFIIE and TFIIH ‣ TFIIE and TFIIH assemble at the promoter, TFIIH plays two roles: ↳ Two subunits of TFIIH are helicases, which unwind DNA around the initiation site ↳ It phosphorylates RNA polymerase II, releasing the polymerase from most of the general transcription factors, so it can begin 6 1.1. Growth & Development - Block Notes transcription 5. RNA processing Before the mRNA is transported to the cytoplasm for protein synthesis it must be first modified 5.1. Capping Modifies the 5’ end of the RNA transcript (5’ end of RNA capped with a protective 7-methyl-guanine) Initiated by the addition of a methylated guanine nucleotide (G residue) The 5’ cap stabilizes the RNA 5.2. Polyadenylation Modifies the 3’ end of the RNA transcript 3’ end is trimmed by endonuclese, an enzyme that cuts the RNA chain at a particular sequence of nucleotides (AAUAAA) Another enzyme , Poly-A polymerase enzyme adds repeated adenine nucleotides (about 200) to cut the end 3’ end of RNA is topped with a protective poli - A tail (multiple adenines) 5.3. Splicing Removal of non coding sequences called introns Takes place in spliceosomes - complex composed of proteins and small nuclear RNAs Special nucletotide sequences in a pre-mRNA transcript signal the begging and the end of an intron The RNA portion of the snRNAs recognizes splice-site sequences through complemantary base pairing 7 1.1. Growth & Development - Block Notes The spliceosome brings the 5’ site close to the adenine nucleotide (the 3’ end), and the intron forms a lariat The lariat is cut and the intron is released and the snRNAs catalyze the linkage of the exon sequences 5.3.1. Alternative splicing This process allows for the production of various mRNAs and proteins from the same gene, this makes it an essential mechanism to increase the complexity of gene expression. Adicionally, it also plays an importante role in cellular differentiation and organism development. During this process, particular exons of a gene may be included within or excluded from the final, processed messenger RNA (mRNA) derived from said gene. This means the exons are joined in different combinations, leading to alternative mRNA strands. Consequently, the proteins translated from alternative spliced mRNA will contain differences in their amino acid sequence and, other, in their biological functions. ↳ Exon skipping: a particular exon may be included in mRNA under some conditions or in particular tissues and omitted from the mRNA in others 6. Exportation from the nucleus The selective transport into and out of the cell is highly selective and is mediated by nuclear pore complexes For the mRNA to leave the nucleus it must contain a set of RNA-binding proteins that signal a mature mRNA 8 1.1. Growth & Development - Block Notes The mRNA then passes through the nuclear pore complexes to be translated in the cytosol After translation the mRNA is degraded by nucletoides by ribonucleases (RNAses) present in the cytosol 7. Translation Translation takes place inside of structures known as ribosomes. Once a ribosome latches on to an mRNA and finds the "start" codon- AUG (metionin)- it will travel rapidly down the mRNA, one codon at a time. As it goes, it will gradually build a chain of amino acids that exactly mirrors the sequence of codons in the mRNA. As it turns out, this matching is not done by the ribosome itself. Instead, it depends on a group of specialized RNA molecules called transfer RNAS (tRNAs). Each tRNA has a three nucleotides sticking out at one end-to these threesomes we call anticodons-, which can recognize (base-pair with) just one or a few particular codons. At the other end, the tRNA carries an amino acid – specifically, the amino acid that matches those codons. There are many tRNAs floating around in a cell, but only a tRNA that matches the codon that's currently being read can bind and deliver its amino acid cargo. Once a tRNA is snugly bound to its matching codon in the ribosome, its amino acid will be added to the end of the 60s polypeptide chain. This process will cesse whenever the ribosome 40s reaches a stop codon-UAA, UAG, UGA An eukaryotic ribosome has 2 subunits : 40s and 60s 7.1. Steps 7.1.1. Initiation process Eukaryotic initiation factors bind the 40s ribosomal subunit and tRNA forming the pre-initiation complex 9 1.1. Growth & Development - Block Notes Initiation factors bind to the 5’ cap of the mRNA and the mRNA is brought to preinitiation complex The 40s ribosomal subunits scans the mRNA until it encounters an initiation codon (AUG sequence) 7.1.2. Elongation process Each ribosome has a binding site for mRNA and 3 binding sites for tRNA. The tRNA sites are designated the A,P and E sites The first tRNA carrying an amino acid enters the P site by base pairing with the complementary codon on the mRNA (step 1 in the picture). A second tRNA carrying an amino acid enters the A site and peptide bond is formed, the amino acids are transferred to the tRNA in the A site (step 2 in the picture). This means that a new aminoacid will always enter via the P site. The large ribosomal subunit shifts forward, moving the spent tRNA to the E site (step 3 in the picture) before ejecting it (step 4 in the picture) and a new tRNA joins on the A site (second step 1 in the picture). 7.1.3. Termination process The end of translation is signaled by stop codons in the mRNA (UAA,UAG,UGA). After that, proteins known as release factors bind to any stop codon that reaches the A site on the ribosome. The completed polypeptide is released, and the ribosome dissociates into its 2 separate subunits. 8. Transcription regulation In addition to the promoter, all genes have regulatory DNA sequences that are used to switch the gene on or off. Regulatory DNA sequences must be recognized by proteins called transcription regulators. It is the binding of a transcription regulator to regulatory DNA sequence that acts as the switch to control transcription 10 1.1. Growth & Development - Block Notes 8.1. Regulation after transcription After the mRNA has left the nucleus two key determinants of how much protein is made from it is its lifespan and how readily the translation machinery can attach to it Small regulatory RNAs are regulators that control mRNA lifespan and translation RNA processing is also part of regulation after transcription 8.2. microRNAs These pair up with a certain mRNA sequence to inhibit the expression of their targets and degrade it They also pair up with a protein that degrade the mRNA, meaning, whenever miRNA bind to mRNA that sequence won’t be translated 8.3. RNA binding proteins RBPs can bind to the mRNA to increase or decrease its stability depending of the region where it bonds enhancer → activator silencer → repressor 11 1.1. Growth & Development - Block Notes Lecture 3 - a trip down the human cell 1. The cell structure Plasma membrane: the boundary of the cell Nucleus: the centre of the cell. It includes the nuclear envelope + nuclear pores + nucleolus Cytoplasm: a gel-like substance surrounding the nucleous and packed with various organelles and molecules, each of which serve a specific function. 2. Plasma membrane The plasma membrane is the main responsible structure for separating the cell from its surroundings. It is involved in cell communication, import and export of molecules, and cell growth and motility. - Receptors in the plasma membrane enable the cell to receive signals from the environment; - Transport proteins in the plasma membrane enable the import and export of small molecules - The flexibility of the membrane and its capacity for expansion allow the cell to grow, change shape, and move. 21 1.1. Growth & Development - Block Notes Homeostatis: balance between the internal enviroment of the cell and the outside 2.1. Structure and functional unit: the phospholipid bilayer The cells membrane is organised in a lipid bilayer, where the main component of which is the phospholipid molecule. This is a amphipathic molecule, meaning it has a hydrophilic head and a hydrophobic tail. There are 5 major types of phospholipids: phosphatidylserine, phosphatidylethano- lamine, phosphatidylcholine (most abundant), sphingomyelin, sphingosine. - The outer part of the membrane consists mainly of phosphatidylcholine (C) and sphingomyelin (D), whereas phosphatidylethanolamine (A) and phosphatidylserine (B) are the predominant phospholipids of the inner part - The head group of phosphatidylserine is negatively charged, so its predominance in the inner leaflet results in a net negative charge on the cytosolic face of the plasma membrane 22 1.1. Growth & Development - Block Notes 2.1.1. Phospholip phenomenons Motility The way in which phospholipids are organised provides fluidity to cell membrane. It encompasses phenomenon like: lateral diffusion (phospholipids switch places), rotation or flip-flop (not as common). Fluidity The hydrocarbon tails of phospholipids can either be saturated (has no double bonds) or unsaturated (have one or more double bonds), those that are unsaturated produce a small kink in their tail making it more difficult for them to be packed together (Figure ). Thus, lipid bilayers that contain more unsaturated hydrocarbon tails are more fluid than those with less The fluidity of the membrane is also related to the cholesterol. Fig.-cholesterol’s location among phospholipid molecules This is because, it fill-in the spaces left by the kinks in the unsaturated hydrocarbons. Cholesterol tends to stiffen the bilayer, making it less flexible, as well as less permeable Membrane fluidity is important for many reasons, mainly because it enables many membrane proteins to diffuse rapidly in the plane of the bilayer and to interact with one another, as is crucial, for example, in cell signaling 23 1.1. Growth & Development - Block Notes 2.2. Glycocalyx All cells in the human body are covered by a dense layer of sugars and the proteins and lipids to which they are attached, collectively termed the “glycocalyx”. The glycocalyx is a central constituent of any cell, consisting of sugars and the proteins and lipids to which they are attached. Some of the glycolaxyx’s main functions: - Very important for cell recognition ‣ Carbohydrates or lipid attached to a protein - mechanism made for recognition (immune system to recognise cells) - Covers the outside of the membrane - Protect the cell from damage, participate in cell to cell interaction and adhesion - Important in determining blood group ABO (blood types depend on the glycoclayx of our blood cells) ‣ Example: antigens 2.2.1. Membrane proteins There are several types of proteins scattered throughout the membrane and their main function is to act in transport through the plasma membrane, helping substances that cannot transpass the bilayer by themselves get to the cytoplasm. These types of proteins are: ‣ Integral membrane proteins: inserted in the phospholipid bilayer ‣ Peripheral membrane proteins: not inserted into the hydrophobic interior of the lipid bilayer, they can be released from the membrane by more gentle extraction procedures Many integral proteins are transmembrane proteins: amphipathic, have their portions exposed on both sides of the membrane These proteins’ main functions are: - To act as markers (glycocalyx) ‣ Example: certain glycoproteins are cell surface markers - Attachment: integrin (cell to extracellular matrix) and cadherins (cell to cell) 24 1.1. Growth & Development - Block Notes - Transport ‣ Channel proteins: based on size ‣ ATP powered pumps: requires energy ‣ Carrier proteins: based on shape Size, shape, concentration, and ion - Receptors charge are factors that determine transport Size, shape, concentration, - Enzymes and ion charge are factors that determine transport 2.3. Plasma membrane transport (1) (2) (3) (4) 2.3.1. Passive transport Determined by gradient, either concentration or electrochemical - Simple diffusion (1): small non-polar particles cross the lipid bilayer (O2, CO2, hormones etc) 25 1.1. Growth & Development - Block Notes - Facilitated diffusion: use of membrane transport proteins for big molecules/ions - Channel mediated (2): form open pores through the membrane, allowing the free diffusion of any molecule of the appropriate size and charge - Transporter mediated (3): aka carrier proteins, they undergo conformational changes that allow the molecule to pass through the membrane and be released on the other side - Water moves across the membrane through aquaporins in the process of osmosis it goes from an area of low solute concentration to high 2.3.2. Active transport (4) Active transport requires the use of energy (namely ATP) since it takes molecules from a lower to a higher concentration. As the molecules go against concentration/ electrochemical gradient we can say they are moving in a non-physiological direction. - ATP-driven pumps: hydrolyse ATP to drive uphill transport - Coupled pumps: cotransporters, link the uphill transport of one solute across a membrane to the downhill transport of another - Light-driven pumps: found mainly in bacterial cells, use energy derived from sunlight to drive uphill transport 2.3.2.1. Channel proteins These channel proteins can function as uniporters or co-transporters in active or passive transport - Symporter: 2 molecules in the same direction - Antiporter: 2 molecules in opposite direction There are various types of channel proteins: - Voltage-gated channel: respond to 26 1.1. Growth & Development - Block Notes (2) membrane potential - Ligand-gated: extracellular ligand or intracellular ligand (3) - mechanically gated: conformational change of the transporter for it to open 2.3.2.2. ATP powered pumps These types of structures utilise energy derived directly from ATP hydrolysis to transport molecules. They come in different shapes and sizes: - P type pump - ABC transporter: important in drug usage ‣ Multi drug resistance bacteria MDR and cancerous cells are able to get the medication that is inside the cell out by using the ABC transporter (1) 2.3.3. Endocytosis Take up of macromolecules and particles Phagocytosis (1): engulfment of large particles. ‣ Membranes fuse to form a large intracellular vesicle called a phagosome. The phagosomes then fuse with lysosomes, producing phagolysosomes, in which the ingested material is digested. Pinocytosis (2): engulfments of fluids or small molecules. (3) 27 1.1. Growth & Development - Block Notes 2.3.4. Exocytosis Secretion of particles, the vesicles they are in fuse with plasma membrane in order to escape. 3. Cytoplasm & cytoskeleton 3.1. Cytoplasm The cytoplasm is the gelatinous liquid that fills the inside of a cell. It is composed of water, salts, and various organic molecules. Some intracellular organelles, such the nucleus and mitochondria, are enclosed by membranes that separate them from the cytoplasm. ↳ Cytoplasm= cytosol+ organelles ↳ Cytosol= fluid + cytoskeleton 3.2. Cytoskeleton Cells are highly structured, in fact they have a network of filaments known as the cytoskeleton (literally, “Cell skeleton”), which not only supports the plasma membrane and gives the cell an overall shape, but also aids in the correct positioning of organelles, provides tracks for the transport of vesicles, and (in many cell types) allows the cell to move. In eukaryotes, there are three types of protein fibres in the cytoskeleton: microfilaments, intermediate filaments, and microtubules. - Microtubules: important in cell to cell adhesion and extracellular proteins adhesion, formed of tubulin ‣ Actin filaments: aka microfilaments, thin flexible fibers, found on the polar side of the cell, helps forming the shape of microvilli (projections of the membrane; increase the surface area for absorption) ‣ Myosin: this is another cell protein that may form fibres. It is mostly involved in cell division, instance in which, a ring made of actin and myosin pinches the cell apart to generate two new daughter cells. ‣ Both of these filaments are of extremely high importance specially in muscle cells, since they are the ones responsible for the actual muscle contraction. 28 1.1. Growth & Development - Block Notes - Intermediate filaments: provide cells with mechanical strength, keratin - Microtubules: are the largest of the three types of cytoskeletal fibres, with a diameter of about 25 nm. A microtubule is made up of tubulin proteins arranged to form a hollow, straw-like tube, and each tubulin protein consists of two subunits, α-tubulin and β-tubulin. 3.3. Cell adhesions - Adherens junction: cell-cell adhesion through transmembrane proteins with active filaments called cadherins - Desmosomes: cell-cell adhesion which contacts are mediated by intermediate filaments related to cadherins - Hemi-desmosomes:cell connection to extracellular matrix though integrins and intermediate filaments - Gap junctions: direct connections between the cytoplasms of adjacent cells, are regulated channels through the plasma membrane that allows ions and small molecules to diffuse between neighbouring cells ‣ Consequently, gap junction couple both the metabolic activities and the electric responses of cells they connect - Tight junctions: separate the apical from basal part of the cell preventing free diffusion of lipids and membrane proteins between them; form seals that prevent the free passage of molecules (including ions) between the cells of the epithelial sheets Adherens junctions and desmosomes bind one epithelial cell to another, while hemidesmosomes bind epithelial cells to the basal lamina. 29 1.1. Growth & Development - Block Notes 4. Cell metabolism & Protein sorting 4.1. Endoplasmatic reticulum — smooth and rough Rough endoplasmic reticulum RER: has ribosomes that are actively synthesising proteins that are delivered into the ER membrane or into the ER interior, the lumen. Smooth endoplasmic reticulum SER: lacks ribosomes, site of lipid synthesis. In the Golgi apparatus we give function to the proteins (where they will go and how they will act) and then are allocated for where they are needed 4.2. The signal hypothesis - Co translation: changes in the proteins that change why translation happens - Post translation: changes in the protein are after translation happen A simplified view of protein translocation across the er membrane, as originally proposed. When the er signal sequence emerges from the ribosome, it directs the ribosome to a translocator on the er membrane that forms a pore in the membrane through which the polypeptide is translocated. a signal peptidase 30 1.1. Growth & Development - Block Notes is closely associated with the translocator and clips off the signal sequence during translation, and the mature protein is released into the lumen of the er immediately after its synthesis is completed. The translocator is closed until the ribosome has bound, so that the permeability barrier of the er membrane is maintained at all times. 4.3. Targeting proteins These are signal sequences direct proteins to the correct destination. Proteins can be translocated into the ER either during their synthesis (cotranslational translocation) or after their translation has been completed on free ribosomes (post- translational translocation) On one hand, proteins destined for the ER, Golgi apparatus, lysosomes, the plasma membrane, and secretion from the cell are synthesised on ER membrane-bound ribosomes. On the other hand, proteins destined to remain in the cytosol or to be incorporated into mitochondria, chloroplasts, or nucleus are synthesised on free ribosomes and released into the cytosol when translation is complete. As translation proceeds, the polypeptide chains are transported into the ER where protein folding and processing take place. From the ER, proteins are transported in vesicles to the Golgi apparatus where they are further processed and sorted for transport to lysosomes, the plasma membrane, or secretion from the cell. 4.4. Vesicular transport Each transport vesicle that buds off from a compartment must take with it only the proteins appropriate to its destination and must fuse only with the appropriate target membrane Vesicles that bud from membranes usually have a distinctive protein coat on the cytosolic surface and are therefore called coated vesicles ↳ This protein coat is made from clathrin Coated proteins These coated proteins are an esemble of proteins that come along the vesicles, more specifically along their outer membrane. 31 1.1. Growth & Development - Block Notes - Vesicles that go between the golgi and the plasma membrane are coated with clathrin protein - Vesicles that are coated with COPII move from the ER to Golgi - COPI marks the vesicles going for the inside the layers of the Golgi and from the cis end of the golgi to the ER - Vesicles coated with clathrin are going from the Golgi to the plasma cell membrane Cargo receptors, with their bound cargo molecules, are captured by adaptins, which also bind clathrin molecules to the cytosolic surface of the budding vesicle Clathrin and dynamic proteins help in shaping the vesicle and pinching it off After budding is complete, the coat proteins are removed, and the naked vesicle can fuse with its target membrane 32 1.1. Growth & Development - Block Notes RAB proteins: responsible for the transport of the vesicle trafficinkg (post man - you need a package officer that send the mail) - specific for each vesicle ↳ Tethering - transport of the vesicle ↳ Docking ↳ Fusion 4.4.1. Vesicular docking Once a transport vesicle had reached its target, it must recognise and dock with its specific organelle, this is possible because the transport vesicle in the cell displays molecular markers on its surface that identify the vesicle according to its origin and cargo. In fact, this identification depends on RAB proteins and SNAREs on the surface of the vesicle and target. - VSNARE on the surface of vesicle SNARES interact by firmly docking the vesicle in place - TSNARE on the target membrane Rab proteins, tethering proteins and SNAREs help direct transport vesicle to their target membranes A v-SNARE on the vesicle then binds to a complementary t-SNARE on the target membrane (like an anchor) Rab and tethering proteins provide the initial recognition between a vesicle and its target membrane, but complementary SNARE proteins ensure that transports vesicles dock at their appropriate target membranes 33 1.1. Growth & Development - Block Notes 4.5. The Golgi apparatus 4.5.1. Golgi apparatus — overview The golgi has 2 distinct faces: an entry face or cis and an exit face or trans. The cis face is adjacent to the ER while the trans face point towards the plasma membrane. Vesicle buds from the trans golgi network and fuses with the membrane in the process of exocytosis. 4.5.2. Secretory pathways 4.5.2.1. Constitutive secretory pathway All our cells have this secretory pathway. The constitutive exocytosis pathway supplies the plasma membrane with newly made lipids and proteins, enabling the plasma membrane to expand prior to cell division. The constitutive pathway also carries soluble proteins to the cell surface to be released to the outside, a process called secretion. 4.5.2.2. Regulated secretory pathway 34 1.1. Growth & Development - Block Notes A molecule that goes to the plasma cell, will go to the receptor and it will be fused (has to have an external stimulus to activate it). Operates only in cells that are specialised for secretion, ex: endocrine glands. Each specialised secretory cell produces large quantities of a particular product - such as a hormone, mucus, or digestive enzymes which is stored in secretory vesicles. 35 1.1. Growth & Development - Block Notes 4.6. Lysosomes Responsible for digestion in our cells they have to have a lot of enzymes (ases- enzymes that will destroy something). - If a lysosome leaks within the cell it will destroy the organelles so we put these enzymes to function on a different PH as of the cytoplasm (enzymes within the lysosomes - acid PH). As the surrounding PH is not acid they will not be activated - PROTECTION OF THE CELL BECAUSE OF THE LYSOSOMES Membranous sacs of hydrolytic enzymes that carry out the intracellular digestion of both extracellular material and worn out organelles. They contain about 40 types of enzymes, including those that degrade proteins, nucleic acids, oligosaccharides and lipids. These enzymes are optimally active in acidic conditions (ph~5); they have H+ pumps in their membrane. 4.7. Peroxisomes This are vesicle/container cells that participate in oxidation reactions. The formation of hydrogen peroxide occurs. They don't have DNA, only proteins, but they can replicate themselves. The content of the peroxisome is produced by the nucleus and ribosomes so the only thing it has to do by itself is division / fission. 5. Cell energetics 5.1. The mitochondria ATP (adenosine triphosphate) is the energy currency of the cell, and is produced in a process known as cellular respiration. Though the process begins in the cytoplasm, the bulk of the energy produced comes from later steps that take place in the mitochondria. There are actually two lipid bilayers that separate the mitochondrial contents from the cytoplasm. We refer to them as the inner and outer mitochondrial membranes. If we cross both membranes we end up in the matrix, where pyruvate is sent after it is created from the breakdown of glucose (this is step 1 of cellular respiration, known as glycolysis). Mitochondria are also somewhat unique in that they are self-replicating and have their own DNA, almost as if they were a completely separate cell. The prevailing 36 1.1. Growth & Development - Block Notes theory, known as the endosymbiotic theory, is that eukaryotes were first formed by large prokaryotic cells engulfing smaller cells that looked a lot like mitochondria. Instead of being digested, the engulfed cells remained intact and the arrangement turned out to be advantageous to both cells, which created a symbiotic relationship. 6. Cell regulation 6.1. Nucleus Our DNA has the blueprints for every protein in our body, all packaged into a neat double helix. The processes to transform DNA into proteins are known as transcription and translation, and happen in different compartments within the cell. The first step, transcription, happens in the nucleus, which holds our DNA. A membrane called the nuclear envelope surrounds the nucleus, and its job is to create a room within the cell to both protect the genetic information and to house all the molecules that are involved in processing and protecting that info. This membrane is actually a set of two lipid bilayers, so there are four sheets of lipids separating the inside of the nucleus from the cytoplasm. The space between the two bilayers is known as the perinuclear space. Though part of the function of the nucleus is to separate the DNA from the rest of the cell, molecules must still be able to move in and out (e.g., RNA). Proteins channels known as nuclear pores form holes in the nuclear envelope. The nucleus itself is filled with liquid (called nucleoplasm) and is similar in structure and function to cytoplasm. It is here within the nucleoplasm where chromosomes are found. The nuclear envelope also keeps molecules responsible for DNA transcription and repair close to the DNA itself - otherwise those molecules would diffuse across the entire cell. While transcription is completed within the nucleus, translation takes place in the cytoplasm. If there was no barrier between the transcription and translation machineries, it’s possible that poorly made or unfinished RNA would get turned into poorly made and potentially dangerous proteins. Before an RNA can exit the nucleus to be translated, it must get special modifications, in the form of a cap and tail at either end of the molecule, that act as a stamp of approval to let the cell know this piece of RNA is complete and properly made. 6.2. Nucleolus Within the nucleus is a small subspace known as the nucleolus. It is not bound by a membrane, so it is not an organelle. This space forms ribosomes which then exit the nucleus through the nuclear pores. 37 1.1. Growth & Development - Block Notes 6.3. Nuclear transport Proteins with a nuclear localisation signal are recognised by nuclear transport receptors called importins, because they carry proteins into the nucleus. The importins work in conjunction with Ran protein, a GTP binding protein, which controls the directionality of movement through the nuclear pore. Proteins are targeted for export from the nucleus by specific amino acid sequences, called nuclear export signals, that are recognised by receptors within the nucleus— exportins, which direct protein transport through the nuclear pore complex to the cytoplasm Ran proteins Ran GTPase activating proteins (Ran GAP): on the side of the cytoplasm Ran guanine nucleotide exchange factor (Ran GEP): phosphorylates Ran and can only exit the nucleus with 3 sets of phosphates, and it comes in with 2 sets of phosphates (dephosphorylation of Ran GTP) 6.3.1. Nuclear import through the nuclear pore complex Transport begins when the sequence of a cargo protein is recognised by an importin. Then, the cargo/importin complex binds to nuclear pore proteins and is transported through the pore. Lastly, at the nuclear side of the envelope, Ran/GTP binds to the importin, disrupting the cargo/importin complex and releasing the cargo protein into the nucleus. 6.3.2. Nuclear export Firstly, complexes between cargo proteins bearing nuclear export signals, exportins, and Ran/GTP form in the nucleus. 38 1.1. Growth & Development - Block Notes After that, following transport through the nuclear pore complex, Ran GAP is formed into Ran/GDP causing the release of the cargo protein and exportin into the cytoplasm. Ran/GDP and the exportin are then returned to the nucleus. Ran/GTP is regenerated for a future nuclear import. 39 1.1. Growth & Development - Block Notes Lecture 5 - Gene mutations & Principles of tumor biology 1. Tumour vs cancer 1.1. Tumour (neoplasia) According to the US Nacional Cancer Institute, a tumour is an abnormal mass of tissue that forms when cells grow and divide more than they should or do not die when they should. These tumours may be benign (not cancer) or malignant (cancer). Benign tumours may grow large but do not spread into, or invade, nearby tissues or other parts of the body, unlike malignant tumours do. 1.2. Cancer When we talk about cancer, we are referring to a situation in which abnormal cells divide without control and can spread/invade nearby tissues. Therefore we are only referring to malignant tumours. Cancer cells can also spread to other parts of the body (metastases) through the blood and lymph systems. 2. DNA damage 2.1. Types of DNA damage DNA damage can occur in the form of: - Gene mutations - Gene amplifications - Chromosomal abnormalities (mutations) - Epigenetic mechanisms - Changes in mRNA and non-coding RNA DNA damage can occur in coding and non-coding (promoters, enhancers, and silencers) areas of the genome. Genetic changes → altered proteins (or no protein at all) → altered cell function 40 1.1. Growth & Development - Block Notes 2.1.1. Gene mutations Gene mutations Chromosomal mutation Gene mutation (Large scale) (Point mutation/small scale) Structural Numerical Structural Monosomy Deletion Substitution Trisomy Duplication Deletion (frame shift) Tetrasomy Inversion Insertion (frame shift) Translocation early stop codon Isochromosome Non-missense Missense synthesis of different AA These mutations may, lead to a loss or gain in fuction (enhanced activation). Dominant negative mutations: new gene product acts antagonistically to the wild- type allele (e.g., P53 mutations). 3. Carcinogenesis Also know as oncogenesis or tumorogenesis, this process reffers to the transformation of normal human cells into malignant derivates and progression of a malignant tumour. Cancer is a genetic disease (genetic defects/mutations) ̶ Not necessarily, but most frequently is not hereditary (somatic vs. germ line cells). However, unlike other genetic diseases there is not a single gene defect causing cancer. This is a complex process that requires time and also takes a sequence of steps — multistep carcinogenesis. 41 1.1. Growth & Development - Block Notes This process sort of resembles Darwinian evolution in the idea that a succession of genetic changes, each conferring one or another type of growth advantage, lead to the transformation of normal human cells into cancerous cells. Important to note that, most mutations are deleterious (meaning ‘to cause harm’) events to cancer cells and that in order for a malignant neoplasia to arise critical genes need to be affected (cancer-associated genes). 3.1. Mutagens vs. carcinogens A mutagen is a chemical agent or physical event that can cause gene mutations (permanently change genetic material). A carcinogen however, is any substance or physical event that promotes carcinogenesis/cancer. From this information, we derive that: - mutagens are carcinogens (as cancer is linked to mutations) - some carcinogens are mutagens (e.g. tobacco smoke) while others are non- genotoxic (e.g. increase cell division rate, as estrogen or TPA) In the past, some professions were thought to be related to cancer, we now know that it is occupational mutagens that are at stake. A few examples are: ‣ Asbestos (e.g., construction workers): malignant mesothelioma ‣ Benzene (e.g., oil refineries): leukaemia ‣ Radiation (e.g., radiology facilities): leukaemia, thyroid cancer (…) 3.1.1. Mutagens These can be endogenous, meaning they are reactive oxygen species (ROS) — highly reactive chemicals formed from O2 (peroxide and superoxide). ↳ Cause DNA strand breaks; generate DNA-DNA or DNA protein linking ↳ Byproduct of normal mitochondria O2 metabolism (oxidative phosphorylation) However, they can also be exogenous, meaning they have an outside cause. ↳ Radiation - UV / X-Rays ↳ Chemicals - Carcinogens (cigarettes), Processed foods and preservatives, Cosmetic and chemical products 42 1.1. Growth & Development - Block Notes ↳ Infectious agents - Viruses (HPV), Bacteria (Heliobacter) 3.1.2. Carcinogens Carcinogens may: - Damage genome (e.g. radiation)→mutagen - Disrupt metabolic processes (e.g. TPA)→non-mutagen Carcinogens are not necessarily immediately toxic. - UV radiation leads to insidious DNA damage and increases risk for melanoma Carcinogens facilitate/promote the multistep process of carcinogenesis: initiation, promotion and progression. 3.2. Multistep carcinogenesis Upon pathological analyses of organ sites, these reveal lesions that seem to depict intermediate steps in a process of evolution from normalcy into invasive cancers. The skin model for carcinogenesis. 43 1.1. Growth & Development - Block Notes 4. Cancer associated genes: oncogenes & tumour-suppressor genes 4.1. Oncogenes To positive regulators in normal cell growth we call proto-oncogenes. Whenever these get altered in ways that make them. - Constitutively active - More active than usual (active under conditions where the wild-type gene isn’t) They get stuck in a state analogous to a stuck accelerator in an automobile: the car still moves forward even when the driver removes his foot from it. Dominant effect: activating one allele is generally sufficient to confer selective growth advantage to the cell Examples: - Receptor tyrosine kinases (EGFR) - Cytoplasmic tyrosine kinases (Abl) - Regulatory GTPases (ras) - Cytoplasmic serine/threonine kinases (Raf) - Transcription factors (c-myc, c-fos, c-jun) 4.2. Tumour-supressor genes These are genes involved in regulating cell growth (cell cycle and apoptosis), DNA repair and external proliferative signals. Tumour suppressor genes are altered to be inactive or less active. This mechanism is analogous to a dysfunctional brake in an automobile: the car does not stop even when the driver presses the brake. They have a recessive effect, this means that the deactivation of the two alleles is generally required to confer selective growth advantage to the cell. A few examples can be examples: - cell cycle checkpoint-control proteins (pRb, BRCA1) - proteins controlling apoptosis (p53) - DNA repair genes (MSH2) 44 1.1. Growth & Development - Block Notes 4.2.1. P53 gene The p53 tumour suppressor gene is the most common target of mutations in human cancers. It regulates both cell cycle progression and apoptosis. In the case of DNA damage occurring p53 is rapidly induced, which activates transcription of both cell cycle inhibitory and proapoptotic genes. p53 activates p21 protein which blocks cell cycle progression in G1, allowing for the DNA to be repaired and preventing the proliferation of a damaged cell. 4.2.2. BRCA gene BRCA genes appear to be involved in checkpoint control of cell cycle progression and repair of double-strand breaks in DNA. BRCA1 and BRCA2 thus function as stability genes, which act to maintain the integrity of the genome. Clinical case: pRb is a tumour suppressor protein: inhibits the cell cycle progression from G1 to S phases until a cell is ready to divide (cell cycle checkpoint); Loss of heterozygosity LOH: a heterozygous cell become homozygous because of a mutation that causes for the allele to be lost; New cell is more likely to show malignant growth if the altered gene is a tumour suppressor gene; Inheritance of a mutant copy of Rb leads to the development of retinoblastoma (intra- ocular tumour); The significance of the Rb tumour suppressor gene thus extends beyond retinoblastoma, and mutations of the Rb gene contribute to development of a substantial fraction of human cancers 45 1.1. Growth & Development - Block Notes 4.3. The two-hit hypothesis (Knudson hypothesis) This theory states that most tumour suppressor genes require both alleles to be inactivated (mutations or epigenetic silencing) to cause cancer. In practical terms, for an individual to have a fully silenced tumour suppressor gene, they would have to get “hit” twice in their genes. In the case we get from our parents one gene that is partially working, this is our “first hit”, therefore, if something else acts upon our genome, that would be our “second hit”, meaning it would definitely cause cancer. 46 1.1. Growth & Development - Block Notes Lecture 6- Hallmarks of cancer 1. A hallmark: framing the problem By definition, a hallmark is a principle, a characteristic that makes something distinctive. And in this case, specifically, it is no more than the rationalisation of the complexity underlying cancer diversity and biology. It proposes a hypothesis— that the various cancers share common features, basic rules that govern the transformation of normal human cells into malignant The hallmarks of cancer framework’s major impact would be to support cancer research in understanding how to counteract mechanisms that allow cancers to kill patients. In reality, cancer cells must overcome many built-in barriers (checks and balances) aiming to prevent cancer inception, development and progression. So, in a way we can look at these hallmarks as the set of capabilities that collectively enable cancer (“the outlaw organ”). Balance between apoptosis and cell proliferation 2. The hallmarks of cancer: The new generation Cancer is a manifestation of these 10 alterations: 1. Self-sufficiency in cell signals It was one of the first hallmarks to be defined since it has a very high prevalence of dominant oncogenes that have been found to modulate it. Tumour cells are able to produce their own GF (acquired GS autonomy/autocrine simulation) by an over-activation of the Ras gene ‣ e.g.: glioblastomas and sarcomas produce PDGF (platelet-driven GF) and TGF⍺ (tumour GF⍺ ) Cancer cells are able to grow in the absence of growth factors due to the abnormalities in intracellular signalling systems, such as unregulated activity of 47 1.1. Growth & Development - Block Notes growth factor receptors or other proteins (e.g., Ras proteins or protein kinases) → this can also make them hypersensitive to stimuli 2. Evading growth suppressors Growth Factors negatively regulate cell proliferation, meaning they stop them from overdoing it Tumour suppressor genes become inactivated (p53, RB1 and TGFβ) - Normally cells are arrested in a G0 phase until DNA is repaired → p53 - RB1 blocks ells between G1 and S phase by regulating the function of cyclin dependent kinases (CDK) → RB1 - TGFβ tops cell cycle at G1 — many cancers have mutated proteins along the signal cascade, preventing action Antigrowth signals act on cells either by forcing them into the G0 stage or to permanently stop proliferating Disruption of the pRb pathway liberates E2Fs and thus allows cell proliferation, rendering cells insensitive to antigrowth factors 48 1.1. Growth & Development - Block Notes - E2F stimulates expression of its target genes, which encode proteins required for cell cycle progression 3. Avoiding immune destruction Majority of small tumours are destroyed by the immune system Large solid tumours avoid detection — evade destruction Greater incidence in immunocompromised or immunodepressed individuals (HIV, tumour viruses — HIV is NOT) Highly immunogenic cancer cell clones are routinely eliminated in immunocompetent hosts (a process referred to as ‘‘immunoediting’’) leaving behind weakly immunogenic variants to grow and generate tumors Such weakly immunogenic cells can thereafter colonize both immunodeficient and immunocompetent hosts 4. Tissue invasion and metastasis Cancer cells have a reduced expression of adhesion proteins such as cadherins, hence their connection to the extracellular matrix is reduced, contributing for their metastasis Malignant cells generally secrete proteases that digest extracellular matrix components, penetrating through the basal lamina, invading underlying connective tissue and adjacent normal tissues. 5. Limitless replicative potential Overcome the shortage of telomere barrier by reactivating the production of telomerase enzyme, enabling them to maintain telomere length indefinitely ‣ Telomere: repeat of TTAGGG at the end of the DNA strand 49 1.1. Growth & Development - Block Notes They don’t reach senescence (phenomenon characterised by the cessation of cell division) ‣ senescence can be circumvented by disabling their pRb and p53 tumour suppressor proteins, enabling these cells to continue multiplying for additional generations 6. Tumour promoting inflamation Inflammation supplies bioactive molecules to the tumour microenvironment, GF that sustain proliferative signalling, survival factors that limit cell death, proangiogenic factors, extracellular matrix-modifying enzymes that facilitate angiogenesis, invasion, and metastasis 7. Sustained angiogenesis Cells inside the tumour are deprived of an adequate supply of oxygen and express vascular endothelial growth factors VEGF - VEGF is over-expressed → blood vessels are formed → otherwise tumours could only grow up until 1 or 2 mm Blood vessels are formed in response to VEGF and basic fibroblast growth factor bFGF, secreted by the tumour cells, that stimulate proliferation of endothelial cells in the walls of capillaries in surrounding tissue ‣ Important in metastasis 50 1.1. Growth & Development - Block Notes ‣ The growing new capillaries formed are easily penetrated by the tumour cells, providing an opportunity for cancer cells to enter the circulatory system and begin the metastatic process. 8. Genome instability and mutation Most cancer cells are genetically unstable, with a greatly increased mutation rate and an abnormal number of chromosomes Mutation of P53 (controls cell cycle) will no longer activate p21 to block cell growth 9. Evasion of programmed cell death — apoptosis They can survive levels of stress that would otherwise kill normal cells by apoptosis 50% of all human cancers have an inactivating mutation in the p53 gene, protein that normally acts as part of a DNA damage response that causes cells with DNA damage to either cease dividing or die by apoptosis 10. Deregulating cellular energetics They limit their energy metabolism largely to glycolysis, and anaerobic respiration (production of lactate which then will form amino acid precursors) ‣ To compensate for the lower efficiency of ATP production afforded by glycolysis, cancer cells express more glucose transporters GLUT1, which substantially increases glucose import into the cytoplasm Tumour cells use the less effective way of producing ATP, however they can grow in hypoxia (anaerobic environment). 3. Limitations Benign tumours may present many of these hallmarks too, expect for the ability to invade and metastasize. Also, cancer is a tissue level /systemic disease and these hallmarks focus too much on cellular level features. Interaction with neighbouring cells and the immune system — tumour micro environment. 51 1.1. Growth & Development - Block Notes Lecture 7- Principles of embryology 1. Introduction 1.1. Clinical applications for the study of embryology Some clinical applications for studying embryology are, for example: - Chromosomic/genetic abnormalities - IVF - Ageing - Therapeutics (cancer) - Interplay with oncology, genetics, evolution, anatomy, physiology 1.2. Human development timeline The pregnancy or gestational age is measure backwards from the last menstrual period (LMP), since that’s what women normally know more accurately. To actually calculate the fertilisation date or embryo/foetus age we count 14 days after menstruation, since that is when ovulation takes place. Knowing this, we can conclude that conception took place up to 24h post ovulation. Embryogenesis (from conception to birth) - Embryo (8/10 weeks — 90% adult structures) - Foetus (until approx. 40 weeks) Human pregnancy -9 months First trimester Second trimester Third trimester Embryonic Foetal Menstruation Menstrual cycle Menstrual cycle Menstrual cycle Embryonic period (stages 1 to 23) Foetal Fertilisation LMP Week 1 to 4 Week 5 to 8 -2 -1 0 1 2 3 4 5 6 7 8 52 1.1. Growth & Development - Block Notes (until approx. 40 weeks) 2. Pregnancy tests These types of tests detect the presence of hCG (human chorionic gonadotropin) hormone in urine or blood samples. Between 6-12 days after ovulation, the developing placenta starts to release hCG in the blood. In the begging these levels rise rapidly, doubling every 2-3 days, they peak between 8-11 weeks of the pregnancy and slowly decrease after birth The most common pregnancy test that we can get in a pharmacy, is called a qualitative hCG test and checks hCG presence (10 days after a missed menstruation). ‣ Positive: 2 distinct lines appear (positive and control line) ‣ Negative: one line control ‣ Invalid: control line fails to appear Quantitive hCG testing also exists and it measures the exact amount of hCG in blood, making it a very sensitive test, however these are not procedural. 53 1.1. Growth & Development - Block Notes ↳ Can track eventual problems during pregnancy, like miscarriages, chromosomic abnormalities or the number of pregnancies Human Chorionic Gonadotropin (hCG): hormone produced by synciotrophlast to keep corpus luteum, thus maintaining high progesterone levels (pregnancy maintenance/uterus mucosa) 3. Gametes The gametes are highly specialised cells, involved in reproduction, they are eggs, in women, and spermatozoa, in men. 3.1. Egg These are non motile cells, that are formed by the zona pellucida, cortical granule, nucleus and corona radiata 3.1.1. Oogenesis Oogenesis occurs in the outermost layers of the ovaries. As with sperm production, oogenesis starts with a germ cell, called an oogonium (plural: oogonia), but this cell undergoes mitosis to increase in number, eventually resulting in up to one to two million cells in the embryo. The cell starting meiosis is called a primary oocyte. This cell will begin the first meiotic division, but be arrested in its progress in the first prophase stage. At the time of birth, all future eggs are in the prophase stage. At adolescence, the primary oocyte finishes the first meiotic division. The cell divides unequally, with most of the cellular material and organelles going to one cell, called a secondary oocyte, and only one set of chromosomes and a small amount of cytoplasm going to the polar body. A secondary meiotic arrest occurs, this time at the metaphase II stage. At ovulation, this secondary oocyte will be released and travel toward the uterus through the oviduct. If the secondary oocyte is fertilised, the cell continues 54 1.1. Growth & Development - Block Notes through the meiosis II, completing meiosis, producing a second polar body and a fertilised egg containing all 46 chromosomes of a human being, half of them coming from the sperm. What is a polar body? A cell that is formed at the same time as an egg cell during oogenesis, but generally doesn’t have the ability to be fertilised 3.1. Spermatozoon This, unlike the egg, is a motile cell and has a head (acrosome, centriole, nucleus)-the acrosome protects the head of the sperm— a mid-piece (mitochondria) and, a flagellum (made of tubulin). 3.1.1. Spermatogenesis Spermatogenesis occurs in the wall of the seminiferous tubules, with stem cells at the periphery of the tube and the spermatozoa at the lumen of the tube. Immediately under the capsule of the tubule are diploid, undifferentiated cells. These stem cells, called spermatogonia (singular: spermatogonium), go through mitosis with one offspring going on to differentiate into a sperm cell, while the other gives rise to the next generation of sperm. Meiosis begins with a cell called a primary spermatocyte. At the end of the first meiotic division, a haploid cell is produced called a secondary spermatocyte. This haploid cell must go through another meiotic cell division. The cell produced at the end of meiosis is called a spermatid. When it reaches the lumen of the tubule and grows a flagellum (or “tail”), it is called a sperm cell. Four sperm result from each primary spermatocyte that goes through meiosis. 55 1.1. Growth & Development - Block Notes 4. Phases: from fertilisation to implantation 4.1. Fertilisation - Occurs in the ampullary part of the fallopian tube - 16-20 hours after fertilisation we obtain the zygote - Sperm must go through 2 layers. Cumulus layer and zona pellucida (A) and (B) 1. Contact of sperm and egg (C) 2. Acrosome reaction: the sperm lyses both layer with digestive enzymes present in the acrosome (D) 3. Recognition of surface proteins of the egg (E) 4. Fusion of sperm and egg: the sperm passes through the egg membrane breaking through integrin proteins (F) 5. The first sperm to arrive induces reaction closing sodium channels in the egg, the charge inside becomes positive preventing any future egg from fusing 6. The influx of calcium to the egg by the sperm induces the reaction of granules in the egg, creating an envelope further blocking the cell from accepting any system - Oocytes are arrested at meiosis I, and when a sperm fertilises the meiosis process continues. - The release of Ca2+ (stored in cytoplasmic vesicles) is critical for activating the eggs metabolism and initiating development. - Release of inhibitors from maternally stored messages, allows mRNA to be translated. - Release the inhibition of nuclear division, thereby allowing cleavage to occur. 56 1.1. Growth & Development - Block Notes 4.2. Cleavage: division without growth - Occurs before the blastocyst reaches the uterus - 24 hours after fertilisation the impregnated oocyte (zygote) begins with the 1st cleavage division - Mitosis needs to be fast so that G1 and S phase don’t occur ‣ Series of rapid mitotic divisions (no G phase) between day 1 and 5, increasing the number of cells - The cells, the blastomeres (totipotent cells) become smaller at each cleavage division (from 2 to 8 cell stages) and the embryo total volume does not increase (because of the zona pellucida) - From 8 to 16 cell — early-morula and then a late morula — 16-32 cells - To implant, we first must remove the zona pellucida 4.3. Compactation - The 8 blastomeres group together tightly and form a compact ball ‣ Blastomeres: refers to any cell that results from the division of fertilised egg ‣ Adhesion proteins: (cadherins and integrins) are expressed establishing tight connections between cells Polarisation - By compaction, morula cells organise themselves into external and internal cells. 57 1.1. Growth & Development - Block Notes ‣ The outer cells express Na+/K+ pumps, leading to an increased osmotic pressure (sodium income)→ water follows. ‣ Fluid accumulation results in the formation (cavitation) of a fluid-filled cavity known as the blastocoel — 1st differentiation event - The embryo is now called blastocyst, with an inner cell mass (ICM) or embryoblast and an exterior layer of cells, the trophoblast 4.4. Hatching - Fertilised blastocyst is transported via the movement of the cilia of the tubal epithelium and the contraction of its muscular layer - The late blastocyst arrives at the uterus and must get rid of zona pellucida (6-7th day) ‣ Zona pellucida prevents the blastocyst from adhering to oviduct walls – if adhesion occurs an ectopic pregnancy occurs - Trophoblast cells secrete proteases that digest the proteins in the zona pellucida, allowing the blastocyst to escape from Z.P and attach to the endometrium (implantation) 4.5. Implantation - 8-9th day - Proteases secreted from the trophoblast will digest the endometrium allowing the blastocyst to bury within the uterine wall - Trophoblast cells synthesize integrins that bind to uterine proteins - Window of implantation: period that implantation should occur for it to occur properly 4.6. Delamination - 10-12th day - Once attached to the uterine wall, the trophoblast cells differentiate themselves into the outer syncytiotrophoblasts (ST) and the inner cytotrophoblasts (CT) 58 1.1. Growth & Development - Block Notes - With the blastocyst implantation in the endometrium, the ST develops quickly and will surround the embryo as soon as it has completely embedded itself in the endometrium - The ICM will differentiate into the hypoblast and epiblast (bilaminar germ disc formation) IMPORTANT TO NOTE: Trophoblast invasion has functional similarities with angiogenesis, invasion and metastasis of cancer. The complex balance between proteases and protease inhibitors was a major cause of the failure of many clinical trials of synthetic protease inhibitors as anti-cancer therapy. The tight control of protease activity is likely lost as well in cases of abnormally invasive placentas. 4.7. Gastrulation - Development of the trilaminar germ disc from the bilaminar disc - Formation of the primitive streak/ endoderm, results from the migration of the epiblast to the middle ‣ the hypoblast will form the yolk sac, doesn’t provide any cells to the actual embryo - The epiblast will originate the actual embryo - Movement of epiblast cells through the primitive streak between the epiblast and hypoblast originates the mesoderm and endoderm - The cells remaining in the epiblast will originate the ectoderm 59 1.1. Growth & Development - Block Notes - EMT occurs during cellular events where migration takes place (like in embryogenesis, wound healing or metastasis). 4.7.1. Notochord - Some mesodermal cells migrate through the primitive grove forming the notochordal process which elongates continuously, and other cells can pass through the primitive groove into the layers of the embryo - On around the 20th day the notochordal process becomes the notochord - The notochord’s most important function is the establishment of the 3 embryonic axis. 4.8. Neurolation - As the notochord develops, that induces the ectoderm to form the neural plate - The neural plate invaginates forming neural crests on both sides and separate from the surface ectoderm to form the neural tube, the primordial of the brain and spinal chord - Neural crest cells migrate to the lateral part of the neural tube and several parts of the mesoderm to originate the peripheral nervous system, and melanocytes - Efficient neural tube closure depends on: ‣ Genetic factors (pax3, shh, open brain) 60 1.1. Growth & Development - Block Notes ‣ Diet (cholesterol, folic acid) ‣ Unknown factors 5. Cell potency Totipotent - From egg to 8 cell stage, loose clump of cells - All cells are able to become embryo or placenta Pluripotent - 16-32 cell stage, cell-cell contact via tight junctions - Trophoblast (placenta) + ICM (embryo and amnion, chorion and yolk sac) - ES cells can become any tissue of the embryo, except for the placenta 61 1.1. Growth & Development - Block Notes - IPS (Yamanaka) Multipotent - Two layered embryo; epiblast (embryo) and hypoblast (extra embryonic cavities) - Epiblast: three germ layers. Within germ layer, cells can become a structure/ organ - Also adult cells and cord blood stem cells Unipotent - Within each germ layer cells are specified and become one structure/organ 6. Tissues - Germ Cell Layers Germ layers are the cellular layers in which our organs and tissues first derive from Formed after fertilisation and then rapid cell division begins 6.1. Ectoderm Has selective affinity: strong affinity for the mesoderm and weak affinity to the endoderm — this changes during different stages of development ‣ The attraction level is determined by the amount of cadherins molecules present on the cells surface Its the outermost layer, being superficial to the mesoderm and endoderm It differentiates to form epithelial and neural tissues (skin, lining of the mouth, anus, sweat glands) — the other types of epithelium derive from the endoderm Can be divided in two parts : - Dorsal surface ectoderm (external) — gives rise to epithelium tissues - Neural plate — invaginates to form the neural tube and neural crest — gives rise to neural tissues 6.2. Mesoderm Middle layer of three germ layers 62 1.1. Growth & Development - Block Notes 4 important components: axial mesoderm, paraxial mesoderm, intermediate mesoderm and, lateral plate mesoderm ‣ Axial: gives rise to the notochord ‣ Paraxial: forms the somitomers, which give rise to the mesenchyme of the head and organise into somites in occipital and caudal segments and give rise to sclerotomes (cartilage and bones) and dermatites (subcutaneous tissues of the skin) ‣ Intermediate: connects the paraxial mesoderm with the lateral plate ‣ Lateral plate: gives rise to the heart, blood vessels and blood cells Formed on the 3 week during gastrulation Forms mesenchyme, mesothelium, non epithelial blood cells and coelomocytes Forms the muscle in process, the myogenesis, mesenteries and parts of gonads. Differentiates from the rest of the embryo through intercellular signaling after which the mesoderm is polarised by an organising centre (position is determines by the regions where beta-catenin is protected from degradation by GSK-3) 6.3. Endoderm Gives rise to certain organs such as the colon, the stomach, the intestines, the lungs, the liver and the pancreas Forms the inner lining of organs Clinical case 1: twins Twins can be divided into two main groups according to the number of oocytes that gave rise two both bodies: - Dizygotic: two oocytes must have been ovulated and fertilised forming 2 zygotes. Each zygote receives X from mother an X or Y from father. Difference in sex is possible - Monozygotic: separation of one oocyte into two. Cells of the inner cell mass (pluripotent) have migrated to 2 different poles within the blastocyst. In this case, both have received same information from mother and father. Difference in sex is not possible ‣ In Siamese twins, the separation of the cells is not complete, thus they share some structures/organs 63 1.1. Growth & Development - Block Notes Lecture 8- differentiation and patterning 1. Gene expression The genome is the same in all somatic cells, so this begs the question, how do cells become different form one another? The answer is, through cell differentiation, or specialisation. In fact this, cell differentiation process is possible because of a subsequencial process called differential gene differentiation. Putting in very simple terms, this is the turning on and off of some genes, allowing for a bigger array of proteins to be produced. This can happen through different mechanisms, namely: ‣ Differential gene transcription (promoters, enhancer, transcription factors) ‣ Selective nuclear RNA processing (mRNA; ex. alternative splicing) ‣ Selective mRNA translation (proteins) ‣ Differential protein modification (functional protein) But if the cell actually regulates which genes are turn-on and turn-off, how does it make such decisions? The cell expresses different genes because it receives different clues. These can be external or internal clues: ‣ Internal clues: asymmetrical segregation of cellular determinants ‣ External clues: paracrine or soluble factors (growth factors, cytokines) or juxtacrine (cell adhesion molecules, ECM) These clues are deciphered through cell communication and signal transduction. 1.1. Cell fate A cell’s fate, differentiation-wise, though specified or committed, is not determined. This means it is a result of cell intrinsic programming in conjunction with environmental signs (the clues we just talked about), which is why this fate can be altered if the cell is in a coercive environment. During development, cell potency decreases as cell specialisation increases. It depends on: 64 1.1. Growth & Development - Block Notes ↳ Cell location ↳ Cell receptors ↳ Ligands (e.g. growth factors) Neural crest cells: derive from the ectoderm, next to the neural tube ans start to migrate to different parts of the body, giving rise to melanocytes, neurons from the parasympathetic nervous system, adrenal glands 1.2. Morphogens Special types of signalling molecules that form concentration gradients in a developmental field and induce different cell fates in a concentration dependent manner. Cells closer to the source receive more of the signalling molecules than those that are far away. Each of the millions of cells in the embryo has to make its own decisions (responses) according to its own copy of genetic instructions and its own particular circumstances. Cell proliferation Cell movement Cell interaction Cell specialisation 2. Cell migration Cells can move (short or long distances) depending on several factors (internal and external) — this phenomenon only occurs during embryogenesis. However, like every other cell mechanism, this movement is dependent on a few factors, such as: - Adhesion strength (integrins, cadherins) 65 1.1. Growth & Development - Block Notes - Type of substratum (extracellular matrix) - External migratory signals and clues - Organization of the cellular cytoskeleton (actin) Cells must attach to the extracellular matrix and move and then attach to another part and move again. They receive migratory signals that allow them to know exactly where they need to go. Epithelial cells must undergo a transition to gain the ability to move. ↳ Cut ligations with neighbour cells (tight junctions, for example) and get detached from the basal lamina, now becoming mesenchymal cells. (Epithelial-mesenchymal transition ) (Mesenchymal-epithelial transition ) The determination of ≠ cell types (cell fates) involve progressive restrictions in their development potential. Determination may result from an asymmetric segregation of cellular determinants or the result of inductive signalling between cells. Exposure to ≠ threshold levels of morphogens lead to ≠ cell fates (differentiation) Differentiation follows determination, as the cell elaborates a cell-specific developmental program. Differentiation results in the different cell types that have clear-cut identities, such as muscle cells, nerve cells, and skin cells. Finally, this means that cell fate depends on its neighbours (localisation). 66 1.1. Growth & Development - Block Notes 3. Limb development 3.1. The organisation of the limb The organisation of the limb is commonly related to the Cartesian coordinate system. The anteroposterior axis runs from the first (anterior) to the fifth (posterior) digit. The back of the hand or foot is dorsal, and the palm or sole is ventral. The proximodistal axis extends from the base of the limb to the tips of the digits.