Science Exam PDF
Document Details
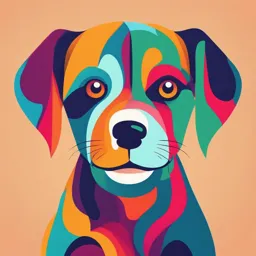
Uploaded by NavigableSard9185
Tags
Summary
This document provides information on ecology, including nutrient cycles (nitrogen and water), food chains, trophic levels, and examples. It details the importance of these concepts in understanding ecosystems and the relationships within them. The document also covers introductory material on the topic.
Full Transcript
ECOLOGY Ecology is the scientific study of the interactions between living organisms and their environment, including both biotic (living) and abiotic (non-living) components. It explores how these interactions shape ecosystems, influence biodiversity, and impact the natural world. Studying ecology...
ECOLOGY Ecology is the scientific study of the interactions between living organisms and their environment, including both biotic (living) and abiotic (non-living) components. It explores how these interactions shape ecosystems, influence biodiversity, and impact the natural world. Studying ecology is crucial because it helps us understand the intricate relationships between living organisms and their environment. By examining these interactions, we can gain insights into how ecosystems function, which is essential for conservation efforts, managing natural resources, and combating climate change. Understanding ecology also allows us to appreciate the delicate balance of nature and the impact of human activities on the planet, guiding us toward more sustainable and environmentally friendly practices. Ultimately, ecology provides the knowledge needed to protect biodiversity and ensure a healthy and resilient planet for future generations. The Earth being a closed system means that it doesn’t exchange matter with its surroundings, so the nutrients within it must be recycled to sustain life. Nutrient cycling is vital because it ensures the continuous availability of essential elements, like carbon, nitrogen, and phosphorus, for organisms to thrive. Without this recycling, nutrients would deplete, leading to the collapse of ecosystems. Example of Nutrient Cycling: The Nitrogen Cycle: Nitrogen is crucial for building proteins and DNA. It cycles between the atmosphere, soil, and living organisms. Nitrogen-fixing bacteria in the soil convert atmospheric nitrogen into forms plants can absorb. Plants use this nitrogen to grow, and when they die, decomposers break them down, returning nitrogen to the soil. Other bacteria then convert it back to atmospheric nitrogen, completing the cycle. The Water Cycle: Water is crucial for all living organisms. The water cycle involves the movement of water through the atmosphere, surface, and underground. Water evaporates from oceans, lakes, and rivers, forming clouds through condensation. It returns to the surface as precipitation (rain, snow), which is then absorbed by plants, animals, and the soil, or it flows back into water bodies, completing the cycle. Pop-Bottle Terrarium as an Example: A pop-bottle terrarium is a miniature ecosystem that demonstrates nutrient cycling. Within this closed environment, plants absorb nutrients from the soil to grow. When plant parts die, decomposers like bacteria and fungi break them down, releasing nutrients back into the soil. This process ensures a continuous supply of essential elements for new plant growth, illustrating the importance of nutrient cycling in sustaining life. Food Chain Example 1. Plants: They are producers that use sunlight to create their own food through photosynthesis. 2. Insects: Primary consumers that feed on plants. They gain energy and nutrients from the plants. 3. Birds: Secondary consumers that feed on insects. They get their energy and nutrients from the insects they eat. 4. Raccoon: Tertiary consumers that can feed on birds, insects, and sometimes plants. They are omnivores and gain energy and nutrients from various sources in the food chain Trophic levels are the hierarchical levels in an ecosystem's food chain, representing the flow of energy and nutrients from one organism to another. Each level consists of organisms that share the same role in the food chain: Trophic Levels: Primary Producers (1st Trophic Level): These are typically plants and algae that produce their own food through photosynthesis. Primary Consumers (2nd Trophic Level): Herbivores that feed on primary producers, such as insects and small animals. Secondary Consumers (3rd Trophic Level): Carnivores or omnivores that feed on primary consumers, such as birds and small mammals. Tertiary Consumers (4th Trophic Level): Carnivores that feed on secondary consumers, such as larger predators like raccoons and foxes. Quaternary Consumers (5th Trophic Level): Apex predators at the top of the food chain that have no natural predators, like eagles and large carnivores. Autotroph (producer) and Heterotroph(consumer) Autotrophs, such as plants, algae, and some bacteria, perform photosynthesis to produce their own food. Both autotrophs and heterotrophs, which include animals, fungi, and many bacteria, perform cellular respiration to convert the energy stored in food into a usable form. If half the raccoons died from a viral disease, bird and insect populations would increase due to reduced predation. This could lead to greater herbivory pressure on plants, affecting their health and growth. Other predators might fill the gap left by raccoons, altering ecosystem balance and interactions. 10% rule: 10 percent of joules are carried over through the organisms so 14000 would go to 1400. Bioaccumulation: The gradual accumulation of toxins in an individual organism over its lifetime. Biomagnification is the process by which the concentration of toxic substances increases in organisms at each successive trophic level of a food chain. This happens because the toxins are not easily broken down or excreted, so they accumulate in the tissues of organisms. Smallest to Largest: Population: A group of individuals of the same species living in a specific area. Community: All the populations of different species that live and interact in a particular area. Ecosystem: A community of living organisms along with the non-living components of their environment, interacting as a system. Biosphere: The global sum of all ecosystems, including all of Earth's living organisms and the environments in which they live. Biotic Factors: Definition: Biotic factors are the living components of an ecosystem. Examples: Plants, animals, fungi, bacteria, and any other living organisms. Role: These factors interact with each other and with abiotic factors, forming food webs and influencing reproduction, predation, and symbiotic relationships. Abiotic Factors: Definition: Abiotic factors are the non-living physical and chemical components of an ecosystem. Examples: Sunlight, temperature, water, soil, air, minerals, and climate. Role: These factors affect the living conditions for organisms, influencing their survival, growth, and reproduction. Sustainability refers to the ability to maintain or improve systems and processes over the long term without depleting resources or causing harm to the environment, society, or economy. It emphasizes meeting the needs of the present without compromising the ability of future generations to meet their own needs. This concept involves balancing environmental health, economic viability, and social equity to ensure a thriving and resilient planet. Lithosphere: The solid, outer part of the Earth, which includes the crust and the upper mantle. It consists of rocks, minerals, and soils. Hydrosphere: All the water on Earth, including oceans, rivers, lakes, groundwater, and even moisture in the atmosphere. Atmosphere: The layer of gases that surrounds the Earth, providing the air we breathe and protecting us from the sun's harmful radiation. Photosynthesis: Word Equation: Carbon dioxide + Water + Light energy → Glucose + Oxygen Math Equation: 6CO2+6H2O+ light energy → 𝐶6𝐻12𝑂6+6O2 Cellular Respiration: Word Equation: Glucose + Oxygen → Carbon dioxide + Water + Energy (ATP) Math Equation: 𝐶6𝐻12𝑂6+6O2 → 6CO2+6H2O+energy (ATP) Plants use sunlight, water, and carbon dioxide to produce glucose and oxygen. This process takes place in the chloroplasts of plant cells, where chlorophyll absorbs light energy to convert water and carbon dioxide into glucose, with oxygen released as a byproduct. Symbiosis refers to a close and long-term interaction between two different species. These interactions can be beneficial, neutral, or harmful to one or both of the organisms involved. Here are the main types of symbiosis: 1. Mutualism: Both organisms benefit from the relationship. Example: Bees and flowers—bees get nectar for food, while flowers are pollinated. 2. Commensalism: One organism benefits, and the other is neither harmed nor helped. Example: Barnacles on whales—barnacles get a place to live and feed, while the whale is unaffected. 3. Parasitism: One organism benefits at the expense of the other. Example: Ticks on a dog—ticks feed on the dog's blood, harming the dog. Carrying capacity refers to the maximum number of individuals of a particular species that an environment can support sustainably over time, without degrading the environment. It is determined by the availability of resources such as food, water, and shelter, as well as factors like predation, disease, and competition. Two key factors that can limit population growth are: 1. Availability of Resources: Limited access to food, water, shelter, and other essential resources can constrain population growth as individuals compete for these necessities. 2. Predation and Disease: Higher rates of predation and disease can reduce population size by increasing mortality rates and decreasing the overall health and reproductive success of the population. Biodiversity refers to the variety and variability of life on Earth, encompassing the different species, genes, ecosystems, and the ecological processes they are part of. Biodiversity is vital for the health and stability of ecosystems, as it ensures natural sustainability and resilience to environmental changes. Habitat destruction and pollution are how humans are hurting ecosystems. They can help biodiversity by 1. Protect Habitats: Establishing and maintaining protected areas to ensure safe spaces for species to thrive. 2. Sustainable Practices: Implementing sustainable agricultural, forestry, and fishing methods to preserve ecosystems and biodiversity. Competition: Definition: Both organisms vie for the same limited resources (e.g., food, water, shelter). Impact: This competition can lead to reduced resource availability, causing stress and potential population decline for both species. It may result in one organism outcompeting the other, leading to the exclusion or displacement of the less competitive species. Predator-Prey: Definition: One organism (predator) hunts and consumes the other (prey). Impact: This relationship helps regulate the population sizes of both species, maintaining ecological balance. Predators control prey populations, preventing overpopulation and resource depletion. The prey population, in turn, influences predator numbers, as an abundant prey supply supports more predators, while a decline in prey can reduce predator numbers. CHEMISTRY States of Matter Solid: Has a definite shape and volume. The particles are closely packed together and only vibrate in place. Liquid: Has a definite volume but no definite shape. It takes the shape of its container, and the particles are less tightly packed than in solids. Gas: Has no definite shape or volume. The particles are spread out and move freely. Pure Substances Elements: Made of one type of atom. Examples include gold (Au) and oxygen (O₂). Compounds: Made of two or more types of atoms chemically bonded together. Examples include water (H₂O) and carbon dioxide (CO₂). Types of Mixtures Homogeneous Mixtures: The composition is uniform throughout. Examples include saltwater and air. Heterogeneous Mixtures: The composition is not uniform. Examples include salad and sand.. Physical Properties: Mass: The amount of matter in an object, usually measured in grams or kilograms. Volume: The amount of space an object occupies, often measured in liters or cubic meters. Density: The mass of an object per unit volume, calculated as mass divided by volume. Color, Odor, and Taste: These are sensory properties that can help identify a substance. Melting and Boiling Points: The temperatures at which a substance changes from solid to liquid (melting) or liquid to gas (boiling). Hardness: The resistance of a material to being scratched or dented. Solubility: The ability of a substance to dissolve in another substance (e.g., sugar dissolving in water). 2. Chemical Properties: Reactivity: How a substance interacts with other substances, leading to chemical changes. Flammability: The ability of a substance to burn in the presence of oxygen. Acidity or Basicity (pH): A measure of how acidic or basic a substance is. Acids have a pH less than 7, bases have a pH greater than 7, and neutral substances have a pH of 7. Oxidation State: The degree of oxidation of an atom in a chemical compound. 3. Thermal Properties: Thermal Conductivity: The ability of a material to conduct heat. Thermal Expansion: The increase in volume of a material when it is heated. 4. Electrical Properties: Conductivity: The ability of a material to conduct electricity. Resistivity: The resistance of a material to the flow of electric current. Atomic Number (Z): This is the number of protons in an atom. It is unique to each element. Number of Protons: Equal to the atomic number (Z). Number of Electrons: In a neutral atom, the number of electrons is equal to the number of protons. Number of Neutrons (N): Calculated using the formula: Number of Neutrons (N) = Mass Number (A) - Atomic Number (Z) Mass Number (A): The sum of the number of protons and neutrons: Mass Number (A) = Number of Protons (Z) + Number of Neutrons (N) The first 20 elements of the periodic table, in order, are Hydrogen, Helium, Lithium, Beryllium, Boron, Carbon, Nitrogen, Oxygen, Fluorine, Neon, Sodium, Magnesium, Aluminium, Silicon, Phosphorus, Sulfur, Chlorine, Argon, Potassium, and Calcium. Group 1: Alkali Metals (e.g., Lithium, Sodium) 1 valence electron Group 2: Alkaline Earth Metals (e.g., Beryllium, Magnesium) 2 valence electrons Group 17: Halogens (e.g., Fluorine, Chlorine) 7 valence electrons Group 18: Noble Gases (e.g., Helium, Neon) 8 valence electrons You can tell groups by the number of valence electrons. Atomic Radius Decreases across a period (left to right). Increases down a group (top to bottom). Ionization Energy Increases across a period. Decreases down a group. Electronegativity Increases across a period. Decreases down a group. Metallic Character Decreases across a period. Increases down a group. Structure of the Atom: 1. Nucleus: ○ Protons: Positively charged particles found in the nucleus. ○ Neutrons: Neutral particles (no charge) found in the nucleus. ○ The nucleus contains most of the atom's mass. 2. Electron Cloud: ○ Electrons: Negatively charged particles that move around the nucleus in regions called energy levels or shells. ○ Electrons are much smaller than protons and neutrons and occupy most of the atom's volume. Bohr-Rutherford Diagrams: Bohr-Rutherford diagrams (or Bohr diagrams) are used to represent the arrangement of electrons around the nucleus. Here's how to draw one: 1. Write down the atomic number, symbol, element name and mass number 2. Identify the number of protons (atomic number) and place them in the nucleus. 3. Calculate the number of neutrons using the formula: Neutrons = Mass Number - Atomic Number. 4. Arrange the electrons in energy levels (shells) around the nucleus. ○ The first shell can hold up to 2 electrons. ○ The second shell can hold up to 8 electrons. ○ The third shell can hold up to 8 electrons (but is often shown with up to 8 for simplicity in lower-level diagrams). Molecules: Groups of two or more atoms bonded together. Compounds: Substances made of different elements bonded together in fixed ratios. Counting Atoms: Use the chemical formula to determine the number of each type of atom in the molecule or compound. Example: Water (H₂O) H₂O: The formula indicates 2 hydrogen (H) atoms and 1 oxygen (O) atom. Example: Glucose (C₆H₁₂O₆) C₆H₁₂O₆: This formula indicates 6 carbon (C) atoms, 12 hydrogen (H) atoms, and 6 oxygen (O) atoms. -ide: Use for simple binary compounds (two elements). ○ Example: Sodium chloride (NaCl). -ate: Use for polyatomic ions with oxygen. ○ Example: Sulfate (SO₄²⁻). -ite: Use for polyatomic ions with one less oxygen than the "-ate" ion. ○ Example: Sulfite (SO₃²⁻). Prefixes: 1. Mono-: One atom. ○ Example: Carbon monoxide (CO). 2. Di-: Two atoms. ○ Example: Carbon dioxide (CO₂). 3. Tri-: Three atoms. ○ Example: Sulfur trioxide (SO₃). 4. Tetra-: Four atoms. ○ Example: Tetrachloromethane (CCl₄). 5. Penta-: Five atoms. ○ Example: Phosphorus pentachloride (PCl₅). Dalton's Atomic Model: John Dalton (1808) proposed that atoms are the indivisible particles of matter. Atoms of a given element are identical in mass and properties. Atoms combine in fixed ratios to form compounds. Diagram: Think of it as a solid, indivisible sphere. Thomson's Atomic Model: J.J. Thomson (1897) discovered the electron through his cathode ray experiments. Proposed the "Plum Pudding Model": ○ Atoms consist of a positively charged "pudding" with negatively charged electrons embedded within, like plums in a pudding. Diagram: A positively charged sphere with electrons scattered throughout. Rutherford's Atomic Model: Ernest Rutherford (1911) conducted the gold foil experiment. Discovered that atoms have a small, dense, positively charged nucleus. Electrons orbit around this nucleus. Diagram: A nucleus at the center with electrons orbiting around it, like planets around the sun. Bohr's Atomic Model: Niels Bohr (1913) refined Rutherford's model. Proposed that electrons orbit the nucleus in fixed energy levels or shells. Electrons can jump between energy levels by absorbing or emitting energy. Diagram: Electrons in discrete orbits around the nucleus. All Matter is Made Up of Tiny Particles: Everything is composed of particles, which can be atoms, molecules, or ions. These particles are too small to be seen with the naked eye. Particles are in Constant Motion: Particles of matter are always moving. The speed and nature of this movement depend on the state of the matter (solid, liquid, gas). There are Spaces Between Particles: There is empty space between particles. The amount of space depends on the state of matter, being smallest in solids and largest in gases. Particles Attract Each Other: Particles are attracted to each other, holding matter together. The strength of this attraction varies between different substances. Temperature Affects Particle Motion: Increasing temperature increases the energy and speed of particle motion. Decreasing temperature slows down the motion of particles. Volume = mass/volume ELECTRICITY 1. Insulators vs Conductors: Insulators: Materials that do not allow electrons to flow freely. They hold onto their electrons tightly, preventing the transfer of electrical charge. Examples include rubber, glass, plastic, and wood. Conductors: Materials that allow electrons to flow freely. They have loosely held electrons that can move easily. Examples include metals like copper, aluminum, and gold. 2. Charging Objects: Friction: When two different materials are rubbed together, electrons can be transferred from one material to the other. One object becomes negatively charged (gains electrons), and the other becomes positively charged (loses electrons). ○ Example: Rubbing a balloon against your hair. Contact (Conduction): When a charged object touches a neutral object, electrons are transferred between the two objects, causing the neutral object to become charged. ○ Example: Touching a metal doorknob after walking on a carpet. Induction: A method of charging an object without direct contact. A charged object is brought near a neutral object, causing a redistribution of charges within the neutral object. ○ Example: Bringing a negatively charged rod close to a neutral metal sphere, causing the side of the sphere nearest the rod to become positively charged. 3. Electrostatic Series: The electrostatic series is a list of materials arranged according to their tendency to gain or lose electrons. When two materials are rubbed together, the one higher on the series loses electrons and becomes positively charged, while the one lower gains electrons and becomes negatively charged. ○ Example: Higher: Wool (loses electrons, becomes positive) Lower: Rubber (gains electrons, becomes negative) 4. Static Electricity in Our Lives: Lightning: A dramatic example of static electricity. During a thunderstorm, particles in clouds rub together, creating a buildup of static electricity. When the buildup becomes too great, it is released as a lightning bolt. Dryers: Clothes dryers can cause static cling. As clothes tumble and rub against each other, electrons are transferred, causing some items to become positively charged and others negatively charged. The opposite charges attract, making clothes stick together. Like Charges Repel: Positive charges repel other positive charges. Negative charges repel other negative charges. Example: Two positively charged balloons will push away from each other. Opposite Charges Attract: Positive charges attract negative charges. Negative charges attract positive charges. Example: A negatively charged balloon will stick to a positively charged wall. Current Electricity 1. Circuits: Electric Circuit: A closed loop that allows electric charges to flow. Components: Includes a power source (battery), conductors (wires), load (resistors, bulbs), and a switch. 2. Circuit Symbols and Diagrams: Battery: Resistor: Switch: Bulb: Wire: 3. Series vs. Parallel Circuits: Series Circuit: Components are connected in a single path. Current is the same through all components. Total resistance is the sum of individual resistances. Parallel Circuit: Components are connected in separate branches. Voltage is the same across all branches. Total resistance is less than the smallest individual resistance. 4. Current: Current (I): Flow of electric charge, measured in Amperes (A). Formula: I=QtI = \frac{Q}{t}, where QQ is the charge in Coulombs (C) and tt is the time in seconds (s). 5. Ohm's Law: Ohm's Law: Relationship between voltage (V), current (I), and resistance (R). Formula: V=I×RV = I \times R ○ Voltage (V) is measured in volts (V). ○ Current (I) is measured in amperes (A). ○ Resistance (R) is measured in ohms (Ω). 6. Resistance: Resistance (R): Opposition to the flow of current, measured in ohms (Ω). Factors Affecting Resistance: Material, length, cross-sectional area, and temperature. 7. Potential Difference: Potential Difference (Voltage): The difference in electric potential energy per unit charge between two points in a circuit, measured in volts (V). Summary Table Quantity Symbol Unit Formula Current I Amperes (A) I = RV Voltage V Volts (V) V=I×R Resistance R Ohms (Ω) R=VI Energy Generation Energy generation is the process of producing electrical power from various energy sources. Here are the main methods: 1. Fossil Fuels: Coal, Oil, and Natural Gas: Burned to produce steam, which drives turbines connected to generators. Pros: Reliable and abundant. Cons: Releases greenhouse gases and pollutants. 2. Nuclear Energy: Nuclear Fission: Splitting atomic nuclei (usually uranium or plutonium) to release energy. Pros: Low greenhouse gas emissions, high energy output. Cons: Radioactive waste, high initial costs, potential for accidents. 3. Renewable Energy: Solar Power: Photovoltaic (PV) cells convert sunlight directly into electricity. ○ Pros: Clean, renewable, abundant. ○ Cons: Intermittent, depends on weather, high initial cost. Wind Power: Wind turbines convert kinetic energy from wind into electrical energy. ○ Pros: Clean, renewable. ○ Cons: Intermittent, depends on wind availability, noise. Hydropower: Uses flowing or falling water to turn turbines. ○ Pros: Clean, renewable, reliable. ○ Cons: Environmental impact on aquatic ecosystems, expensive to build. Geothermal Energy: Uses heat from the Earth's interior to produce steam and drive turbines. ○ Pros: Clean, renewable, reliable. ○ Cons: Limited to geologically active areas, high initial cost. Biomass: Organic materials (wood, agricultural waste) burned to produce electricity. ○ Pros: Renewable, reduces waste. ○ Cons: Air pollution, competition with food supply. 4. Emerging Technologies: Tidal and Wave Energy: Harnesses the energy from ocean tides and waves. ○ Pros: Clean, renewable. ○ Cons: Expensive, environmental impact on marine life. Hydrogen Fuel Cells: Converts hydrogen and oxygen into electricity, with water as a byproduct. ○ Pros: Clean, efficient. ○ Cons: Expensive, hydrogen production can be energy-intensive. Study Sheet: The Sun, Solar System, Stars, and Their Life Cycles 1. Definitions and Key Concepts Astronomical Unit (AU) Definition: An Astronomical Unit (AU) is a standard unit of measurement used to describe distances within our solar system 1 AU = The distance from the Earth to the Sun Distance: 1 AU = 1.5 × 10⁸ kilometres (or 150 million kilometres). Light Year (ly) Definition: A Light Year (ly) is a unit of distance that describes how far light travels in one year. Distance: 1 light year = 9.46 × 10¹² kilometres (or about 5.88 trillion miles). Speed of Light: Light travels at a speed of 299,792 kilometres per second (km/s). Speed of Light Definition: The Speed of Light is the speed at which light travels in a vacuum. Use: The speed of light helps explain why distances in space are measured in light years, as light travels incredibly fast, but space is so vast that even light takes a long time to travel between stars and galaxies. The Sun (Sol) Name: The Sun is often referred to simply as the Sun, but its proper name is Sol. Type of Star: Sol is a G-type dwarf star, characterized by moderate temperatures and emitting yellow-white light. Diameter: About 110 Earths could fit across the Sun's diameter. Volume: Approximately 1.3 million Earths could fit inside the Sun. Weight: An object on the Sun’s surface would weigh 28 times more than on Earth. Structure of the Sun Core: ○ Temperature: Around 15 million K. ○ Nuclear Fusion: The Sun fuses hydrogen nuclei into helium, releasing enormous amounts of energy. ○ Hydrogen Consumption: The Sun converts 400 million tonnes of hydrogen into helium every second. Radiative Zone: Extends ¾ of the way up to the surface, where light takes around 100,000 years to pass through.