Safe Management of Healthcare Waste PDF
Document Details
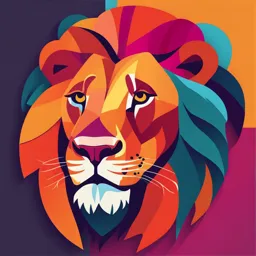
Uploaded by treasuredviking
University of the West Indies, St. Augustine
2014
Yves Chartier, Jorge Emmanuel, Ute Pieper, Annette Prüss, Philip Rushbrook, Ruth Stringer, William Townend, Susan Wilburn and Raki Zghondi
Tags
Summary
This document is a second edition of a guide on safe management of wastes from health-care activities published by the World Health Organization in 2014. It provides information on different types of healthcare waste, their generation rates in various settings and countries.
Full Transcript
Safe management of wastes from health-care activities Second edition Edited by Yves Chartier, Jorge Emmanuel, Ute Pieper, Annette Prüss, Philip Rushbrook, Ruth Stringer, William Townend, Susan Wilburn and Raki Zghondi Safe management of wastes from health-care activities 2nd edi...
Safe management of wastes from health-care activities Second edition Edited by Yves Chartier, Jorge Emmanuel, Ute Pieper, Annette Prüss, Philip Rushbrook, Ruth Stringer, William Townend, Susan Wilburn and Raki Zghondi Safe management of wastes from health-care activities 2nd edition WHO Library Cataloguing-in-Publication Data Safe management of wastes from health-care activities / edited by Y. Chartier et al. – 2nd ed. 1.Medical waste. 2.Waste management. 3.Medical waste disposal – methods. 4.Safety management. 5.Handbook. I.Chartier, Yves. II.Emmanuel, Jorge. III.Pieper, Ute. IV.Prüss, Annette. V.Rushbrook, Philip. VI.Stringer, Ruth. VII.Townend, William. VIII.Wilburn, Susan. IX.Zghondi, Raki. X.World Health Organization. ISBN 978 92 4 154856 4 (NLM classification: WA 790) © World Health Organization 2014 All rights reserved. Publications of the World Health Organization (WHO) are available on the WHO web site (www.who.int) or can be purchased from WHO Press, World Health Organization, 20 Avenue Appia, 1211 Geneva 27, Switzerland (tel.: +41 22 791 3264; fax: +41 22 791 4857; e-mail: [email protected]). Requests for permission to reproduce or translate WHO publications –whether for sale or for non-commercial distribution– should be addressed to WHO Press through the WHO web site (www.who.int/about/licensing/copyright_form/en/index.html). The designations employed and the presentation of the material in this publication do not imply the expression of any opinion whatsoever on the part of the WHO concerning the legal status of any country, territory, city or area or of its authorities, or concerning the delimitation of its frontiers or boundaries. Dotted lines on maps represent approximate border lines for which there may not yet be full agreement. The mention of specific companies or of certain manufacturers’ products does not imply that they are endorsed or recommended by WHO in preference to others of a similar nature that are not mentioned. Errors and omissions excepted, the names of proprietary products are distinguished by initial capital letters. All reasonable precautions have been taken by WHO to verify the information contained in this publication. However, the published material is being distributed without warranty of any kind, either expressed or implied. The responsibility for the interpretation and use of the material lies with the reader. In no event shall the WHO be liable for damages arising from its use. The named editors alone are responsible for the views expressed in this publication. Declarations of interest The members of the health-care waste-management working group completed the WHO standard form for declaration of interests prior to the meeting. At the start of the meeting, all participants were asked to confirm their interests, and to provide any additional information relevant to the subject matter of the meeting. It was from this working group that chapter authors and lead editors were selected. None of the members declared current or recent () Country (increasing gross national income per capita) n = total health-care waste; o = infectious waste; points represent averages; vertical columns are ranges of data 1-Bangladesh (includes clinics), 2-Cambodia, 3-Lao PDR, 4-Nigeria, 5-Vietnam, 6-Pakistan, 7-India, 8-Guyana, 9-Philippines, 10-Jordan, 11-Colombia, 12-Peru, 13-Thailand, 14-Iran, 15-Bulgaria, 16-Brazil (includes health centres and laboratories), 17-Turkey; 18-Taiwan (China), 19-Portugal, 20-Hong Kong (China), 21-Kuwait, 22-Italy, 23-United States of America Source: Emmanuel (2007) Figure 2.2 Total and infectious waste generation in selected hospitals (kg per bed per day) Definition and characterization of health-care waste 15 12 bed/day kg/occupied bed-day or 10 or kg/patient/day kg/patient-day 8 kg/occupied 6 4 Average 2 Average 0 0 1 2 3 4 5 6 7 8 9 10 11 12 CountryCountry (increasing ing Grosgross national income per capita) Country (increasing gross national income per capita) (incre as s National Incom e pe r capita→) n = total health-care waste; o = infectious waste 1-Tanzania, 2-Vietnam, 3-Mongolia, 4-Bhutan, 5-Jordan, 6-Ecuador, 7-Peru, 8-Bulgaria, 9-South Africa, 10-Mauritius, 11-United States of America Source: Emmanuel (2007) Figure 2.3 Total and infectious waste generation in selected hospitals (kg per occupied bed per day or kg per patient per day) 0.8 kg/patient/day 0.7 kg/patient-day 0.6 0.5 0.4 0.3 Average Average 0.2 0.1 0 0 1 2 3 4 5 6 7 8 Countries Countrie (increasing s (incre gross as ing Gros national Incom s National incomee per pe rcapita) capital→) n = total health-care waste; o = infectious waste 1-Tanzania, 2-Bangladesh, 3-Pakistan, 4-Mongolia, 5-Ecuador, 6-South Africa, 7-Mauritius Source: Emmanuel (2007) Figure 2.4 Total and infectious waste generation in small clinics, health centres and dispensaries (in kg per patient per day) 16 Safe management of wastes from health-care activities Table 2.5 Total and infectious waste generation by type of health-care facility (Pakistan, Tanzania, South Africa) Type of health-care facility Total health-care waste generation Infectious waste generation Pakistan Hospitals 2.07 kg/bed/day (range: 1.28–3.47) Clinics and dispensaries 0.075 kg/patient-day 0.06 kg/patient-day Basic health units 0.04 kg/patient-day 0.03 kg/patient-day Consulting clinics 0.025 kg/patient-day 0.002 kg/patient-day Nursing homes 0.3 kg/patient-day Maternity homes 4.1 kg/patient-day 2.9 kg/patient-day Tanzania Hospitals 0.14 kg/patient-day 0.08 kg/patient-day Health centres (urban) 0.01 kg/patient-day 0.007 kg/patient-day Rural dispensaries 0.04 kg/patient-day 0.02 kg/patient-day Urban dispensaries 0.02 kg/patient-day 0.01 kg/patient-day South Africa National central hospital 1.24 kg/patient-bed/day Provincial tertiary hospital 1.53 kg/patient-bed/day Regional hospital 1.05 kg/patient-bed/day District hospital 0.65 kg/patient-bed/day Specialized hospital 0.17 kg/patient-bed/day Public clinic 0.008 kg/patient-day Public community health centre 0.024 kg/patient-day Private day-surgery clinic 0.39 kg/patient-day Private community health centre 0.07 kg/patient-day Sources: Pakistan data from four hospitals and other facilities in Karachi; Pescod & Saw (1998). Tanzania data based on a survey of facilities in Dar es Salaam; Christen (1996), used with permission. South Africa data from a survey of 13 hospitals and 39 clinics in Gauteng and Kwa Zulu Natal; clinics have no beds and may not be open all week; community health centres have up to 30 beds and operate 7 days a week; DEAT (2006) Definition and characterization of health-care waste 17 Table 2.6 Total and infectious waste generation by type of health-care facility: high-income country (United States of America) Type of health-care facility Total health-care waste Infectious waste generation generation Metropolitan general hospitals 10.7 kg/occupied bed/day 2.79 kg/occupied bed/day Rural general hospitals 6.40 kg/occupied bed/day 2.03 kg/occupied bed/day Psychiatric and other hospitals 1.83 kg/occupied bed/day 0.043 kg/occupied bed/day Nursing homes 0.90 kg/occupied bed/day 0.038 kg/occupied bed/day Laboratories 7.7 kg/day 1.9 kg/day Doctor’s office (group practice, urban) 1.78 kg/physician-day 0.67 kg/physician-day Doctor’s office (individual, urban) 1.98 kg/physician-day 0.23 kg/physician-day Doctor’s office (rural) 0.93 kg/physician-day 0.077 kg/physician-day Dentist’s office (group practice) 1.75 kg/dentist-day 0.13 kg/dentist-day Dentist’s office (individual) 1.10 kg/dentist-day 0.17 kg/dentist-day Dentist’s office (rural) 1.69 kg/dentist-day 0.12 kg/dentist-day Veterinarian (group practice, metropolitan) 4.5 kg/veterinarian-day 0.66 kg/veterinarian-day Veterinarian (individual, metropolitan) 0.65 kg/veterinarian-day 0.097 kg/veterinarian-day Veterinarian (rural) 7.7 kg/veterinarian-day 1.9 kg/veterinarian-day Source: Survey of 37 hospitals, 41 nursing homes, 20 laboratories, 8 funeral homes, 41 doctors’ offices, 64 dentists’ offices and 17 veterinarians in Florida, United States of America; Sengupta (1990) Different average bulk densities for health-care waste have been reported in the literature: 594 kg/m3 (urban hospitals, Tanzania; Mata & Kaseva, 1999); 218 kg/m3 for total waste, 211 kg/m3 for general waste and 226 kg/m3 for contaminated waste (hospitals, Peru; Diaz et al., 2008); 151 kg/m3 for general waste and 262 kg/m3 for contaminated waste (urban hospitals, Philippines; Pescod & Saw, 1998); and 110 kg/m3 including boxes and 100 kg/m3 without boxes (large hospital, Italy; Liberti et al., 1994). More detail on the bulk densities for different components of health-care waste found in Ecuador and Canada is given in Table 2.7. Table 2.7 Bulk densities of health-care waste by components Canada Ecuador Component kg/m 3 Component kg/m3 Human anatomical 800–1200 General wastes 596 Plastics 80–2300 Kitchen wastes 322 Swabs, absorbents 80–1000 Yard wastes 126 Alcohol, disinfectants 800–1000 Paper/cardboard 65 Animal infected anatomical 500–1300 Plastic/rubber 85 Glass 2800–3600 Textiles 120 Bedding, shavings, paper, faecal matter 320–730 Sharps 429 Gauze, pads, swabs, garments, paper, 80–1000 Food wastes 580 cellulose Plastics, polyvinyl chloride (PVC), syringes 80–2300 Medicines 959 Sharps, needles 7200–8000 Fluid, residuals 990–1010 Sources: Ontario Ministry of the Environment (1986); Diaz et al. (2008) Determination of the material composition of general waste is important when setting up recycling programmes. Table 2.8 shows the average material compositions of health-care waste from hospitals in different countries. 18 Safe management of wastes from health-care activities Table 2.8 Average material composition of health-care waste Jordana Peru Turkey Taiwan (China) Kuwait Italy Component % Component % Component % Component % Component % Component % Paper 38 Mixed paper 22 Paper 16 Paper 34 Paper 24 Paper 34 Cardboard 5 Cardboard 5 Cardboard 8 Plastic 27 Plastic 12 Plastic 41 Plastic 26 Plastic 18 Plastics 46 Glass 10 Glass 8 Glass 7 Glass 7 Glass 10 Glass 8 Metals 5 Metal 2 Metal 4 Metal 9 Metal 0.4 Food 17 Food 15 Food 12 Textiles 11 Cotton/ 18 Textiles 10 Textiles 9 Textiles 11 gauze Placenta 8 Anatomical 0.1 Garbage 9 Other 27 Other 3 Other 3 Other 8 Liquids 12 a Kitchen waste excluded Sources: Jordan data based on a 224-bed hospital; Awad, Obeidat & Al-Shareef (2005). Peru data based on an average of 6 hospitals; Ministerio de Salud (1995). Turkey data based on 4 hospitals; Altin et al. (2003). Taiwan (China) data based on an average of 3 hospitals; Chih-Shan & Fu-Tien (1993). Kuwait data from 2 hospitals; Hamoda, El-Tomi & Bahman (2005). Italy data based on 120 samples from a 1900-bed hospital; Liberti et al. (1994) The moisture content of different components of overall health-care waste and infectious waste is shown in Table 2.9. Wide differences are noted. Health-care waste from a 1900-bed hospital in Italy had an average moisture level of 26.76%, with a standard deviation of 8.48%, based on 409 samples (Liberti et al., 1994). Some departments, such as obstetrics, paediatrics and dialysis, had moisture levels as high as 50%. Lower moisture content was found in the waste from four hospitals in Turkey. They had an average moisture content of 14.15%. In addition, these hospitals had an average percentage of incombustibles of about 8% (Altin et al., 2003). Table 2.9 Moisture content (%) of health-care waste components Overall health-care waste (%) Infectious waste (%) Component Ecuador Component Jordan Turkey Component Canada Paper/cardboard 16 Paper 22–57 4.5 Human anatomical 70–90 Food 45 Food 63 Plastics 0–1 Textile 30 Textile 37–68 8.6 Swabs, absorbents 0–30 Plastic/rubber 15 Plastic 11–54 2.8 Alcohol, disinfectants 0–0.2 Kitchen waste 47 Garbage 37–57 Animal-infected anatomical 60–90 Garden wastes 40 Carton 5 Glass 0 Medicines 64 Metal 2.25 Bedding, shavings, paper, faecal 10–50 matter Glass 2.05 Gauze, pads, swabs, garments, 0–30 paper, cellulose Other 8 Plastics, polyvinyl chloride, 0–1 syringes Sharps, needles 0–1 Fluid, residuals 80–100 Sources: Diaz et al. (2008); Awad, Obeidat & Al-Shareef (2005); Altin et al. (2003); Ontario Ministry of the Environment (1986) Definition and characterization of health-care waste 19 The percentages of residues from infectious hospital waste, based on 409 samples from a hospital in Italy, were 66% at 110 °C, 15% at 550 °C, and 14% at 1000 °C. A low heating value of wet hazardous health-care waste was measured at 3500 kcal/kg (14.65 MJ/kg). The ranges of heating values for different components of health-care waste are provided in Table 2.10. Table 2.10 Heating value of health-care waste components Component Heating value (as fired) MJ/kg kcal/kg Human anatomical 2–8.4 400–2000 Plastics 32–46 7700–11000 Swabs, absorbents 13–28 3100–6700 Alcohol, disinfectants 25–32 6100–7800 Animal infected anatomical 2–15 500–3600 Glass 0 0 Bedding, shavings, paper, faecal matter 9–19 2200–4500 Gauze, pads, swabs, garments, paper, 13–28 3100–6700 cellulose Sharps, needles 0–0.1 0–30 Fluid, residuals 0–5 0–1100 Source: Based on Milburn (1990) The approximate chemical composition of hospital waste is 37% carbon, 18% oxygen and 4.6% hydrogen, as well as numerous other elements (Liberti et al., 1994). The toxic metals that are found in health-care waste and that are readily emitted during combustion include lead, mercury, cadmium, arsenic, chromium and zinc. In the past, elemental compositions were used to estimate the products of combustion, but this can be misleading since health-care waste varies widely. Moreover, persistent organic pollutants such as polychlorinated dioxins and furans cannot be predicted reliably from basic elemental compositions. These dioxins and furans are toxic at extremely low concentrations. However, decreasing the percentage of halogenated plastics (such as polyvinyl chloride) reduces the amounts of hydrogen chloride and other halogenated pollutants. As much as 40% of plastic waste in modern hospitals is chlorinated plastics. To facilitate recycling, common plastics are now frequently labelled with internationally recognized symbols and numbers: 1 – polyethylene terephthalate, 2 – high-density polyethylene, 3 – polyvinyl chloride, 4 – low-density polyethylene, 5 – polypropylene, 6 – polystyrene and 7 – other. Unfortunately, many polyvinyl chloride products in health care, such as blood bags, gloves, enteral feeding sets and film wraps, are not labelled. 2.10 Minimum approach to overall management of health-care waste All personnel dealing with health-care waste should be familiar with the main categories of health-care waste as set out in either national or local regulations on waste classification. As a minimum, managers responsible for health- care waste should conduct a walk-through of the facility to identify the medical areas that produce waste, to obtain an initial estimate of the types and quantities of waste generated, and to understand how the waste is handled and disposed of. A rapid assessment, combining observations with interviews and survey questionnaires, should provide sufficient data to identify problems and begin the process of addressing them. 20 Safe management of wastes from health-care activities 2.11 Desirable improvements to the minimum approach Beyond the minimal requirements, health-care facilities should adopt an organized approach to waste characterization to obtain accurate data. This approach is necessary to develop or improve the waste management system in use. Undertaking a formal waste assessment entails planning and preparation. From a systematic assessment, one could: identify locations in the health-care facility where good waste segregation is undertaken and where segregation practices need to be improved determine the potential for recycling and other waste-minimization measures estimate the quantities of hazardous health-care waste that require special handling obtain data to specify and size waste collection and transport equipment, storage areas, treatment technology and disposal arrangements to be used. Key points to remember Between 75% and 90% of the waste produced by health-care facilities is non-hazardous or general health-care waste, and only 10% to 25% of health-care waste has a hazard that requires careful management. The distinct categories of health-care waste are sharps, infectious waste, pathological waste, pharmaceutical (including cytotoxic) waste, hazardous chemical waste, radioactive waste and non-hazardous general waste. Infectious waste can be further classified as wastes contaminated with blood or other body fluids, cultures and stocks, and waste from isolation wards. Hazardous chemical waste includes halogenated and non-halogenated solvents, disinfectants, toxic metals such as mercury, and other organic and inorganic chemicals. Health-care waste comes from many sources, including major sources such as hospitals, clinics and laboratories, as well as minor sources such as doctors’ offices, dental clinics and convalescent homes. A significant portion of non-hazardous, general waste is recyclable or compostable. Waste generation rates vary widely and are best estimated by local measurements. Physicochemical characteristics of wastes vary widely and influence the suitability of individual recycling, collection, storage, transport, treatment and disposal systems. 2.12 References and further reading References Altin S et al. (2003). Determination of hospital waste composition and disposal methods: a case study. Polish Journal of Environmental Studies, 12(2):251–255. Awad AR, Obeidat M, Al-Shareef M (2005). Mathematical-statistical models of generated hazardous hospital solid waste. Journal of Environmental Science and Health, Part A, 39(2):313–327. Chih-Shan L, Fu-Tien J (1993). Physical and chemical composition of hospital waste. Infection Control and Hospital Epidemiology, 14(3):145–150. Christen J (1996). Dar es Salaam Urban Health Project. Health care waste management in district health facilities: situational analysis and system development. St Gallen, Switzerland, Swiss Centre for Development Cooperation in Technology and Management. DEAT (Department of Environmental Affairs and Tourism) (2006). National Waste Management Strategy implementation South Africa: projections for health care risk waste generation. DEAT Report Number 12/9/6. Republic of South Africa, Department of Environmental Affairs and Tourism. Definition and characterization of health-care waste 21 Diaz LF et al. (2008). Characteristics of health care waste. Waste Management, 28(7):1219–1226. Emmanuel J (2007). Survey of health care waste characteristics and generation data from different countries. New York, United Nations Development Programme Global Environment Facility Global Demonstration Project on Health Care Waste. Hamoda HM, El-Tomi HN, Bahman QY (2005). Variations in hospital waste quantities and generation rates. Journal of Environmental Science and Health, A40:467–476. Liberti L et al. (1994). Optimization of infectious hospital waste management in Italy. Part I: Waste production and characterization study. Waste Management and Research, 12(5):373–385. Mato RAM, Kaseva ME (1999). A critical review of industrial and medical waste practices in Dar es Salaam City. Resources, Conservation and Recycling, 25:271–287. Milburn T (1990). Biomedical Waste Incineration BACT Application Considerations. In: Proceedings of the Third National Symposium on Infectious Waste Management. Cited in Barton R et al. (1989). State of the art assessment of medical waste thermal treatment. Irvine, California, Energy & Environmental Research Corporation. Ministerio de Salud, Direccion General de Salud Ambiental [Ministry of Health, General Direction Environmental Health] (1995). Diagnostico situacional del manejo de los residuos solidos de hospitales administrados per el Ministerio de Salud [Situational analysis of hospital solid waste in hospitals administered by the Ministry of Health]. Lima, Peru, Ministerio de Salud, Direccion General de Salud Ambiental. Ontario Ministry of the Environment (1986). Incinerator design and operating criteria – Volume II, Biomedical waste incineration. Ontario, Ontario Ministry of the Environment. Pescod MB, Saw CB (1998). Hospital waste management in four cities: A synthesis report. Nieuwehaven, Netherlands, Urban Waste Expertise Programme, WASTE (UWEP Working Document 8). Sengupta S (1990). Medical waste generation, treatment and disposal practices in the state of Florida. Gainesville, State University System of Florida, Florida Center for Solid and Hazardous Waste Management (Report 90-3). Tudor TL (2007). Towards the development of a standardized measurement unit for health care waste generation. Resources, Conservation and Recycling 50:319–333. WHO (World Health Organization) (2004). Laboratory biosafety manual, 3rd ed. Geneva, World Health Organization. Further reading Economopoulos AP (1993). Environmental technology series: assessment of sources of air, water and land pollution. A guide to rapid source inventory techniques and their use in formulating environmental control strategies. Part 1: Rapid inventory techniques in environmental pollution. Geneva, World Health Organization. Halbwachs H (1994). Solid waste disposal in district health facilities. World Health Forum, 15:363–367. Johannessen LM (1997). Management of health care waste. In: Proceedings of the Environment ’97 Conference, 16–18 February 1997, Cairo. Dokki-Giza, Egyptian Environmental Affairs Agency. NCSA (National Conservation Strategy [Coordinating] Agency) (1996). Study on the management of medical wastes. Gaborone, Botswana, National Conservation Strategy (Co-ordinating) Agency (Report No. NCS/GTZ 7/96, in cooperation with the German Agency for Technical Cooperation, coordinated by Integrated Skills Ltd). 22 Safe management of wastes from health-care activities PAHO (Pan American Health Organization) (1994). Hazardous waste and health in Latin America and the Caribbean. Washington, DC, Pan American Health Organization. Secretariat of the Basel Convention (2003). Technical guidelines on the environmentally sound management of biomedical and health care wastes (Y1; Y3). Basel Convention series/SBC No. 2003/3. Châtelaine, Secretariat of the Basel Convention/United Nations Environment Programme. WHO (World Health Organization) (1985). Management of waste from hospitals and other health care establishments. Report on a WHO meeting, Bergen, 28 June – 1 July 1983. EURO Reports and Studies, No. 97. Copenhagen, World Health Organization. WHO CEPIS (Council of European Professional Informatics Societies) (1994). Guia para el manejo interno de residuos solidos hospitalarios [Guide to the internal management of solid hospital waste]. Lima, World Health Organization/Pan American Sanitary Engineering and Environmental Sciences Center. Definition and characterization of health-care waste 23 3 Risks associated with health-care waste Key questions to answer What are the main types of hazards associated with health-care waste? What are the benefits from good health-care waste management? Who is at risk from health-care waste? What are the public health risks of health-care waste? What are the environmental risks of health-care waste? 3.1 Overview of hazards This chapter is concerned with identifying the types of hazards associated with health-care waste and who may be at risk from them by describing the public and environmental health impacts that need to be controlled. The large component of non-hazardous health-care waste is similar to municipal waste and should not pose any higher risk than waste produced in households. It is the smaller hazardous health-care waste component that needs to be properly managed so that the health risks from exposure to known hazards can be minimized. Protection of the health of staff, patients and the general public is the fundamental reason for implementing a system of health-care waste management. 3.1.1 Types of hazards The hazardous nature of health-care waste is due to one or more of the following characteristics: presence of infectious agents a genotoxic or cytotoxic chemical composition presence of toxic or hazardous chemicals or biologically aggressive pharmaceuticals presence of radioactivity presence of used sharps. 3.1.2 Persons at risk All individuals coming into close proximity with hazardous health-care waste are potentially at risk from exposure to a hazard, including those working within health-care facilities who generate hazardous waste, and those who either handle such waste or are exposed to it as a consequence of careless actions. The main groups of people at risk are: medical doctors, nurses, health-care auxiliaries and hospital maintenance personnel patients in health-care facilities or receiving home care visitors to health-care facilities workers in support services, such as cleaners, people who work in laundries, porters 25 workers transporting waste to a treatment or disposal facility workers in waste-management facilities (such as landfills or treatment plants), as well as informal recyclers (scavengers). The general public could also be at risk whenever hazardous health-care waste is abandoned or disposed of improperly. The hazards associated with scattered, small sources of health-care waste should not be overlooked. These sources include pharmaceutical and infectious waste generated by home-based health care, and contaminated disposable materials such as from home dialysis and used needles from insulin injection, or even illicit intravenous drug use. 3.1.3 Hazards from infectious waste and sharps Infectious waste should always be assumed to potentially contain a variety of pathogenic microorganisms. This is because the presence or absence of pathogens cannot be determined at the time a waste item is produced and discarded into a container. Pathogens in infectious waste that is not well managed may enter the human body through several routes: through a puncture, abrasion or cut in the skin through mucous membranes by inhalation by ingestion. The transmission of infection and its control is illustrated by a “chain of infection” diagram (Box 3.1). Each link in the chain must be present and in the precise sequential order for an infection to occur. Health workers should understand the significance of each link and the means by which the chain of infection can be interrupted. Consequently, good health-care waste management can be viewed as an infection-control procedure. It is also important to note that breaking any link in the chain will prevent infection, although control measures for health- care waste are most often directed at the “mode of transmission” stage in the chain of infection. Box 3.1 Chain of infection Infectious Infectious agent: a microorganism that can cause disease agent Reservoir: a place where microorganisms can thrive and reproduce (e.g. in humans, animals, inanimate objects) Portal of exit: a means for a microorganism to leave the reservoir Susceptible Reservoir (e.g. respiratory, genitourinary and gastrointestinal tracts; skin host and mucous membranes; and the placenta) Mode of transmission: how the microorganism moves from one Chain of infection place to another (e.g. contact, droplets, airborne) Portal of entry: an opening allowing the microorganism to invade a new host Portal Susceptible host: a person susceptible to the disease, lacking Portal of entry of exit immunity or physical resistance to prevent infection Mode of tranmission Source: Adapted from Korn & Lux (2001) 26 Safe management of wastes from health-care activities Examples of infections that might be caused by exposure to health-care waste are listed in Table 3.1, together with the body fluids that are the usual vehicles of transmission and that contaminate waste items. Concentrated cultures of pathogens and contaminated sharps (particularly hypodermic needles) are the waste items that pose the most acute potential hazards to health. Table 3.1 Potential infections caused by exposure to health-care wastes, causative organisms and transmission vehicles Type of infection Examples of causative Transmission vehicles organisms Gastroenteric infections Enterobacteria, e.g. Salmonella, Faeces and/or vomit Shigella spp., Vibrio cholerae, Clostridium difficile, helminths Respiratory infections Mycobacterium tuberculosis, Inhaled secretions, saliva measles virus, Streptococcus pneumoniae, severe acute respiratory syndrome (SARS) Ocular infection Herpesvirus Eye secretions Genital infections Neisseria gonorrhoeae, Genital secretions herpesvirus Skin infections Streptococcus spp. Pus Anthrax Bacillus anthracis Skin secretions Meningitis Neisseria meningitidis Cerebrospinal fluid Acquired immunodeficiency Human immunodeficiency virus Blood, sexual secretions, body syndrome (AIDS) (HIV) fluids Haemorrhagic fevers Junin, Lassa, Ebola and Marburg All bloody products and viruses secretions Septicaemia Staphylococcus spp. Blood Bacteraemia Coagulase-negative Nasal secretion, skin contact Staphylococcus spp. (including methicillian-resistant S. aureus), Enterobacter, Enterococcus, Klebsiella and Streptococcus spp. Candidaemia Candida albicans Blood Viral hepatitis A Hepatitis A virus Faeces Viral hepatitis B and C Hepatitis B and C viruses Blood and body fluids Avian influenza H5N1 virus Blood, faeces There is particular concern about infection with human immunodeficiency virus (HIV) and hepatitis viruses B and C, for which there is strong evidence of transmission from injury by syringe needles contaminated by human blood, which can occur when sharps waste is poorly managed. Although theoretically any needle-stick injury can lead to the transmission of bloodborne infections, there is some evidence that hollow needles are associated with a higher risk of transmission than solid needles, such as suture needles (Puro, Petrosillo & Ippolito, 1995; Trim & Elliott, 2003; Ganczak, Milona & Szych, 2006). Sharps represent a double risk. They may not only cause physical injury but also infect these wounds if they are contaminated with pathogens. The principal concern is that infection may be transmitted by subcutaneous introduction of the causative agent (e.g. viral blood infections). The existence in health-care facilities of bacteria resistant to antibiotics and chemical disinfectants may also contribute to the hazards created by poorly managed health-care waste. It has been demonstrated that plasmids from laboratory strains contained in health-care waste were transferred to indigenous bacteria via the waste disposal system (Novais et al., 2005). Moreover, antibiotic-resistant Escherichia coli have been shown to survive in an activated sludge plant, although there does not seem to be significant transfer of this organism under normal conditions of wastewater disposal and treatment. Risks associated with health-care waste 27 3.1.4 Hazards from chemical and pharmaceutical waste Many of the chemicals and pharmaceuticals used in health care are hazardous. They are commonly present in small quantities in health-care waste, whereas larger quantities may be found when unwanted or outdated chemicals and pharmaceuticals are sent for disposal. Chemical wastes may cause intoxication, either by acute or chronic exposure, or physical injuries – the most common being chemical burns. Intoxication can result from absorption of a chemical or pharmaceutical through the skin or the mucous membranes, or from inhalation or ingestion. Injuries to the skin, the eyes or the mucous membranes of the airways can occur by contact with flammable, corrosive or reactive chemicals (e.g. formaldehyde and other volatile substances). Laboratory staff are regularly exposed to dozens of chemicals during the course of their work, especially in specialist and research hospitals. The hazardous properties most relevant to wastes from health care are as follows: Toxic. Most chemicals are toxic at some level of exposure. Fumes, dusts and vapours from toxic materials can be especially harmful because they can be inhaled and pass quickly from the lungs into the blood, permitting rapid circulation throughout the body. Corrosive. Strong acids and alkali bases can corrode completely through other substances, including clothing. If splashed on the skin or eyes, they can cause serious chemical burns and permanent injury. Some of these also break down into poisonous gases, which further increase their hazardousness. Explosive. Some materials can explode when exposed to heat or flame, notably flammable liquids when ignited in confined spaces, and the uncontrolled release of compressed gases. Flammable. Compounds with this property catch fire easily, burn rapidly, spread quickly and give off intense heat. Many materials used and stored in medical areas, laboratories and maintenance workshops are flammable, including solvents, fuels and lubricants. Chemically reactive. These materials should be used with extreme caution and stored in special containers. Some can burn when exposed to air or water, some when mixed with other substances. It is important to note that reactive materials do not have to be near heat or flames to burn. They may burn spontaneously in the presence of air and also give off vapours that may be harmful if inhaled. Common chemical waste types Mercury Mercury is a naturally occurring heavy metal. At ambient temperature and pressure, mercury is a silvery-white liquid that readily vaporizes and may stay in the atmosphere for up to a year. When released to the air, mercury is transported by air currents, ultimately accumulating in marine and lake bottom sediments. In these environments, bacteria can transform inorganic mercury compounds into an organic form – methyl mercury – which is known to accumulate in fish tissue and subsequently affect humans through the food chain (Box 3.2). Mercury is highly toxic, especially in elemental form or as methyl mercury. It may be fatal if inhaled and harmful if absorbed through the skin. Around 80% of the inhaled mercury vapour is absorbed into the blood through the lungs. The nervous, digestive, respiratory and immune systems and kidneys can be harmed, as well as the lungs. Adverse health effects from mercury exposure can be tremors, impaired vision and hearing, paralysis, insomnia, emotional instability, developmental deficits during fetal development, and attention deficit and developmental delays during childhood. Recent studies suggest that mercury may have no threshold below which some adverse effects do not occur (WHO, 2005). 28 Safe management of wastes from health-care activities Box 3.2 Health sector contribution of mercury in the environment Mercury is used in several medical devices, especially fever thermometers and blood-pressure monitoring equipment. These represent a hazard in terms of both breakage and long-term disposal. A less well-known source of mercury in medical waste is batteries, particularly the small button batteries. American and European manufacturers are removing mercury from their products, but it may still be present in those produced elsewhere (EC, 2006; Department of Environmental Protection, 2009). Many health-care facilities have adopted a policy of gradual replacement with mercury-free alternatives. Health-care facilities also contribute up to 5% of the release of mercury to water bodies through untreated wastewater. Environment Canada estimates that one third of mercury load in sewerage systems comes from dental practices. Health-care waste incineration is one of the main sources of mercury release into the atmosphere from health-care facilities. The United States Environmental Protection Agency estimates that medical incinerators may have historically contributed up to 10% of mercury air releases. In the United Kingdom, more than 50% of total mercury emissions come from mercury contained in dental amalgam, and laboratory and medical devices. Sources: Risher (2003); WHO (2005) Silver The use of mercury in health care is decreasing. Conversely, silver, another toxic heavy metal, is being used in ever more applications, including as a bactericide and in nanotechnology. In large doses, it can turn a person’s skin permanently grey. There is increasing concern with both regulators and others about the potential effects of silver, including the possibility that bacteria develop resistance to the metal and subsequently also develop a resistance to antibiotics (Chopra, 2007; Senjen & Illuminato, 2009). Disinfectants Disinfectants, such as chlorine and quaternary ammonium, are used in large quantities in health-care facilities, and are corrosive. It should also be noted that reactive chemicals such as these may form highly toxic secondary compounds. Where chlorine is used in an unventilated place, chlorine gas is generated as a by-product of its reaction with organic compounds. Consequently, good working practices should be used to avoid creating situations where the concentration in air may exceed safety limits. Pesticides Obsolete pesticides, stored in leaking drums or torn bags, can directly or indirectly affect the health of anyone who comes into contact with them. During heavy rains, leaking pesticides can seep into the ground and contaminate groundwaters. Poisoning can occur through direct contact with a pesticide formulation, inhalation of vapours, drinking contaminated water or eating contaminated food. Other hazards may include the possibility of spontaneous combustion if improperly stored, and contamination as a result of inadequate disposal, such as open burning or indiscriminate burying. 3.1.5 Hazards from genotoxic waste Special care in handling genotoxic waste is essential. The severity of the hazards for health-care workers responsible for the handling or disposal of genotoxic waste is governed by a combination of the substance toxicity itself and the extent and duration of exposure. Exposure to genotoxic substances in health care may also occur during the preparation of, or treatment with, particular drugs or chemicals. The main pathways of exposure are inhalation of dust or aerosols, absorption through the skin, ingestion of food accidentally contaminated with cytotoxic drugs, ingestion as a result of bad practice, such as mouth pipetting, or from waste items. Exposure may also occur through contact with body fluids and secretions of patients undergoing chemotherapy. The cytotoxicity of many antineoplastic drugs is cell-cycle specific, targeted on specific intracellular processes such as DNA synthesis and mitosis. Other antineoplastics, such as alkylating agents, are not phase specific but are cytotoxic at any point in the cell cycle. Experimental studies have shown that many antineoplastic drugs are Risks associated with health-care waste 29 carcinogenic and mutagenic; secondary neoplasia (occurring after the original cancer has been eradicated) is known to be associated with some forms of chemotherapy. Many cytotoxic drugs are extreme irritants and have harmful local effects after direct contact with skin or eyes (Box 3.3). Cytotoxic drugs may also cause dizziness, nausea, headache or dermatitis. Additional information on health hazards from cytotoxic drugs may be obtained on request from the International Agency for Research on Cancer (IARC).1 Any discharge of genotoxic waste into the environment could have disastrous ecological consequences. Box 3.3 Cytotoxic drugs hazardous to eyes and skin Alkylating agents Vesicant (blistering) drugs: aclarubicin, chlormethine, cisplatin, mitomycin Irritant drugs: carmustine, cyclophosphamide, dacarbazine, ifosfamide, melphalan, streptozocin, thiotepa Intercalating agents Vesicant drugs: amsacrine, dactinomycin, daunorubicin, doxorubicin, epirubicin, pirarubicin, zorubicin Irritant drugs: mitoxantrone Vinca alkaloids and derivatives Vesicant drugs: vinblastine, vincristine, vindesine, vinorelbine Epipodophyllotoxins Irritant drugs: teniposide 3.1.6 Hazards from radioactive waste The nature of illness caused by radioactive waste is determined by the type and extent of exposure. It can range from headache, dizziness and vomiting to much more serious problems. Radioactive waste is genotoxic, and a sufficiently high radiation dose may also affect genetic material. Handling highly active sources, such as those used in diagnostic instruments (e.g. gallium sealed sources) may cause much more severe injuries, including tissue destruction, necessitating the amputation of body parts. Extreme cases can be fatal. The hazards of low-activity radioactive waste may arise from contamination of external surfaces of containers or improper mode or duration of waste storage. Health-care workers, and waste-handling and cleaning personnel exposed to radioactivity are most at risk. 3.1.7 Hazards from health-care waste-treatment methods In addition to the specific hazards posed by different types of health-care waste, there are occupational hazards associated with waste-treatment processes. Some are similar to those common in industries using machinery: Flue gases from waste incinerators may have an impact on people living and working close to a treatment site. The health risk is most serious where an incinerator is improperly operated or poorly maintained. If poorly controlled, emissions from waste incinerators may cause health concern from particulates (associated with increased cardiovascular and respiratory mortality and morbidity); volatile metals, such as mercury and cadmium (associated with damage to the immune system, neurological system, lungs and kidneys); and dioxins, furans and polycyclic aromatic hydrocarbons (which are known carcinogens but may also cause other serious health effects) (Fritsky, Kumm & Wilken, 2001; Levendis et al., 2001; Matsui, Kashima & Kawano, 2001; Brent & Rogers, 2002; Lee et al., 2002; Rushton, 2003; Lee, Ellenbecker & Moure-Eraso, 2004; Segura-Muñoz et al., 2004). 1 See http://www.iarc.fr 30 Safe management of wastes from health-care activities Ash from the incineration of hazardous health-care waste may continue to pose a risk. Burnt-out needles and glass may have been disinfected but can still cause physical injury. Furthermore, incinerator ash may contain elevated concentrations of heavy metals and other toxic items, and the ash provides ideal conditions for the synthesis of dioxins and furans, because it is often exposed for a long time to a temperature range of 200–450 °C. Autoclave and steam disinfection treatment methods can also pose potential hazards that need to be managed. In particular, good maintenance and operation should be undertaken to avoid physical injuries from high operating temperatures and steam generation. Post-waste treatment water contains organic and inorganic contaminants. The concentrations should be monitored to ensure that discharges to sewerage systems are within regulated limits. Health-care waste treatment mechanical equipment, such as shredding devices and waste compactors, can cause physical injury when improperly operated or inadequately maintained. Burial of health-care waste in landfill sites may pose hazards to workers and public. The risks are often difficult to quantify, and the most likely injury comes from direct physical contact with waste items. Chemical contaminants or pathogens in landfill leachate may be released into surface streams or groundwater. On poorly controlled land-disposal sites, the presence of fires and subsurface burning waste poses the further hazard of airborne smoke. The smoke may contain heavy metals and other chemical contaminants that over time may affect the health of site workers and the general public. 3.2 Public sensitivity Quite apart from fear of health hazards, the general public is sensitive about the visual impact of anatomical waste, particularly recognisable human body parts, including fetuses. There are no normal circumstances where it is acceptable to dispose of anatomical waste inappropriately, such as dumping in a landfill. In Muslim and some other cultures, especially in Asia, religious beliefs require human body parts to be returned to a patient’s family and buried in cemeteries. 3.3 Public health impact 3.3.1 Impacts of infectious waste and sharps In the year 2000, sharps injuries to health-care workers were estimated to have caused about 66 000 hepatitis B (HBV), 16 000 hepatitis C (HCV) and 200–5000 HIV infections among health-care workers (Prüss-Ustun et al., 2005). For health-care workers, the fractions of these infections that are due to percutaneous occupational exposure to HBV, HCV and HIV are 37%, 39% and 4%, respectively. It is estimated that more than two million health-care workers are exposed to percutaneous injuries with infected sharps every year (Prüss-Üstün et al., 2005). In certain facilities and countries, health-care workers may have several percutaneous sharps injuries per year, although this could be avoided by training on the safe management of sharps. Table 3.2 lists the common medical and waste- management procedures that led to a sharps injury, in selected countries. Scavengers on waste disposal sites are also at significant risk from used sharps (although these risks are not well documented). The risk of a sharps injury among patients and the public is much lower. Risks associated with health-care waste 31 Table 3.2 Frequency of procedure that health-care workers were using at the moment of percutaneous injury, selected countries Procedure involved in the accident (%)a Country Recapping Stuck by Disassembling During Unattended Movement of (reference) colleague device disposal needle patient New Zealand 15.0 NR NR 21.0 NR NR (Lum et al., 1997) Nigeria 18.0 18.0 10.0 NR NR 29.0 (Adegboye et al., 1994) South Africa 17.4 7.2 3.0 9.6 4.8 23.4 (Karstaedt & Pantanowitz, 2001) Taiwan (Guo 32.1 3.1 2.6 6.1 NR NR et al., 1999) USA 12.0 NR NR 13.0 8.0 NR (Mangione et al., 1991) NR, not reported; USA, United States of America a The percentages do not sum to 100% because individual studies reported different categories of procedures from those in this table. Source: Rapiti, Prüss-Üstün & Hutin (2005) The annual rates of injuries from sharps in medical waste for health-care and sanitary service personnel, within and outside hospitals, were estimated by the United States Agency for Toxic Substances and Diseases Registry (ATSDR) in its report to Congress on medical waste (ATSDR, 1990). Many injuries are caused by recapping of hypodermic needles before disposal into sharps containers, by unnecessary opening of these containers, and by using materials that are not puncture-proof to construct these containers. Box 3.4 summarizes data on occupational transmission of HIV. As of 30 June 1999, 191 American workers had been reported to the Centers for Disease Control and Prevention’s (CDC’s) national surveillance system for occupationally acquired HIV infection. Of this number, 136 workers reported occupational exposures to blood, body fluids, or laboratory specimens containing HIV, and were considered possible cases of occupationally acquired HIV infection (Beltrami et al., 2000). The risk of viral hepatitis B and C infection from contact with health-care waste may be more significant, because these viruses are viable outside a host for longer than HIV. The ATSDR report estimated the annual numbers of HBV infections resulting from injuries from sharps among medical personnel and waste-management workers (Table 3.3). The annual number of HBV infections in the United States of America resulting from exposure to health-care waste was between 162 and 321, out of an overall yearly total of 300 000 cases from all causes. There were insufficient data on other infections linked to health-care waste to allow any conclusions to be reached. However, on the basis of the figures for HBV, all personnel handling health-care waste should be immunized against the disease. A similar approach is not possible for HBC, because no vaccine is available. 32 Safe management of wastes from health-care activities Box 3.4 Occupational transmission of HIV in France and the United States of America France In 1992, eight cases of human immunodeficiency virus (HIV) infection were recognized as occupational infections. Two of these cases, involving transmission through wounds, occurred in waste handlers. USA In 1997, the Centers for Disease Control and Prevention (CDC) recognized 52 HIV infections as occupational infections, 45 of which were caused by percutaneous exposure, and 5 of which were caused by mucocutaneous exposure. The infections caused by percutaneous exposure occurred through the following pathways: 41 hollow-bore needles 2 broken glass vials 1 scalpel 1 unknown sharp object. Source: Bouvet & Groupe d’Etude sur le Risque d’Exposition au Sang, 1993; CDC, 1998 Table 3.3 Viral hepatitis B infections caused by occupational injuries from sharps (USA) Professional category Annual number of people Annual number of HBV injured by sharps infections caused by injury Nurses: in hospital 12 600–22 200 56–96 outside hospital 28 000–48 000 26–45 Hospital laboratory workers 800–7500 2–15 Hospital housekeepers 11 700–45 300 23–91 Hospital technicians 12 200 24 Physicians and dentists in 100–400 500 lbs/h. At least 97% of half-hourly average concentrations must meet the first value and 100% must meet the second value. All half-hourly average concentrations taken in any 24-hour period must meet this value. The sampling period for dioxins/furans must be a minimum of 6 hours and a maximum of 8 hours under the EU directive. Treatment and disposal methods AP 42 (EPA, 1996) are emission estimates for incinerators without air pollution equipment and are shown for comparison; adapted from Batterman (2004). Sources: EPA (2011); European Parliament and the Council of the European Union (2000) 123 Incinerators require emission controls equipment to meet modern emission standards. Ferraz & Afonso (2003) determined that without emission controls dioxin concentrations in combustion gases were 93 to 710 times higher than the European Union legal limit (0.1 ng TEQ/m3), depending on variations in the waste composition. Flue (exhaust) gases from incinerators contain fly ash (particulates), heavy metals, dioxins, furans, thermally resistant organic compounds, and gases such as oxides of nitrogen, sulfur, carbon and hydrogen halides. The flue gases should be treated, and this should be done in at least two different stages: “de-dusting” to remove most of the fly ash washing with alkaline substances to remove hydrogen halides and sulfur oxides. Flue gas treatment can be performed by wet, dry or semidry treatment, or a combination of these processes. The temperature of the combustion process has to be very closely controlled to avoid generating furans and dioxins, and the temperature in the flue gases should be cooled down rapidly to prevent dioxins and furans from reforming. Stockholm Convention The Stockholm Convention is a legally binding treaty with the goal of protecting human health and the environment from persistent organic pollutants. Under the convention, the countries party to the treaty are required to use the best available techniques for new incinerators. The Stockholm Convention’s guidelines for best available techniques and best environmental practices limit the levels of dioxins and furans in air emissions to 0.1 ng I-TEQ/Nm3 at 11% O2. Moreover, dioxins in the wastewater of treatment plants treating effluents from any gas treatment scrubber effluents should be well below 0.1 ng I-TEQ per litre. In addition, the guidelines list primary and secondary measures to achieve the performance levels for removal of dioxins and furans. The primary measures are to: introduce the waste into the combustion chamber only at temperatures of ≥850 °C; install auxiliary burners for start-up and shut-down operations; avoid regular starting and stopping of the incineration process; avoid combustion temperatures below 850 °C and cold regions in the flue gas; control oxygen input depending on the heating value and consistency of feed material; maintain minimum residence time of two seconds above 850 °C in the secondary chamber after the last injection of air or at 1100 °C for wastes containing more than 1% halogenated organic substances (generally the case for health-care waste) and 6% O2 by volume; maintain high turbulence of exhaust gases and reduction of excess air by injection of secondary air or recirculated flue gas, preheating of the air-streams or regulated air inflow; conduct on-line monitoring for combustion control (temperature, oxygen content, carbon monoxide, dust), and operation and regulation of the incinerator from a central console. The secondary measures to further reduce dioxins and furans are an appropriate combination of dust-removal equipment and other techniques, such as catalytic oxidation, gas quenching and wet or (semi) dry adsorption systems. Furthermore, fly and bottom ash, as well as wastewater, should be treated appropriately. Carbon monoxide, oxygen in the flue gas, particulate matter, hydrogen chloride, sulfur dioxide, nitrogen oxides, hydrogen fluoride, airflows and temperatures, pressure drops and pH in the flue gas should be routinely monitored according to national laws and manufacturers’ guidance. 8.8.7 Dust removal The design of flue gas cleaning facilities assumes normal operation of an incinerator, especially temperature and air inputs. Depending on the type of incinerator, it is likely to produce between 25 and 30 kg of dust per tonne of waste (known as fly ash). For example, an incinerator of 20 tonnes/day capacity would need to be equipped with dust 124 Safe management of wastes from health-care activities removal equipment to handle at least 600 kg/day (30 kg/tonne × 20 tonnes) of dust. The most common types of dust removal equipment used at incinerator plants are: cyclonic scrubbers fabric dust removers (commonly called “baghouse filters”) electrostatic precipitators. Flue gas emerges from the post-combustion chamber at about 800–1000 °C and must be cooled to 200–300 °C before entering the dust-removal equipment. This can be achieved in cooling towers, called quenching towers or baths, where the gas is cooled by water circulating in a closed system. (The water may subsequently be used for preheating waste or for other purposes.) A common method is the use of a boiler in which heat exchange takes place between the hot flue gas stream and boiler water. The hot flue gas stream is cooled, and boiler water is heated (the energy of this heated water or steam can be used for generating electricity or for other purposes). The flue gas can also be cooled by introducing fresh air, although this method is less efficient. Removal of acids or alkalis Three processes – wet, semi-dry and dry – are available for removing acids such as hydrofluoric acid (HF), hydrochloric acid (HCl), and sulfuric acid (H2SO4). In the wet process, gases are washed in a spraying tower with soda or lime solution, which also contributes to gas cooling and to the removal of very small particulates. In the semi-dry process (also known as semi-wet process), a lime suspension is injected into the gas column. Salts generated by the neutralization process have to be removed. In the dry process, lime powder is injected into the gas column, and the salts produced during the neutralization have to be removed. The wet process is the most efficient of these three options, but requires complex treatment of the resultant wastewater. Wastewater from gas washing and quenching of ashes must undergo a chemical neutralization treatment before being discharged into a sewer. This treatment includes neutralization of acids and flocculation, and precipitation of insoluble salts. Solid residues Sludges from wastewater treatment and from cooling of fly ash should be considered as hazardous waste. They may either be sent to a waste-disposal facility for hazardous chemicals, or be treated onsite by drying, followed by encapsulation. Solid ashes from health-care waste incineration (known as bottom ash) are often assumed to be less hazardous than fly ash and in the past have been reused in civil engineering works. Recently, growing debate about potential leakage of toxic substances from these ashes and possible pollution of groundwater has led some countries to require these ashes to be disposed of in landfills designed specifically for hazardous substances. The United Nations Environment Programme tested two hospital waste incinerators that had been built in the mid-1990s and reported that “the bottom ashes [from a hospital waste incinerator] were between 1,410 and 2,300 ng I-TEQ/kg” (UNEP, 2001). The extremely high concentrations in the bottom ashes reflect the inefficient combustion in the furnace and the synthesis of polychlorinated dibenzodioxins or polychlorinated dibenzofurans overnight. 8.9 Encapsulation and inertization Disposal of untreated health-care waste in municipal landfills is not advisable. However, if the health-care facility has no other option, the waste should be contained in some way before disposal. One option is encapsulation, which involves filling containers with waste, adding an immobilizing material, and sealing Treatment and disposal methods 125 the containers. The process uses either cubic boxes made of high-density polyethylene or metallic drums, which are three quarters filled with sharps or chemical or pharmaceutical residues. The containers or boxes are then filled up with a medium such as plastic foam, bituminous sand, cement mortar, or clay material. After the medium has dried, the containers are sealed and placed into landfill sites. This process, where the encapsulation materials are available, is appropriate for establishments for the disposal of sharps and chemical or pharmaceutical residues. Encapsulation alone is not recommended for non-sharps waste, but may be used in combination with treatment of such waste. The main advantage of the process is its effectiveness in reducing the risk of scavengers gaining access to the hazardous health-care waste. The process of inertization involves mixing waste with cement and other substances before disposal to minimize the risk of toxic substances contained in the waste migrating into surface water or groundwater. It is especially suitable for pharmaceuticals and for incineration ashes with a high metal content (in this case, the process is also called “stabilization”). For the inertization of pharmaceutical waste, the packaging should be removed, the pharmaceuticals ground, and a mixture of water, lime and cement added. A homogeneous mass is formed, and cubes (e.g. of 1 m3) or pellets are produced onsite. Subsequently, these can be transported to a suitable storage site. Alternatively, the homogeneous mixture can be transported in liquid state to a landfill and poured onto the surface of previously landfilled municipal waste, then covered with fresh municipal waste. The following are typical proportions (by weight) for the mixture: 65% pharmaceutical waste 15% lime 15% cement 5% water. The process is reasonably inexpensive and can be performed using relatively unsophisticated mixing equipment. Other than personnel, the main requirements are a grinder or road roller to crush the pharmaceuticals, a concrete mixer and supplies of cement, lime and water. 8.10 Emerging technologies Developing and emerging technologies should be carefully evaluated before their selection for routine use, because most do not have a demonstrable track record in health-care waste applications. Technologies most commonly discussed in the literature are plasma pyrolysis, superheated steam, ozone and promession. Plasma pyrolysis makes use of an ionized gas in the plasma state to convert electrical energy to temperatures of several thousand degrees using plasma arc torches or electrodes. The high temperatures are used to pyrolyse waste in an atmosphere with little or no air. Another emerging technology uses superheated steam at 500 °C to break down infectious, hazardous chemical or pharmaceutical wastes. The vapours are then heated further in a steam- reforming chamber to 1500 °C. These technologies are expensive, and – like incineration – require pollution- control devices to remove pollutants from the exhaust gas. Ozone (O3) can be used for disinfecting waste. Ozone gas is a strong oxidizer and breaks down easily to a more stable form (O2). Ozone systems require shredders and mixers to expose the waste to the bactericidal agent. Ozone has been used for water treatment and air purification. At concentrations greater than 0.1 ppm, ozone can cause eye, nose and respiratory tract irritation. As with other chemical treatment technologies, regular tests should be conducted to ensure that the microbial inactivation standard is met. Promession is a new technology that combines a mechanical process and the removal of heat to destroy anatomical waste. It involves cryogenic freeze-drying using liquid nitrogen and mechanical vibration to disintegrate human 126 Safe management of wastes from health-care activities remains into powder before burial. The process speeds up decomposition, reduces both mass and volume, and allows the recovery of metal parts. Emerging technologies for destroying hazardous chemical waste include gas phase chemical reduction, base- catalysed decomposition, supercritical water oxidation, sodium reduction, vitrification, superheated steam reforming, ozonation, Fe-TAML/peroxide treatment (see section 8.11.3), biodegradation, mechanochemical treatment, sonic technology, electrochemical technologies, solvated electron technology, and phytotechnology (Global Environment Facility, 2004; EPA, 2005). These emerging technologies are not ready for routine application to health-care waste. 8.11 Applications of treatment and disposal methods to specific waste categories Treatment options should be chosen according to the national and local situation. Below are examples from middle- and low-income countries of treatment methods applied to specific components of health-care waste. 8.11.1 Sharps Improper disposal of sharps waste poses a high risk of disease transmission among health-care workers, waste workers and the general public. In India, sharps are often collected in cardboard safety boxes and burned in small incinerators. Several non- burn methods have been developed in response to concerns about air pollution and the short lifespan of brick incinerators (WHO, 2005a; PATH, 2007). The methods generally entail the following steps: 1. using onsite mechanical needle cutters or electric needle destroyers 2. shredding the treated plastic parts 3. burying the metal pieces in sharps pits 4. remelting the plastics for recycling. Alternatively, the sharps waste can be autoclaved, shredded and then encapsulated in cement blocks that later become useful items such as hospital benches. A study in Ukraine used mechanical needle cutters, steam treatment of the separated plastic and needle portions in existing autoclaves, with the options of remelting the plastic syringe parts in recycling plants and burying the needle portions or melting them in a foundry (Laurent, 2005). Similarly, a pilot study by the Swiss Red Cross in Kyrgyzstan used needle cutters, treated the separated plastic syringes and needles in an autoclave, shredded the plastics in a locally made hammer-mill shredder, and sold the plastic pieces to a plastics manufacturer that remelted the plastics to make coat hangers, flower pots and other commodities (Emmanuel, 2006). Needle cutters were used in Guyana, with the plastic portions treated as infectious waste and the needle portions collected in a 45-gallon plastic barrel with an aluminium funnel. A sharps barrel could hold 150 000 needles. Regular cleaning and maintenance of the needle cutters was found to be crucial (Furth, 2007). In the Philippines, 19.5 million auto-disable syringes collected in 5-litre safety boxes during a one-month mass immunization campaign were handled in one of several ways (Emmanuel, Ferrer & Ferrer, 2004): treated in a centralized autoclave facility and buried in special landfill trenches treated in a centralized microwave facility, shredded and buried in the landfill buried in concrete vaults buried in sharps pits with clay or cement floors. Treatment and disposal methods 127 A concrete vault design is shown in Figure 8.5. The groundwater level should be considered, to avoid flooding of the vault. The feeding door should be suitable to the size of the sharps container. 1.0 m 0.3 m 1.0 m 0.3 m Above highest flood water level Ground level 0.1 m thick walls on all sides with 10 mm diameter 1.8 m steel bars spaced every 0.4 m both ways 0.1 m (all sides) 1.0 m Source: Adapted from Emmanuel, Ferrer & Ferrer (2004) Figure 8.5 Sample design of a concrete sharps vault Studies have been conducted to modify existing gravity-fed autoclaves for treating sharps and other infectious wastes (ETLogHealthGmbH, 2007; Emmanuel, Kiama & Heekin, 2008). Modifications include adding special loading baskets, air filters and changing the process cycle to include steam flushing and several pressure pulses to remove air and meet a minimum disinfection level inside the waste containers. 8.11.2 Anatomical waste, pathological waste, placenta waste and contaminated animal carcasses The treatment of anatomical, pathological, and placenta and fetal remains wastes may be bound by sociocultural, religious and aesthetic norms and practices. Two traditional options have been: interment (burial) in cemeteries or special burial sites burning in crematoria or specially designed incinerators. A more recent option is alkaline digestion, especially for contaminated tissues and animal carcasses. Promession is a newer technology designed especially for human cadavers. In some countries, placenta waste is composted or buried in placenta pits designed to facilitate natural biological decomposition. More information about treatment disposal methods of anatomical waste can be found in Annex 6. 128 Safe management of wastes from health-care activities 8.11.3 Pharmaceutical waste Pharmaceutical waste can be minimized by good inventory control or a “just-in-time” inventory strategy; by purchasing drugs in the dosages routinely administered; by monitoring expiration dates so that existing stock is used before newly arrived supplies (also known as good “stock rotation”); by replacing prepackaged unit dose liquids with patient-specific oral doses; and other good management practices (Practice Greenhealth, 2008). Before treatment, pharmaceutical waste should be labelled and sorted using proper personal protective equipment. Pharmaceutical waste can be sorted according to dosage form (solids, semi-solids, powders, liquids or aerosols) or by active ingredient, depending on the treatment options available. Special consideration is needed for controlled substances (e.g. narcotics), anti-infective drugs, antineoplastic and cytotoxic drugs, and disinfectants. Several options exist for small quantities of pharmaceutical waste: return of expired pharmaceuticals to the donor or manufacturer; encapsulation and burial in a sanitary landfill; chemical decomposition in accordance with the manufacturer’s recommendations if chemical expertise and materials are available; dilution in large amounts of water and discharge into a sewer for moderate quantities of relatively mild liquid or semi-liquid pharmaceuticals, such as solutions containing vitamins, cough syrups, intravenous solutions and eye drops. Antibiotics or cytotoxic drugs should not be discharged into municipal sewers or watercourses. For large quantities of pharmaceutical waste, the options available include: encapsulation and burial in a sanitary landfill; incineration in kilns equipped with pollution-control devices designed for industrial waste and that operate at high temperatures; dilution and sewer discharge for relatively harmless liquids such as intravenous fluids (salts, amino acids, glucose). Some emerging technologies include large-scale ozonation and decomposition using iron-tetraamidomacrocyclic ligand (Fe-TAML) peroxide catalysis. These technologies should be evaluated carefully, because many do not have an established record for treating health-care-related pharmaceutical waste. 8.11.4 Cytotoxic waste Chemotherapeutic waste, including cytotoxic, antineoplastic and cytostatic waste, should first be minimized by careful segregation, purchasing optimal drug quantities, using proper spill containment and clean-up procedures, and substituting environmentally persistent drugs with degradable drugs, where possible. Cytotoxic waste is highly hazardous and should never be landfilled or discharged into the sewerage system. Disposal options include: return to the original supplier incineration at high temperatures chemical degradation in accordance with manufacturers’ instructions. Full destruction of all cytotoxic substances may require incineration temperatures up to 1200 °C and a minimum gas residence time of two seconds in the second chamber. The incinerator should be equipped with gas-cleaning equipment. Incineration at lower temperatures may release hazardous cytotoxic vapours into the atmosphere. Incineration in most municipal incinerators, in single-chamber incinerators or by open-air burning, is inappropriate for the disposal of cytotoxic waste. Treatment and disposal methods 129 Chemical degradation methods, which convert cytotoxic compounds into non-toxic/non-genotoxic compounds, can be used for drug residues and for cleaning contaminated urinals, spillages and protective clothing (IARC, 1983; IARC, 1985). These methods are not used widely and require special knowledge. They are not appropriate for treating contaminated body fluids. The International Agency for Research on Cancer (IARC) Unit of Gene– Environment Interactions can be contacted for further information.15 Further information can also be found in Annex 3. It should be noted that neither incineration nor chemical degradation currently provides a completely satisfactory solution for treating waste items, spillages or biological fluids contaminated by antineoplastic agents. Until such a solution is available, hospitals should exercise the utmost care in the use and handling of cytotoxic drugs. Where neither high-temperature incineration nor chemical degradation methods are available, and where exportation of cytotoxic wastes for adequate treatment to a country with the necessary facilities and expertise is not possible, encapsulation or inertization may be considered as a last resort. Alkaline hydrolysis and some of the emerging technologies may have useful applications in the destruction of cytotoxic waste. 8.11.5 Chemical waste Chemical safety and hazardous chemical waste management should ideally be the subject of a national strategy with an infrastructure, cradle-to-grave legislation, competent regulatory authority and trained personnel. Improving the management of chemical waste begins with waste minimization. Minimization options include: substituting highly toxic and environmentally persistent cleaners and solvents with less toxic and environmentally friendly chemicals; using minimum concentrations where possible; ensuring good inventory control (i.e. “just-in-time” purchasing); designing storage areas well; integrating pest management; keeping disinfecting trays covered to prevent loss by evaporation; developing spill prevention and clean-up procedures; recovering solvents using fractional distillation. Where allowed by local regulations, non-recyclable, general chemical waste, such as sugars, amino acids and certain salts, may be disposed of with municipal waste or discharged into sewers. However, official permission from the appropriate authority may be required, and the types and quantities of material that can be discharged may be limited. Generally, conditions for discharge may include restrictions on pollutant concentrations, content of suspended solids, temperature, pH, and, sometimes, rate of discharge. Unauthorized discharge of hazardous chemicals can be dangerous to sewage treatment workers and may adversely affect the functioning of sewage treatment works. Petroleum spirit (volatilizes to produce flammable vapours), calcium carbide (produces flammable acetylene gas on contact with water) and halogenated organic solvents (many compounds are environmentally persistent or ecologically damaging) should not be discharged into sewers. It is not possible to dispose both safely and cheaply of large quantities of hazardous chemical waste without using sophisticated treatment methods. The appropriate means of storage and disposal is dictated by the nature of the hazard presented by the waste. The following measures are suggested: Hazardous chemical wastes of different composition should be stored separately to avoid unwanted chemical reactions. Hazardous chemical waste should not be discharged into sewerage systems. 15 See http://www.iarc.fr 130 Safe management of wastes from health-care activities Large amounts of chemical waste should not be buried, because they may leak from their containers, overwhelm the natural attenuation process provided by the surrounding waste and soils, and contaminate water sources. Large amounts of chemical disinfectants should not be encapsulated, because they are corrosive to concrete and sometimes produce flammable gases. An option for disposing of hazardous chemicals is to return them to the original supplier, who should be equipped to deal with them safely. Where such an arrangement is envisaged, appropriate provisions should be included in the original purchase contract for the chemicals. Preferably, these wastes should be treated by a specialist contractor with the expertise and facilities to dispose safely of hazardous waste. Use of certain products for non-medical purposes may also be considered; for example, use of outdated disinfectants to clean toilets is often acceptable. Photochemicals should be collected separately, because there is a recovery value from silver compounds contained in the solutions. Recovery of silver from photoprocessing wastewater may be possible using cation exchange, electrolytic recovery or filtration. Spent fixing bath and developing bath solutions should be carefully mixed and the neutralized solution stored for a minimum of one day. The mixture should be diluted (1:2) and very slowly poured into a sewer. 8.11.6 Waste containing heavy metals Some health-care wastes contain heavy metals, such as high concentrations of cadmium from dry-cell batteries, and mercury from thermometers, sphygmomanometers, cantor tubes, dilators, mercury switches and some button-shaped batteries. A WHO policy paper on mercury in health care outlines a strategy that includes developing safe mercury clean-up, handling and storage procedures; reducing unnecessary use of mercury equipment; replacing mercury-containing products with mercury-free alternatives; and supporting a replacement of the use of mercury-containing devices in the long term (WHO, 2005b). Wastes containing mercury or cadmium should not be burned or incinerated. Cadmium and mercury volatilize at relatively low temperatures and can cause atmospheric pollution. In some countries, mercury- or cadmium-containing wastes can be sent to facilities that specialize in the recovery of heavy metals. It may also be possible to send back the waste to the suppliers of the original equipment, with a view to reprocessing or final disposal. Exporting the waste to countries with the expertise and facilities for its adequate treatment should also be considered, but only within the rules laid down by the Basel Convention. If none of the above options are feasible, the wastes would have to go to a disposal or storage site designed for hazardous industrial waste. The Secretariat of the Basel Convention has been developing technical guidelines on the environmentally sound management of mercury waste (UNEP, 2012). The guidelines include mercury waste prevention and minimisation, handling, interim storage, transportation, treatment, recovery, long-term storage and disposal. The United Nations Development Programme has developed detailed guidance on the clean-up, transport and interim storage of mercury waste from health-care facilities (UNDP, 2010). 8.11.7 Radioactive waste The treatment and disposal of radioactive waste is generally under the jurisdiction of a nuclear regulatory agency, which defines clearance levels and waste classifications according to activity levels and half-lives of the radionuclides present. A radioactive waste-management plan should include a programme of waste minimization. The primary methods of waste minimization are source reduction, extended storage for decay of radioactivity, and substitution with a non-radioactive alternative. Source reduction strategies include limiting the quantity of radioactivity purchased, and laboratory procedures that reduce the volume of waste generated. Substitution means replacing long-lived radionuclides with shorter half-life radionuclides or non-radioactive substitutes, where possible. Treatment and disposal methods 131 Unsealed sources – short-lived radionuclides Three disposal methods are possible for low-level radioactive waste: “decay in storage”, which is the safe storage of waste until its radiation levels are indistinguishable from background radiation; a general rule is to store the waste for at least 10 times the half-life of the longest lived radionuclide in the waste (more information can be found in Chapter 7); return to supplier; long-term storage at an authorized radioactive waste disposal site. Containers used for storing radioactive waste should be clearly identified (marked with the words “RADIOACTIVE WASTE” and the radiation symbol) and labelled to show the activity of the radionuclide on a particular date, period of storage required, origin of the waste, surface dose rate on a particular date, quantity and responsible person. Facilities should segregate radioactive waste according to the length of time needed for storage: short-term storage (half-lives less than 60 days) and long-term storage (half-lives more than 60 days). Decayed but infectious waste should be disinfected before subsequent treatment and disposal. A health-care facility should ensure that radionuclides are not released to the environment unless: the radioactivity released is confirmed to be below the clearance levels; or the radioactivity of liquid or gaseous effluents is within limits authorized by a regulatory authority. Sealed sources and long-lived radionuclides Sealed sources, long-lived radionucleotides and spent sources (e.g. from X-ray equipment) should be returned to the producer or supplier of their original form. Health-care facilities planning to import a sealed source with a radioactivity greater than 100 MBq should require the supplier to accept the source back after expiration of its useful lifetime and within a year after a notification is made. If this is not possible, the waste must be stored in an approved long-term storage facility in keeping with international g