Cellular Processes PDF
Document Details
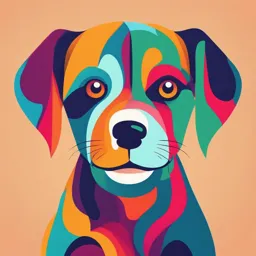
Uploaded by WorthwhileUvite
University of Nueva Caceres
Tags
Summary
This document provides an overview of cellular processes, focusing on cell division, and its various aspects such as mitosis and meiosis. It also includes explanations of concentration gradients, diffusion, active transport and osmosis.
Full Transcript
WIKI WHAT ARE THE CELLULAR PROCESSES This WIKI contains the basic concepts of cellular processes. ---------------------------------------------------------------------------------------------------- Sometimes you accidentally bite your lip or...
WIKI WHAT ARE THE CELLULAR PROCESSES This WIKI contains the basic concepts of cellular processes. ---------------------------------------------------------------------------------------------------- Sometimes you accidentally bite your lip or skin your knee, but in a matter of days, the wound heals. Is it magic? Or is there another explanation? Every day, every hour, and every second, one of the most important events in life is going on in your body—cells are dividing. When cells divide, they make new cells. A single cell divides to make two cells and these two cells then divide to make four cells, and so on. We call this process "cell division" and "cell reproduction, resulting in the formation of new cells when old cells divide. The ability of cells to divide is unique for living organisms. WHY DO CELLS DIVIDE? Cells divide for many reasons. For example, when you skin your knee, cells divide to replace old, dead, or damaged cells. Cells also divide so living things can grow. When organisms grow, it isn't because cells are getting larger. Rather, organisms grow because cells are dividing to produce more and more cells. In human bodies, nearly two trillion cells divide every day. HOW MANY CELLS ARE IN YOUR BODY? You and I began as a single cell, or what is called an “egg”. By the time you are an adult, you will have trillions of cells. That number depends on the size of the person, but biologists put that number around 37 trillion cells. Yes, that is trillion with a "T". HOW DO CELLS KNOW WHEN TO DIVIDE? In cell division, the cell that is dividing is called the "parent" cell. The parent cell divides into two "daughter" cells. The process then repeats in what is called the cell cycle. Cells regulate their division by communicating with each other using chemical signals from special proteins called cyclins. These signals act like switches to tell cells when to start dividing and later when to stop dividing. It is important for cells to divide so you can grow and so your cuts heal. It is also important for cells to stop dividing at the right time. If a cell can not stop dividing when it is supposed to stop, this can lead to a disease called cancer. Some cells, like skin cells, are constantly dividing. We need to continuously make new skin cells to replace the skin cells we lose. Did you know we lose 30,000 to 40,000 dead skin cells every minute? That means we lose around 50 million cells every day. This is a lot of skin cells to replace, making cell division in skin cells very important. Other cells, like nerve and brain cells, divide much less often. HOW DO CELLS DIVIDE? Depending on the type of cell, there are two ways by which cells divide—mitosis and meiosis. Each of these methods of cell division has special characteristics. One of the key differences in mitosis is that a single cell divides into two cells that are replicas of each other and have the same number of chromosomes. This type of cell division is good for basic growth, repair, and maintenance. In meiosis, a cell divides into four cells that have half the number of chromosomes. Reducing the number of chromosomes by half is important for sexual reproduction and provides for genetic diversity. MITOSIS CELL DIVISION Mitosis is how somatic—or non-reproductive cells—divide. Somatic cells make up most of your body's tissues and organs, including skin, muscles, lungs, gut, and hair cells. Reproductive cells (like eggs) are not somatic cells. In mitosis, the important thing to remember is that the daughter cells each have the same chromosomes and DNA as the parent cell. The daughter cells from mitosis are called diploid cells. Diploid cells have two complete sets of chromosomes. Since the daughter cells have exact copies of their parent cell's DNA, no genetic diversity is created through mitosis in normal healthy cells. THE MITOSIS CELL CYCLE Before a cell starts dividing, it is in the "Interphase." It seems that cells must be constantly dividing (remember there are 2 trillion cell divisions in your body every day), but each cell actually spends most of its time in the interphase. Interphase is the period when a cell is getting ready to divide and start the cell cycle. During this time, cells are gathering nutrients and energy. The parent cell is also making a copy of its DNA to share equally between the two daughter cells. The mitosis division process has several steps or phases of the cell cycle—interphase, prophase, prometaphase, metaphase, anaphase, telophase, and cytokinesis—to successfully make the new diploid cells. When a cell divides during mitosis, some organelles are divided between the two daughter cells. For example, mitochondria are capable of growing and dividing during the interphase, so the daughter cells each have enough mitochondria. The Golgi apparatus, however, breaks down before mitosis and reassembles in each of the new daughter cells. Many of the specifics about what happens to organelles before, during, and after cell division are currently being researched. < The mitosis cell cycle includes several phases that result in two new diploid daughter cells. Each phase is highlighted here and shown by light microscopy with fluorescence. Click on the image to learn more about each phase. (Image from OpenStax College with modified work by Mariana Ruiz Villareal, Roy van Heesheen, and the Wadsworth Center.)> MEIOSIS CELL DIVISION Meiosis is the other main way cells divide. Meiosis is cell division that creates sex cells, like female egg cells or male sperm cells. What is important to remember about meiosis? In meiosis, each new cell contains a unique set of genetic information. After meiosis, the sperm and egg cells can join to create a new organism. Meiosis is why we have genetic diversity in all sexually reproducing organisms. During meiosis, a small portion of each chromosome breaks off and reattaches to another chromosome. This process is called "crossing over" or "genetic recombination." Genetic recombination is the reason full siblings made from egg and sperm cells from the same two parents can look very different from one another. < The meiosis cell cycle has two main stages of division -- Meiosis I and Meiosis II. The end result of meiosis is four haploid daughter cells that each contain different genetic information from each other and the parent cell. Click for more detail. (Image from Science Primer from the National Center for Biotechnology Information.)> THE MEIOSIS CELL CYCLE Meiosis has two cycles of cell division, conveniently called Meiosis I and Meiosis II. Meiosis I halves the number of chromosomes and is also when crossing over happens. Meiosis II halves the amount of genetic information in each chromosome of each cell. The end result is four daughter cells called haploid cells. Haploid cells only have one set of chromosomes - half the number of chromosomes as the parent cell. Before meiosis I starts, the cell goes through interphase. Just like in mitosis, the parent cell uses this time to prepare for cell division by gathering nutrients and energy and making a copy of its DNA. During the next stages of meiosis, this DNA will be switched around during genetic recombination and then divided between four haploid cells. So remember, Mitosis is what helps us grow and Meiosis is why we are all unique! Aside from Meiosis and Mitosis, there are still other processes that are essential for the proper function of cells. These are as follows: (a) diffusion; (b) active transport; (c) passive transport; (d) exocytosis; (e) endocytosis; and (f) osmosis. UNDERSTANDING DIFFUSION CONCENTRATION GRADIENTS Our bodies need energy for just about everything they do. We use energy to think and to breathe. We also use energy to move things within our bodies, whether we are moving large muscles or tiny nutrients. All organisms spend energy to build molecules. One of the ways this is done is by using energy created by concentration gradients. Concentration can be thought of as how common something is in a specific area. Imagine sticking a Popsicle into a glass of water. The Popsicle has sugar and flavoring all packed in tight, but as it melts, that sugar and flavoring start to mix with the water. Why does the melting popsicle mix with water? Why does it not melt and stay in a separate layer as when oil is mixed with water? This is because molecules tend to move from areas of high concentration to low concentration. In the given example, there is no sugar in the water, making it a lower concentration compared with the frozen popsicle yet to be dissolved. Thus, the sugar moves away from the Popsicle and mixes into the water. A movement like this is called diffusion. Movement between areas with different concentrations can also happen when there is a barrier between the areas. An example of a barrier is the cell membrane, a sheet of material that acts as a boundary between the inside of the cell, and its outside environment. Within these cell membranes, there are special passageways, which allow molecules to move in and out of the cell. We call these channels or transporters. Many channels and transporters control what materials go in and out of the cell. For instance, ion passes through the membrane through a channel or transporter made up of protein. This selective permeability of the cell membrane causes differences in the concentration of the materials inside and outside of the cell. A Popsicle is a treat packed with sugar and flavoring. MOVING ON DOWN (THE GRADIENT) Movement of molecules down a concentration gradient can be used to move or change other molecules. When the concentration of something builds upon only one side of a membrane, such as when concentration is high on one side, but low on the other, there is a concentration gradient. Let's think of this in terms of a hydrogen ion, H+. Hydrogen ions naturally move from high to low concentration. Think of the ions as trying to even out the concentration of the areas. To understand this idea, imagine that ions are water and the cell membrane is a dam. If one side of the dam has a lot more water, there will also be a higher pressure on the same side. When the flood gate opens, the water will flow through (from the side with more water to the side with less water) until the water on both sides of the dam is of the same level. As the water further flows through the dam, it can be used to turn a turbine (like a water wheel) to generate electricity. Going back to the cell membrane, the gradience, and how it facilitates movement can be used to store more energy in molecules like ATP. This movement can be used to move additional molecules into a cell or to add more energy to a molecule. ACTIVE TRANSPORT During active transport, molecules move from an area of low concentration to an area of high concentration. This is the opposite of diffusion, and these molecules are said to flow against their concentration gradient. Active transport is called "active" because this type of transport requires energy to move molecules. ATP is the most common source of energy for active transport. As molecules are moving against their concentration gradients, active transport cannot occur without assistance. A carrier protein is always required in this process. Like facilitated diffusion, a protein in the membrane carries the molecules across the membrane, except this protein moves the molecules from a low concentration to a high concentration. These proteins are often called "pumps" because they use energy to pump the molecules across the membrane. There are many cells in your body that use pumps to move molecules. For example, your nerve cells (neurons) would not send messages to your brain unless you had protein pumps moving molecules by active transport. The sodium-potassium pump (Figure below) is an example of an active transport pump. The sodium-potassium pump uses ATP to move three sodium (Na+) ions and two potassium (K+) ions to where they are already highly concentrated. Sodium ions move out of the cell, and potassium ions move into the cell. How do these ions then return to their original positions? As the ions now can flow down their concentration gradients, facilitated diffusion returns the ions to their original positions either inside or outside the cell. The sodium-potassium pump moves sodium ions to the outside of the cell and potassium ions to the inside of the cell, areas where these ions are already highly concentrated. ATP is required for the protein to change shape. ATP is converted into ADP (adenosine diphosphate) during active transport. VOCABULARY Term Definition active transport Movement across a membrane during which molecules move from an area of low concentration to an area of high concentration. ATP Adenosine triphosphate; a usable form of energy inside the cell. carrier protein Transport protein that aids in diffusion by carrying a molecule across the membrane. diffusion Movement of molecules from an area of high concentration to an area of low concentration. sodium-potassiu Protein in the membrane that moves sodium ions to the outside of m pump the cell and potassium ions to the inside of the cell with the input of energy. DIFFUSION Sometimes the cell needs help in moving things as well or facilitating the diffusion process. This is the job of a special type of protein. FACILITATED DIFFUSION Facilitated diffusion is the diffusion of solutes through integral membrane transport proteins. Facilitated diffusion is a type of passive transport. Even though facilitated diffusion involves transport proteins (and is essentially a transport process), it can still be considered passive transport because the solute is moving down the concentration gradient, and no input of energy is required. Facilitated diffusion utilizes proteins known as uniporters. A uniporter can be either a channel protein or a carrier protein. As was mentioned earlier, small nonpolar molecules can easily diffuse across the cell membrane. However, due to the hydrophobic nature of the phospholipids that make up cell membranes, polar molecules and ions cannot do so. Instead, they diffuse across the membrane through transport proteins. A transport protein completely spans the membrane, and allows certain molecules or ions to diffuse across the membrane. Channel proteins, gated channel proteins, and carrier proteins are three types of transport proteins that are involved in facilitated diffusion. A channel protein, a type of transport protein, acts like a pore in the membrane that lets water molecules or small ions through quickly. Water channel proteins allow water to diffuse across the membrane at a very fast rate. Ion channel proteins allow ions to diffuse across the membrane. A gated channel protein is a transport protein that opens a "gate," allowing a molecule to pass through the membrane. Gated channels have a binding site that is specific for a given molecule or ion. A stimulus causes the "gate" to open or shut. The stimulus may be chemical or electrical signals, temperature, or mechanical force, depending on the type of gated channel. For example, the sodium gated channels of a nerve cell are stimulated by a chemical signal which causes them to open and allow sodium ions into the cell. Glucose molecules are too big to diffuse through the plasma membrane easily, so they are moved across the membrane through gated channels. In this way glucose diffuses very quickly across a cell membrane, which is important because many cells depend on glucose for energy. A carrier protein is a transport protein that is specific for an ion, molecule, or group of substances. Carrier proteins "carry" the ion or molecule across the membrane by changing shape after the binding of the ion or molecule. Carrier proteins are involved in passive and active transport. A model of a channel protein and carrier proteins is shown in Figure below. Facilitated diffusion through the cell membrane. Channel proteins and carrier proteins are shown (but not a gated-channel protein). Water molecules and ions move through channel proteins. Other ions or molecules are also carried across the cell membrane by carrier proteins. The ion or molecule binds to the active site of a carrier protein. The carrier protein changes shape, and releases the ion or molecule on the other side of the membrane. The protein then returns to its original shape. ION CHANNELS Ions such as sodium (Na+), potassium (K+), calcium (Ca2+), and chloride (Cl-), are important for many cell functions. Because they are polar, these ions do not diffuse through the membrane. Instead they move through ion channel proteins where they are protected from the hydrophobic interior of the membrane. Ion channels allow the formation of a concentration gradient between the extracellular fluid and the cytosol. Ion channels are very specific as they allow only certain ions through the cell membrane. Some ion channels are always open, others are "gated" and can be opened or closed. Gated ion channels can open or close in response to different types of stimuli such as electrical or chemical signals. SUMMARY 1. Facilitated diffusion is the diffusion of solutes through transport proteins in the plasma membrane. 2. Channel proteins, gated channel proteins, and carrier proteins are three types of transport proteins that are involved in facilitated diffusion. EXOCYTOSIS AND ENDOCYTOSIS Is it possible for objects larger than a small molecule to be engulfed by a cell? Of course it is. This image depicts a cancer cell being attacked by a cell of the immune system. Cells of the immune system consistently destroy pathogens by essentially "eating" them. VESICLE TRANSPORT Some molecules or particles are just too large to pass through the plasma membrane or to move through a transport protein. So cells use two other active transport processes to move these macromolecules (large molecules) into or out of the cell. Vesicles or other bodies in the cytoplasm move macromolecules or large particles across the plasma membrane. There are two types of vesicle transport, endocytosis and exocytosis (illustrated in the Figure below). Both processes are active transport processes, requiring energy. Illustration of the two types of vesicle transport, exocytosis and endocytosis. ENDOCYTOSIS AND EXOCYTOSIS Endocytosis is the process of capturing a substance or particle from outside the cell by engulfing it with the cell membrane. The membrane folds over the substance and it becomes completely enclosed by the membrane. At this point a membrane-bound sac, or vesicle, pinches off and moves the substance into the cytosol. There are two main kinds of endocytosis: Phagocytosis, or cellular eating, occurs when the dissolved materials enter the cell. The plasma membrane engulfs the solid material, forming a phagocytic vesicle. Pinocytosis, or cellular drinking, occurs when the plasma membrane folds inward to form a channel allowing dissolved substances to enter the cell, as shown in the Figure below. When the channel is closed, the liquid is encircled within a pinocytic vesicle. Transmission electron microscope image of brain tissue that shows pinocytotic vesicles. Pinocytosis is a type of endocytosis. Exocytosis describes the process of vesicles fusing with the plasma membrane and releasing their contents to the outside of the cell, as shown in the Figure below. Exocytosis occurs when a cell produces substances for export, such as a protein, or when the cell is getting rid of a waste product or a toxin. Newly made membrane proteins and membrane lipids are moved on top the plasma membrane by exocytosis. Illustration of an axon releasing dopamine by exocytosis. SUMMARY 1. Active transport is the energy-requiring process of pumping molecules and ions across membranes against a concentration gradient. 2. Endocytosis is the process of capturing a substance or particle from outside the cell by engulfing it with the cell membrane, and bringing it into the cell. 3. Exocytosis describes the process of vesicles fusing with the plasma membrane and releasing their contents to the outside of the cell. 4. Both endocytosis and exocytosis are active transport processes. VOCABULARY TERM DEFINITION active transport Energy-requiring movement of substances across a plasma membrane. endocytosis Type of vesicle transport that moves substances into a cell. exocytosis Type of vesicle transport that moves substances out of a cell. phagocytosis Process in which leukocytes engulf and break down pathogens and debris. pinocytosis Type of vesicle transport that occurs when the plasma membrane folds inward to form a channel, allowing dissolved substances to enter the cell. FACILITATED OSMOSIS Facilitated Osmosis describes the diffusion of water molecules across a selectively permeable membrane from an area of higher concentration to an area of lower concentration. SALTWATER FISH VS. FRESHWATER FISH? Fish cells, like all cells, have semi-permeable membranes. Eventually, the concentration of "stuff" on either side of them will even out. A fish that lives in salt water will have somewhat salty water inside itself. Put it in the freshwater, and the freshwater will, through osmosis, enter the fish, causing its cells to swell, and the fish will die. What will happen to a freshwater fish in the ocean? OSMOSIS Imagine you have a cup that has 100ml water, and you add 15g of table sugar (sucrose, C12H22O11) to the water. The sugar dissolves and the mixture that is now in the cup is made up of a solute (the sugar), that is dissolved in the solvent (the water). The mixture of a solute in a solvent is called a solution. Imagine now that you have a second cup with 100ml of water, and you add 45 grams of sucrose to the water. Just like the first cup, the sugar is the solute, and the water is the solvent. But now you have two mixtures of different solute concentrations. In comparing two solutions of unequal solute concentration, the solution with the higher solute concentration is hypertonic, and the solution with the lower concentration is hypotonic. Solutions of equal solute concentration are isotonic. The first sugar solution is hypotonic to the second solution. The second sugar solution is hypertonic to the first. You now add the two solutions to a beaker that has been divided by a selectively permeable membrane. The pores in the membrane are too small for the sugar molecules to pass through, but are big enough for the water molecules to pass through. The hypertonic solution is on one side of the membrane and the hypotonic solution on the other. The hypertonic solution has a lower water concentration than the hypotonic solution, so a concentration gradient of water now exists across the membrane. Water molecules will move from the side of higher water concentration to the side of lower concentration until both solutions are isotonic. What if the two solutions being compared are on either side of a cell membrane? A hypertonic solution is one having a larger concentration of a substance on the outside of a cell than is found within the cells themselves. A hypotonic solution contains a lesser concentration of impermeable solutes outside the cell compared to within the cell. Osmosis is the diffusion of water molecules across a selectively permeable membrane from an area of higher concentration to an area of lower concentration. Water moves into and out of cells by osmosis. If a cell is in a hypertonic solution, the solution has a lower water concentration than the cell cytosol does, and water moves out of the cell until both solutions are isotonic. Cells placed in a hypotonic solution will take in water across their membrane until both the external solution and the cytosol are isotonic. A cell that does not have a rigid cell wall (such as a red blood cell), will swell and lyse (burst) when placed in a hypotonic solution. Cells with a cell wall will swell when placed in a hypotonic solution, but once the cell is turgid (firm), the tough cell wall prevents any more water from entering the cell. When placed in a hypertonic solution, a cell without a cell wall will lose water to the environment, shrivel, and probably die. In a hypertonic solution, a cell with a cell wall will lose water too. The plasma membrane pulls away from the cell wall as it shrivels, a process called plasmolysis. Animal cells tend to do best in an isotonic environment, plant cells tend to do best in a hypotonic environment. This is demonstrated in Figure below. Unless an animal cell (such as the red blood cell in the top panel) has an adaptation that allows it to alter the osmotic uptake of water, it will lose too much water and shrivel up in a hypertonic environment. If placed in a hypotonic solution, water molecules will enter the cell causing it to swell and burst. Plant cells (bottom panel) become plasmolyzed in a hypertonic solution, but tend to do best in a hypotonic environment. Water is stored in the central vacuole of the plant cell. OSMOTIC PRESSURE When water moves into a cell by osmosis, osmotic pressure may build up inside the cell. If a cell has a cell wall, the wall helps maintain the cell's water balance. Osmotic pressure is the main cause of support in many plants. When a plant cell is in a hypotonic environment, the osmotic entry of water raises the turgor pressure exerted against the cell wall until the pressure prevents more water from coming into the cell.At this point the plant cell is turgid (Figure below). The effects of osmotic pressures on plant cells are shown in Figure above. The central vacuoles of the plant cells in this image are full of water, so the cells are turgid. Osmosis can be seen very effectively when potato slices are added to a high concentration of salt solution (hypertonic). The water from inside the potato moves out of the potato cells to the salt solution, which causes the potato cells to lose turgor pressure. The more concentrated the salt solution, the greater the difference in the size and weight of the potato slice after plasmolysis. The action of osmosis can be very harmful to organisms, especially ones without cell walls. For example, if a saltwater fish (whose cells are isotonic with seawater), is placed in fresh water, its cells will take on excess water, lyse, and the fish will die. Another example of a harmful osmotic effect is the use of table salt to kill slugs and snails. CONTROLLING OSMOSIS Organisms that live in a hypotonic environment such as freshwater, need a way to prevent their cells from taking in too much water by osmosis. A contractile vacuole is a type of vacuole that removes excess water from a cell. Freshwater protists, such as the paramecia shown in Figure below, have a contractile vacuole. The vacuole is surrounded by several canals, which absorb water by osmosis from the cytoplasm. After the canals fill with water, the water is pumped into the vacuole. When the vacuole is full, it pushes the water out of the cell through a pore. Other protists, such as members of the genus Amoeba, have contractile vacuoles that move to the surface of the cell when full and release the water into the environment. The contractile vacuole is the star-like structure within the paramecia. SUMMARY: Osmosis is the diffusion of water molecules across a semipermeable membrane and down a concentration gradient. They can move into or out of a cell, depending on the concentration of the solute. CITED REFERENCES 1. Bianconi E, Piovesan A, Facchin F, Beraudi A, Casadei R, Frabetti F, Vitale L, Pelleri MC, Tassani S, Piva F, Perez-Amodio S, Strippoli P, Canaider S. Ann. An estimation of the number of cells in the human body. Retrieved March 14, 2014 from https://www.ncbi.nlm.nih.gov/pubmed/23829164(link is external). 2. Joshua Haussler, Karla Moeller. (2015, December 19). Concentration Gradients. ASU - Ask A Biologist. Retrieved from https://askabiologist.asu.edu/concentration-gradients. 3. Harwood, et.al.2020. Ck-12.Active Transport.[online]Available at: 4. CK-12.2020.Facilitated Diffusion - Advanced.[online]Available at: https://www.ck12.org/book/ck-12-biology-advanced-concepts/section/3.28/ 5. CK-12.2020.Exocytosis and Endocytosis. [online]Available at: https://www.ck12.org/biology/exocytosis-and-endocytosis/lesson/Exocytosis- and-Endocytosis-BIO/ 6. CK-12.2020. Osmosis-advanced.[online]Available at: https://www.ck12.org/c/biology/osmosis/lesson/Osmosis-Advanced-BIO-ADV /?referrer=concept_detail ADDITIONAL REFERENCE Belardo. G.M. General Biology. 2015. OpenStax College. WIKI PARTS OF ANIMAL AND PLANT CELL This WIKI contains the definition, description and parts of animal and plant cells. ---------------------------------------------------------------------------------------------------- Plant and animal cells have several differences and similarities. For example, animal cells do not have a cell wall or chloroplasts but plant cells do. Animal cells are mostly round and irregular in shape while plant cells have fixed, rectangular shapes. Plant and animal cells are both eukaryotic cells, so they have several features in common, such as the presence of a cell membrane, and cell organelles, like the nucleus, mitochondria and endoplasmic reticulum. ANIMAL CELL DIAGRAM