Cell Biology - Class VI Membrane Traffic PDF
Document Details
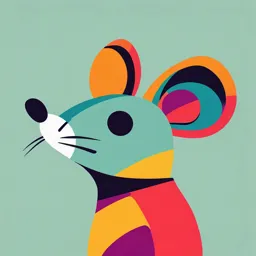
Uploaded by VirtuousSerpentine7510
University of Haifa
Prof’ Elah Pick
Tags
Summary
This document is a lecture or class notes on cell biology, specifically focusing on membrane traffic. It covers protein expression, vesicle transport, and other related cellular processes. It includes figures and links to external resources.
Full Transcript
Cell Biology – Class VI Prof’ Elah Pick Membrane traffic 1 חלבונים :ביטוי ותהליכי מבואות ונדבכים במאה מבנה הממברנה הביולוגית...
Cell Biology – Class VI Prof’ Elah Pick Membrane traffic 1 חלבונים :ביטוי ותהליכי מבואות ונדבכים במאה מבנה הממברנה הביולוגית הבשלה; תפקודם של עקות ובקרת האיכות של ה20-21 חלבונים ,זמן חיים, חלבונים מודיפיקציות מותם של חלבונים: תנועה של חלבונים מבנה הגרעין ועיצוב דגרדציה ,בקרת איכות שלד התא ותנועה של מאתר תרגומם אל הכרומטין; הכפלת התא על ידי סילוק תאים האברונים השונים הדנ"א ובקרת ההכפלה חלבונים המשתית החוץ תאית (***שיעור ללמידה מחזור התא ,בקרת מותם של תאים עצמית מלווה שיעור ה"צ'ק פוינט" מוקלט מלווה במצגת, ושתי עבודות להגשה) 2 Literature * (Additional literature is indicated in the slides) Chapter 14 3 Exocytosis and endocytosis Figure 13–1 Exocytosis and endocytosis. 4 The secretory pathway leads outward from the ER to the Golgi apparatus and cell surface Figure 13–2 Vesicle transport. 5 Proteins must pass through the ER before being sent to the Golgi before secretion A lesson from S. Cerevisiae mutant strains - 6 Figure 8–51 Using genetics to determine the order of function of genes. Figure 14.1 Overview of the secretory and endocytic pathways of protein sorting. PM ER 7 A “road map” of the secretory and endocytic pathways Figure 13–3 A “road map” of the secretory and endocytic pathways. 8 Clathrin-coated, COPI-coated, COPII- coated, and retromer-coated Figure 13–4 Electron micrographs of clathrin-coated, COPI- coated, COPII-coated, and retromer-coated vesicles. 9 cryo-electron tomography (cryo-ET) 10 https://elifesciences.org/articles/32493 Yury S Bykov ET AL. elife 2017 atomic electron tomography (AET) 11 Miao et al. 2016, Science Cryo-EM movie: https://www.youtube.com/watch?v=Qq8DO-4BnIY 12 The nobel prize of 2017 https://www.youtube.com/watch?v=026rzT Xb1zw 13 ALPHAFOLD https://www.youtube.com/watch?v=gg7Wju Fs8F4 14 I. Coated vesicle – Clathrin coated vesicles as an example Figure 13–7 Clathrin-coated pits and vesicles. This * This rapid-freeze, deep-etch electron micrograph shows numerous clathrin-coated pits and vesicles on the inner surface of the plasma membrane of cultured fibroblasts. The cells were rapidly frozen in liquid helium, fractured, and deepetched to expose the cytoplasmic surface of the plasma membrane. 15 The assembly of a clathrin coat drives vesicle formation Figure 13–5 Use of different coats for different steps in vesicle traffic. 16 17 From the following article: Molecular model for a complete clathrin lattice from electron cryomicroscopy Alexander Fotin, Yifan Cheng, Piotr Sliz, Nikolaus Grigorieff, Stephen C. Harrison, Tomas Kirchhausen and Thomas Walz Nature 432, 573-579(2 December 2004) 18 doi:10.1038/nature03079 משושים 19 * There are 36 clathrin triskelions in the structure, which has D6 symmetry. Thus, there are three symmetry- independent triskelions (or nine symmetry-independent legs). The coloured triskelions show one choice of the 20 three independent triskelions. A specific set of transmembrane and soluble cargoes selected by adaptor proteins is packaged into a clathrin-coated transport vesicle Figure 13–8 The assembly and disassembly of a clathrin coat. 21 Phosphoinositides Mark Organelles and Membrane Domains Figure 13–9 Lipid-induced conformation switching of AP2. 22 Phosphoinositides mark organelles and membrane domains 1. The assembly of adaptor proteins on the membrane is regulated by cooperative interactions with the membrane, transmembrane cargoes, and other coat components. 2. The AP2 adaptor binds to a specific phosphorylated phosphatidylinositol lipid (phosphoinositide). 3. Upon binding, AP2 undergoes a conformational change, revealing binding sites for cargo receptors. 4. This enhances AP2's membrane binding. 5. AP2 binding induces membrane curvature, facilitating the binding of additional AP2 proteins. 6. Clathrin binding further amplifies AP2 coat assembly, leading to the formation and budding of a transport vesicle. 23 phosphoinositides mark organelles and membrane domains Figure 13–10 Phosphatidylinositol (PI) and phosphoinositides (phosphatidylinositol phosphates, or PIPs). * The interconversion of phosphatidylinositol (PI) and PIPs is highly compartmentalized: different organelles in the endocytic and secretory pathways have distinct sets of PI and PIP kinases and PIP phosphatases 24 Membrane-bending proteins help deform the membrane during vesicle formation Figure 13–12 The structure of BAR domains. * Membrane-bending proteins that contain crescent-shaped domains, called BAR domains, bind to and impose their shape on the underlying membrane Figure 13–11 The intracellular location of phosphoinositides * The PIP-binding proteins then help regulate vesicle formation 25 and other steps in the control of vesicle traffic The control of coat assembly Figure 13–13 Local actin polymerization helps drive budding of membrane vesicles. * The clathrin machinery, together with BAR-domain proteins, helps nucleate and stimulate the local assembly of actin filaments, which push on the membrane surrounding the budding vesicle and move it away 26 from the membrane. GTPases act as molecular switches https://doi.org/10.3390/ijms140918181 * GTPases cycle between an active GTP-bound state and an inactive GDP-bound state. * Guanine Exchange Factors (GEFs) activate GTPases, which in turn interact with specific effectors to mediate downstream pathways. * The intrinsic GTPase activity of these small G proteins is stimulated by GAPs, which accelerate the inactivation of the regulatory activity of the GTPases. 27 GTPases act as molecular switches Figure 3–63 Many different GTP-binding proteins function as molecular switches. * GTP-binding proteins are in their “on” conformation when GTP is bound, but they can hydrolyze this GTP to 28 GDP—which releases a phosphate and flips the protein to its “off” conformation General model for scission of coated-buds doi: 10.1126/science.1171004 * Dynamin is localized at the neck, where multiple cycles of membrane association depend on GTP exchange, while hydrolysis to the GDP-bound state leads to membrane dissociation. 29 Figure 14.19 Model for dynamin-mediated pinching off of clathrin/AP-coated vesicles. 30 Experimental Figure 14.20 GTP hydrolysis by dynamin is required for pinching off of clathrin-coated vesicles in cell-free extracts. 31 Dynamin is a monomeric GTPases controlling coat assembly Figure 13–14 The role of dynamin in pinching off clathrin-coated vesicles. 32 Dynamin is a monomeric GTPases controlling coat assembly Figure 13-12b Molecular Biology of the Cell (© Garland Science 2008) 33 2013 Nobel Prize in physiology awarded to trio for fundamental research into cellular transport 34 http://www.theguardian.com/science/video/2013/oct/07/cellular- transport-2013-nobel-prize-physiology-medicine-video II. GTPases as molecular switches: COPII coats as an example COPII coated vesicle Clathrin coated vesicle https://doi.org/10.1073/pnas.241522198 35 Monomeric GTPases control coat assembly 36vesicle. Figure 13–15 Formation of a COPII coated Monomeric GTPases control coat assembly 37vesicle. Figure 13–15 Formation of a COPII coated Few proteins arew attached to the bilayer solely by a covalently bound lipid chain—either a fatty acid chain or a prenyl group Figure 10–17 Various ways in which 38 proteins associate with the lipid bilayer. The covalent attachment of either type of lipid can help localize a water-soluble protein to a membrane Figure 10–18 Membrane protein attachment by a fatty acid chain 39 or a prenyl group. Monomeric GTPases control coat assembly 40vesicle. Figure 13–15 Formation of a COPII coated The shape and size of transport vesicles are diverse Figure 13–16 Packaging of procollagen into large tubular COPII-coated vesicles. * Two COPII coat assembly modes from cryo-electron tomography. On a spherical membrane (left), Sec23/24 proteins form patches that anchor the Sec13/31 cage. For procollagen packaging (right), specialized proteins modify the coat assembly. 41 Summary of the common between coated vesicle formation Steps: (1) Assembly of coat subunits on the membrane, during which cargo recognition and sorting takes place. (2) Maturation, during which the coat and underlying membrane acquires curvature. (3) Scission of the coated bud finally generates a coated vesicle. doi: 10.1126/science.1171004 42 Membrane active GTPases in coated vesicular transport Clathrin coats COPII coats myristoyl chain COPI coats myristoyl chain 43 doi: 10.1126/science.1171004 44 Figure 14.8 Model for the role of Sar1 in the assembly and disassembly of COPII coats. 45 How do vesicles fuse with the target membrane? ? 46 Rab Proteins Guide Transport Vesicles to Their Target Membrane Figure 13–18 The formation of a Rab5- associated patch on the endosome membrane. 47 48 Rab proteins create and change the identity of an organelle Figure 13–18 The formation of a Rab5- associated patch on the endosome 49 Rab cascades * A Rab protein can recruit another Rab, changing the organelle's identity by inactivating the first Rab and disassembling its membrane patch—this process is called a Rab cascade Figure 13–19 A model for a generic Rab cascade. 50 SNAREs Mediate Membrane Fusion Figure 13–21 Dissociation of SNARE pairs by NSF after a membrane fusion cycle. 51 SNAREs Mediate Membrane Fusion Figure 14.10 Model for docking and fusion of transport vesicles with their target membranes. 52 Interacting SNAREs Need to Be Pried Apart Before They Can Function Again Figure 13–21 Dissociation of SNARE pairs by NSF after a membrane fusion cycle. * There are at least 35 different SNAREs in an animal cell, each associated with a particular organelle in the secretory or endocytic pathway. * SNAREs exist as complementary sets, with v-SNAREs usually found on vesicle membranes and t-SNAREs usually found on target membranes. 53 How viruses enter cells? Viruses encode specialized membrane fusion proteins needed for cell entry Figure 13–22 The entry of enveloped virus s into cells. Electron micrographs showing how HIV enters a cell by fusing its membrane with the plasma membrane of the cell. (From B.S. Stein et al., Cell 49:659–668, 1987. With permission from Elsevier.) * Viruses such as the human immunodeficiency virus (HIV), which causes AIDS, bind to cell-surface receptors and then fuse with the plasma membrane of the target cell. The fusion allows the viral nucleic acid inside the nucleocapsid to enter the cytosol, where it replicates. 54 Virions come in a wide variety of shapes and sizes Figure 23–11 Examples of viral morphology. 55 virus entry strategies HIV, fuse directly with the host-cell plasma membrane to release their RNA and capsid proteins into the cytosol. Influenza virus, bind to cell-surface receptors, then, fuses with the endosomal membrane, releasing the viral RNA genome and capsid proteins into the cytosol. Poliovirus, a nonenveloped virus, induces receptor- mediated endocytosis, and then forms a pore in the endosomal membrane to extrude its RNA into the cytosol. Adenovirus, a nonenveloped virus, induces receptor- mediated endocytosis and then disrupts the endosomal membrane, releasing the capsid including its DNA genome into the cytosol. Figure 23–19 Four virus entry strategies that follow virus binding to virus receptors. ( 56 HIV uses membrane fusion proteins similar to SNAREs to enter cells * A model for the fusion process. HIV binds first to the CD4 protein on the surface of the target cell. The viral gp120 which is bound to the HIV fusion protein, mediates this interaction. A second cell surface protein (CD4) interacts with gp120. The interaction releases the HIV – allows insertion of the virus. 57 HIV require two types of receptors to enter a host cell Figure 23–18 Receptor and co-receptors for HIV. HIV require the CD4 protein as a primary receptor. Early in an infection, most of the viruses use CCR5 as a co-receptor, allowing them to infect macrophages and monocytes. As the infection progresses, mutant variants of the virus arise now use CXCR4 as a co-receptor, enabling them to infect helper T cells efficiently. 58 In summary: Vesicle Formation & Transport: Transport vesicles (spherical, tubular, or irregular) bud from coated regions of donor membranes. Coats assemble to collect specific cargo and shape the vesicle. Types of coated vesicles: Clathrin-coated: Plasma membrane, endosomes, trans Golgi network. COPI & COPII-coated: Golgi cisternae, ER to Golgi transport. Retromer-coated: Endosome to Golgi transport. Coat Structure: Inner layer: Adaptor proteins trap cargo. Outer layer: Forms a cage and deforms the membrane. Coat is shed before vesicle fusion with target membrane. Specificity of Transport: Phosphoinositides: Create binding sites for coat assembly and vesicle budding. GTPases (e.g., Sar1, ARF, Rab proteins): Regulate coat assembly/disassembly and control transport specificity. SNAREs: Complementary v-SNAREs and t-SNAREs drive membrane fusion. Rab Cascades: Rab proteins and effectors dynamically determine organelle identity and vesicle targeting. 59 “Only Proteins That Are Properly Folded and Assembled Can Leave the ER” (see class IV) Transport from the ER through the Golgi apparatus 60 Proteins leave the ER in COPII-coated transport vesicles Figure 13–15 Formation of a COPII coated vesicle. 61 The monomeric GTPase Sar1 control coat assembly 62vesicle. Figure 13–15 Formation of a COPII coated Proteins destined for the Golgi apparatus or beyond are first packaged into COPII-coated transport vesicles at the ER Figure 13–23 The recruitment of membrane and soluble cargo 63 molecules into ER transport vesicles. Vesicular Tubular Clusters Mediate Transport from the ER to the Golgi Apparatus ….. And so, on Figure 13–24 Homotypic membrane fusion. * After budding from ER exit sites, transport vesicles shed their coat and fuse with each other. * Fusion of membranes from the same compartment is called homotypic fusion. * Fusion of membranes from different compartments is called heterotypic fusion. 64 when ER-derived vesicles fuse with one another are called vesicular tubular clusters Figure 13–25 Vesicular tubular clusters. 65 How are escaped proteins returned to the ER?? * Golgi-derived COPI-coated vesicles are involved in several vesicular transport steps, including bidirectional transport within the Golgi and recycling to the ER 66 The Retrieval Pathway to the ER Uses Sorting Signals ER membrane proteins use a KKXX sequence to bind COPI coats and are packaged into vesicles for retrograde transport. Soluble proteins have a KDEL sequence that binds to the KDEL receptor, enabling their return to the ER in COPI-coated vesicles. v- and t-SNAREs and cargo receptors use this pathway for ER retrieval. Genetic Engineering Impact: Removing or adding retrieval signals affects protein secretion or accumulation in the ER. 67 Figure 14.11 Vescle-mediated protein trafficking between the ER and cis-Golgi. 68 Many Proteins Are Selectively Retained in the Compartments in Which They Function Figure 13–26 Retrieval of soluble ER resident proteins. 69 Walter Nickel, Britta Brügger, Felix T. Wieland 70 Journal of Cell Science 2002 115: 3235-3240; https://jcs.biologists.org/content/115/16/3235 Walter Nickel, Britta Brügger, Felix T. Wieland 71 Journal of Cell Science 2002 115: 3235-3240; An example for a soluble protein that leave the ER and must return 72 73 Newly synthesized polypeptides are inserted into the ER and transported to the secretory pathway. Immature (unfolded or misfolded) proteins due to intrinsic defects (e.g., mutated sequence, missing subunits) or extrinsic insults (e.g., ischemia, malnutrition, hypoxia and toxic substances) accumulate in the ER, inducing the ER stress response or unfolded protein response (UPR). 74 https://www.frontiersin.org/articles/10.3389/fnmol.2017.00222/full Newly synthesized polypeptides are inserted into the ER and transported to the secretory pathway. Immature (unfolded or misfolded) proteins due to intrinsic defects (e.g., mutated sequence, missing subunits) or extrinsic insults (e.g., ischemia, malnutrition, hypoxia and toxic substances) accumulate in the ER, inducing the ER stress response or unfolded protein response (UPR). 75 Q: What type of cell synthesizes antibodies? A: Lymphocyte There are two main types of lymphocytes: B cells and T cells. 1. The B cells produce antibodies that are used to attack invading bacteria, viruses, and toxins. 2. The T cells destroy the body's own cells that have themselves been taken over by viruses or become cancerous. 76 An example for a membrane-bound protein that leave the ER and must return 77 Interacting SNAREs Need to Be Pried Apart Before They Can Function Again Figure 13–21 Dissociation of SNARE pairs by NSF after a membrane fusion cycle. * There are at least 35 different SNAREs in an animal cell, each associated with a particular organelle in the secretory or endocytic pathway. * SNAREs exist as complementary sets, with v-SNAREs usually found on vesicle membranes and t-SNAREs usually found on target membranes. 78 Sec22 is a v-SNARE involved in vesicular trafficking between the ER and the Golgi apparatus. Schematic representation of Sec22 with N-terminal Longin domain, coiled-coil region (CC), low-complexity region (LC), and its C-terminal transmembrane domain (TMD). 79 V- SNAREs are recycled through COP-I vesicles DOI: 10.3390/cells12111547 80 V- SNAREs are recycled through COP-I vesicles https://doi.org/10.1016/S0167-4889(98)00045-7 81 Role of Sec22 during anterograde and retrograde trafficking along with compatible SNAREs and associated factors like COP-I and COP-II, Rab-GTPase, and tethers 82 The Golgi Apparatus The Golgi apparatus, visualized by silver stains, was one of the first organelles described by light microscopy. It consists of flattened membrane-bound compartments called cisternae, typically arranged in stacks of 4-6, though some unicellular flagellates may have over 20. In animal cells, tubular connections link cisternae across stacks, forming a single complex near the nucleus and centrosome, dependent on microtubules. Depolymerization of microtubules causes the Golgi to reorganize into individual stacks distributed throughout the cytoplasm, near ER exit sites. Some cells, like plant cells, have hundreds of individual Golgi stacks. 83 The Golgi Apparatus Figure 13–27 The Golgi apparatus 84 Localization of the Golgi apparatus in animal and plant cells The Golgi in a cultured fibroblast stained with a fluorescent The Golgi apparatus in a plant cell that is expressing a fusion antibody that recognizes a Golgi resident protein. protein consisting of a resident Golgi enzyme fused to GFP Figure 13–28 Localization of the Golgi 85 cells. apparatus in animal and plant Molecular compartmentalization of the Golgi apparatus unstained Nucleoside diphosphate Functional differences between the cis, medial, and trans subdivisions of the Golgi apparatus by localizing the enzymes involved in processing N-linked oligosaccharides in distinct regions of the organelle. Osmium, preferentially labels the cisternae of the cis compartment. Osmium Acid Nucleoside diphosphatase is found in the phosphatase trans Golgi cisternae. Acid phosphatase is found in the trans Golgi network. * Note that usually more than one cisterna is stained. The enzymes are therefore thought to be highly enriched rather than precisely localized to a specific cisterna. 86 Transport of VSVG-GFP Experimental Figure 14.2 Protein transport through the secretory pathway can be visualized by fluorescence microscopy of cells producing a GFP-tagged membrane protein. * This assay uses a temperature-sensitive mutant of the viral envelope protein VSVG fused to GFP. After transfection and incubation at 41oC, the protein is unfolded and thus retained in the ER. At 0 time the cells are transferred to 32oC, resulting in renaturing and transport, first to the Golgi, later to the plasma membrane 87 Golgi Matrix Proteins Help Organize the Stack Golgi apparatus architecture relies on the microtubule cytoskeleton and Golgi matrix proteins. Golgi Reassembly and Stacking Proteins (GRASPs) provide structural integrity by scaffolding between adjacent cisternae. Golgins form long, stiff tentacles (100–400 nm) with coiled-coil domains and hinge regions, extending from the Golgi stack. Different golgin family members localize to distinct Golgi regions and bind specific Rab proteins. Golgins likely act as tethers, guiding transport vesicles to appropriate Golgi stack regions based on their Rab protein markers. Figure 13–37 A model of golgin function. 88 The intracellular localization of the Golgi complex Is an outcome of it interaction with the MT cyto-skeleton + - MTOC Microtubule Organizing Center 89 + - MTOC Antibody that recognizes the Golgi 90 Transport Through the Golgi Apparatus Occurs by Multiple Mechanisms Figure 13–36 Two mechanisms explaining the organization of the Golgi apparatus and how proteins move through it. It 91 Molecular compartmentalization of the Golgi apparatus Figure 13–30 Oligosaccharide processing in Golgi compartments. 92 Oligosaccharide Chains Are Processed in the Golgi Apparatus Figure 13–31 The two main classes of asparagine-linked (N-linked) oligosaccharides found in mature mammalian glycoproteins. 93 Oligosaccharide chains are processed in the Golgi apparatus Figure 13–32 Oligosaccharide processing in the ER and the Golgi apparatus. 94 Proteoglycans Are Assembled in the Golgi Apparatus Figure 13–33 N- and O-linked glycosylation. In each case, only the single sugar group that is directly attached to the protein chain is shown. * O-linked glycosylation is catalyzed by glycosyl transferases that use the sugar nucleotides in the lumen of the Golgi apparatus to add sugars to a protein. * Usually, N-acetylgalactosamine is added first, followed by a variable number of additional sugars. 95 What is the purpose of Glycosylation? Limited flexibility of sugar chains restricts the approach of other macromolecules to a glycoprotein’s surface, making it more resistant to proteolytic enzyme digestion. A large family of genetic human diseases known as congenital disorders of glycosylation (CDG) is caused by inherited mutations in individual enzymes involved in glycan modification of proteins and lipids. 96 Congenital Disorders of Glycosylation (CDG) A group of genetic disorders that affect the Esotropia, long philtrum, large process of glycosylation hypoplastic or dysplastic ears, hypotonic facies, down slanting palpebral fissures Most common sub-type: PMM2-CDG (formerly CDG-Ia). Genetic defect: Loss of phosphomannomutase 2 (PMM2), the enzyme responsible for converting Profile shows a prominent chin, mannose-6-phosphate to mannose-1- which becomes more pronounced with age phosphate. Lethality: 20% of patients die ~ 4 YO Survival: Survivors generally have a good life expectancy, with the oldest patient in her late 40s. Treatment: Impossible to cure. Focuses on symptom management and prevention of DOI:10.21037/atm.2018.10.45 complications. 97 One another purpose for Glycosylation Glycosylation of proteins is vital for protective barriers like the mucus coat in lung and intestinal cells, defending against pathogens. Lectins, including selectins, are crucial for cell adhesion. They mediate transient adhesion interactions, crucial for the migration of blood cells, especially white blood cells, during immune responses. By controlling the binding of white blood cells to the endothelial cells lining blood vessels, selectins enable these cells to exit the bloodstream and enter tissues, a key step in immune surveillance and response. P-selectin, which attaches to the actin Selectins and integrins cytoskeleton through mediate the cell–cell adaptor proteins adhesions required for a white blood cell to migrate out of the bloodstream into a tissue. Figure 19–28 The structure and function of selectins. 98 Transport from the trans Golgi network to the cell exterior and endosomes 99 Golgi Matrix Proteins Help Organize the Stack Golgi structural relies on microtubules and cytoplasmic Golgi matrix proteins. Structure integrity and formation between adjacent cisternae depends on Golgi Reassembly and Stacking Proteins (GRASPs). Fliamentous proteins named Golgin are localize to different regions of the Golgi. Different members of the Golgin family of proteins are localized to different regions of the Golgi apparatus. Golgins act as tethers, selecting transport vesicles for engagement with the Golgi. Figure 13–37 A model of golgin function 100 Cell cycle regulation of Golgi membrane dynamics During the cell cycle Golgi fragments evenly distributed to daughter cells. Phosphorylation by mitotic protein kinases causes fragmentation and dispersion while Dephosphorylation reassembles the Golgi stack. DOI: 10.1016/j.febslet.2009.10.077 101 From the TGN to the cell exterior (exocytosis) and endosomes 102 From the TGN to the cell exterior and endosomes In secreting cells: 1 M6P receptor proteins 2 the default pathway 3 Figure 13–38 The three best-understood pathways of protein sorting in the trans Golgi network. hormones, neurotransmitters or digestive enzymes 103 1 A mannose 6-phosphate receptor sorts lysosomal hydrolases in the TGN Figure 13–39 The structure of mannose 6-phosphate on a lysosomal hydrolase. 104 A mannose 6-phosphate receptor sorts lysosomal hydrolases in the TGN Figure 13–40 The transport of newly synthesized lysosomal hydrolases to endosomes. 105 A mannose 6-phosphate receptor sorts lysosomal hydrolases in the TGN Figure 13–41 The recognition of a lysosomal hydrolase. 106 Defects in the GlcNAc phosphotransferase cause a ‘Lysosomal Storage Disease’ in Humans Lysosomal Storage Diseases (LSDs) Genetic defects in lysosomal hydrolases. Accumulation of undigested substrates in lysosomes. Severe pathological effects, especially in the nervous system. Mutation in Structural Genes Mutation affects specific lysosomal hydrolases. Example: Hurler's disease (defective glycosaminoglycan breakdown). Inclusion-Cell Disease (I-Cell Disease) Rare, severe inherited metabolic disorder. Almost all hydrolytic enzymes are missing in lysosomes. Accumulation of substrates forms large inclusions in cells. Affects all organ systems, skeletal integrity, and mental development. Life expectancy: Rarely beyond 6-7 years. 107 I-cell disease is due to a single gene defect and, like most genetic enzyme deficiencies, it is recessive * People deficient in the phosphotransferase enzyme develop I-cell disease, characterized by the absence of eight acid hydroloases normally present in lysosomes. * These enzymes lack phosphor mannose(M6P) and are found in blood and urine. 108 2 Secretory Vesicles Bud from the Trans Golgi Network Figure 13–42 The formation of secretory vesicles. * The immature secretory vesicles resemble dilated trans Golgi cisternae that have pinched off from the Golgi stack. * At the next step, clathrin-coated transport vesicles bud from the mature secretory vesicles, and got back to the TGN. 109 בדף הבא השיטה Figure 9.29 Gold particles coated with protein A are used to detect an antibody-bound protein by transmission electron microscopy. 110 Secretory Vesicle Maturation and Rapid Exocytosis Immature vesicles sometimes fuse and become more acidic due to increasing V-type ATPases. Acidification condenses the secretory protein aggregate inside. Excess membrane is returned to the trans-Golgi network (TGN). Mature vesicles are densely packed, allowing rapid release of large amounts of material via exocytosis when triggered Figure 13–43 Exocytosis of secretory vesicles. 111 Precursors of secretory proteins are sometimes proteolytically processed during the formation of secretory vesicles Figure 13–44 Processing pathways for the prohormone polyprotein proopiomelanocortin. 112 3 Secretory Vesicles Wait Near the Plasma Membrane Until Signaled to Release Their Contents Figure 13–45 Exocytosis of synaptic vesicles. 113 Secretory Vesicles Wait Near the Plasma Membrane Until Signaled to Release Their Contents Figure 13–46 The formation of synaptic vesicles in a nerve cell. 114 Scale models of a brain presynaptic terminal and a synaptic vesicle Figure 13–47 Scale models * Each synaptic vesicle membrane contains 7000 phospholipid molecules and 5700 cholesterol molecules. Each also contains close to 50 different integral membrane protein molecules 115 Experimental Figure 14.23 Proteolytic cleavage of proinsulin occurs in secretory vesicles after they have budded from the trans-Golgi network. 116 Figure 14.24 Proteolytic processing of proproteins in the constitutive and regulated secretion pathways. 117 118 Figure 13-68 Molecular Biology of the Cell (© Garland Science 2008) Regulated exocytosis – mast cells has been activated to secrete Histamine Figure 13-68 Molecular Biology of the Cell (© Garland Science 2008) 119 Another kind of sorting: Polarized Cells Direct Proteins from the Trans Golgi Network to the Appropriate Domain of the Plasma Membrane Transport vesicles Transcytosis Figure 13–49 Two ways of sorting plasma membrane proteins in a polarized epithelial cell. 120 Endocytosis 121 Transport into the cell from the PM - endocytosis Figure 13–50 Endosome maturation: the endocytic pathway from the plasma membrane to lysosomes. 122 The endocytic part of the cycle often begins at clathrin- coated pits Figure 13–51 The formation of clathrin-coated vesicles from the plasma membrane. 123 Cells Use Receptor-mediated Endocytosis to Import Selected Extracellular Macromolecules: low-density lipoproteins (LDLs) as example Most cholesterol is transported in the blood as “cholesterol esters” in the form of lipid–protein particles known as low-density lipoproteins (LDLs). LDLs architecturally, resemble lipid droplets bearing a core of triacylglycerol, free cholesterol, and cholesterol esters. Figure 13–53 A low-density lipoprotein (LDL) particle. 124 Most cholesterol in the blood is cholesteryl-ester in lipid– protein particles (LDL) This area is associate with coated pits 125 In the early endosome, the LDL receptor discharges LDL and recycled back to PM for reuse Figure 13–54 The receptor-mediated endocytosis of LDL. 126 Experimental Figure 14.28 Pulse-chase experiment demonstrates precursor-product relations in cellular uptake of LDL. 127 Figure 14.29 Endocytic pathway for internalizing low-density lipoprotein (LDL). 128 Figure 14.30 Model for pH-dependent binding of LDL particles by the LDL receptor. 129 Cells Use Receptor-mediated Endocytosis to Import Selected Extracellular Macromolecules: transferrin receptor as example Transferrin is a soluble protein that carries iron in the blood. In early endosomes (low pH), iron release from transferrin, while iron-free apotransferrin remains bound to the receptor. The receptor–apotransferrin complex is then recycled back to the plasma membrane, where apotransferrin dissociates at neutral pH. Transferrin continuously shuttles between the extracellular fluid and early endosomes, delivering iron to the cell without entering lysosomes, supporting cell growth and proliferation. DOI: 10.4236/jct.2012.34039 130 Figure 14.31 The transferrin cycle, which operates in all growing mammalian cells. 131 Next subject 132 Figure 12–2 An electron micrograph of part of a liver cell seen in cross section. Lysosome is an endpoint The Lysosome Lysosomes contain digestive enzymes that degrade intracellular organelles, macromolecules and endocytosed particles. It includes enzymes that function best at the low pH (∼5). (unlike in the cytosol that is close-to- neutral pH (∼7.2) ). Its membrane include H+ pumps that maintain the low pH, also found in endosomes and secretory vesicles. This V-type H+ pumps use the energy of ATP hydrolysis to pump H+ to acidify the interior of Figure 13–62 Lysosomes. these organelles Lysosome-like organelles Plant Budding yeast Mammalians Figure 13–65, 66 The plant-cell vacuole. How to enrich lysosomes? Figure 8–5 Cell fractionation by centrifugation. Endosomes fuse with one another and with lysosomes to form endolysosomes, which degrade their contents Figure 13–64 A model for lysosome maturation Endosomes fuse with one another and with lysosomes to form endolysosomes, which degrade their contents Figure 13–50 Endosome maturation: the endocytic pathway from the plasma membrane to lysosomes. ESCRT Protein Complexes Mediate the Formation of Intralumenal Vesicles in MVBs Figure 13–58 Cryo-electron microscopy (cryo-EM) tomogram of multivesicular bodies in cultured human lymphocytes. * As endosomes mature, patches of their membrane invaginate into the endosome lumen and pinch off, forming intralumenal vesicles, seen in the electron microscope, known as called multivesicular bodies (MVBs) * MVBs carry proteins that to be degraded 140 Once delivered to the endosomal membrane: Membrane proteins are tagged with ubiquitin on their cytosolic domains for sorting. Ubiquitin tags guide proteins into clathrin-coated vesicles at the plasma membrane. At the endosomal membrane, ubiquitin tags are recognized by ESCRT complexes.ESCRT-0, -I, -II, and -III bind sequentially to mediate sorting into intralumenal vesicles.’ Invaginations requires PI(3)P, produced by lipid kinase phosphorylation of phosphatidylinositol. ESCRT complexes need PI(3)P and ubiquitylated cargo for membrane bindingbefore bending the membrane to facilitate vesicle formation. Figure 13–60 Sorting of endocytosed membrane proteins into the intralumenal vesicles of a multivesicular body. 141 ESCRT function ESCRT complexes 1 cytokinesis 2 internal budding 3 virus budding 2 1 3 Figure 13–61 Conserved mechanism in multivesicular body formation, virus budding, and cytokinesis. *ESCRT complexes are thought to have originated from similar components that mediate cell-membrane deformation during cytokinesis in archaea. 142 Many receptors also possess sorting signals that guide them into the appropriate transport pathway MBVs Figure 13–56 Possible fates for transmembrane receptor proteins that have been endocytosed. MVBs carry proteins that to be degraded Figure 13–59 The sequestration of endocytosed proteins into intralumenal vesicles of multivesicular bodies. 144 חלבונים :ביטוי ותהליכי מבואות ונדבכים במאה מבנה הממברנה הביולוגית הבשלה; תפקודם של עקות ובקרת האיכות של ה20-21 חלבונים ,זמן חיים, חלבונים מודיפיקציות מותם של חלבונים: תנועה של חלבונים מבנה הגרעין ועיצוב דגרדציה ,בקרת איכות שלד התא ותנועה של מאתר תרגומם אל הכרומטין; הכפלת התא על ידי סילוק תאים האברונים השונים הדנ"א ובקרת ההכפלה חלבונים המשתית החוץ תאית (***שיעור ללמידה מחזור התא ,בקרת מותם של תאים עצמית מלווה שיעור ה"צ'ק פוינט" מוקלט מלווה במצגת, ושתי עבודות להגשה) 145 The Mechanism or ubiquitination and protein degradation – in our next class Ubiquitin proteasome Autophagy Lysosome 146