Psych Re-Write PDF: Neural Code, Brain Recordings, Memory
Document Details
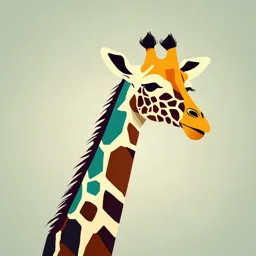
Uploaded by AchievableFriendship7196
Tags
Summary
This document is a lecture series covering topics in neuroscience, including neuronal structure, brain recordings, memory systems, and synaptic transmission. It explains membrane potential, the mechanisms underlying action potentials, and methods for analyzing brain activity, offering an introduction to the complexities of the brain.
Full Transcript
Psych Re-Write electrons = +ion; More electrons> protons= -ion Ions are important for neural signaling and naturally, flow to opposite charge ○ Positive ions → Na+, K+, Ca2+ ○ Negative ions → Cl- Electric Potential: Energy needed to move a positive ion to the positive...
Psych Re-Write electrons = +ion; More electrons> protons= -ion Ions are important for neural signaling and naturally, flow to opposite charge ○ Positive ions → Na+, K+, Ca2+ ○ Negative ions → Cl- Electric Potential: Energy needed to move a positive ion to the positive source Positive ions have more energy near their source Positive ions lose energy as they move toward negative sources Potential Difference: Difference in energy charge between two locations Current: Movement of charged particles Membrane Potential Resting Membrane Potential: Neurons voltage across its membrane = -70mV Determined by: 1. Uneven distribution of ions inside and outside of the cell → a. Inside of cell More negatively charged b. Outside of cell = More positively charged 2. RMP [maintained] by Potassium Leak Channels: While cell is at rest, [K+] leaks through potassium channels- maintains membrane potential & keeps inside of cell negative 2 Types of Channels Along Cell Membranes 1. Voltage-Gated Ion Channels: Channel opens at membrane potential threshold (spike) at -45mV a. Is triggered to open upon membrane potential threshold being met- AKA spike potential at -45mV b. Charge changes conformation of protein subunit= channel opens= Na+ flows into cell 2. Ligand Gated Ion Channels: Ligand/ neurotransmitter binds to channel, changes its conformation, and opens it 2 Outcomes When Channels [Open] 1. Depolarization: Membrane potential becomes more positive 2. Hyperpolarization: Membrane potential becomes more negative Depolarizing: Cell membrane potential becomes Hyperpolarization: Cell membrane becomes more more positive negative 1. [Na+] into cell, sodium influx 1. Na+ channels close, Na+ stops flowing in 2. Cell membrane becomes more positive 2. K+ channels open- K+ out of cell, Potassium efflux 3. Cell membrane becomes more negative Membrane Potential Threshold & Action Potentials (spikes) Membrane Potential Threshold (~-45mV): Voltage that generates action potential, and initiates action potential propagation Triggers [Voltage-Gated Ion] channels to open Action Potential: Temporary shift in neurons membrane potential caused by ions suddenly flowing in and out of cell 1. All-or-Nothing event- Action potential either fires or doesn't 2. Spike is propagated/ generated along multiple points along the axon; starts at the axon hillock leaves via axon terminal 3. At each spot, membrane potential goes from -70mV to 30mV to -70mV 3 Major Phases of Action Potential Propagation 0) Triggering Event Occurs → Depolarizes (+) cell body Causes positive ions to flow into cell body Positive charge triggers [Voltage-Gated Ion channels] to open → Na+ into cell ○ Only occurs if voltage reaches membrane potential threshold (-70mV to -45mV) 1) Depolarization → [Voltage-gated Sodium Channels] at axon hillock open Na+ flows into [axon] → Depolarizes axon at that particular location ○ ^Causes domino effect of depolarization (Na+ inflow) along the axon Signal is sent out at the axon terminal 2) Hyperpolarization → Brings cell back to resting membrane potential (-70mV) → Take out [positive charge] in cell, so that it can return to its negative resting potential 1. [Voltage gated Sodium Channels] close- Na+ stops flowing in 2. More [K+ Channels] open- K+ flows out of cell 3) Sodium Potassium Pump → Reestablishes [resting membrane potential] against concentration gradient Lecture 2, Cracking the Neural Code Neural/ Brain Recordings 2 things can be recorded from single-neurons → 1. Action Potentials (spikes) 2. Subthreshold Membrane Fluctuations: Voltage below the AP Two Forms of Recordings 1. Intracellular Recordings: Records activity inside of each cell a. Record AP + Subthreshold membrane potentials b. Pros: High spatial resolution → Can identify location of signal 2. Extracellular Recordings: Records activity near cell and of nearby cells a. Records AP + LFPs i. LFP: Summed membrane fluctuations of nearby neurons b. Cell you’re closest to = Shows largest AP Amplitude i. Further the cell is from target cell= smaller AP amplitude Extracellular Recording Devices Extracellular Recordings: Record activity near cell, and of nearby cells Records AP + LFP Cells you're closest to = Larger AP; further you are from target cell= Smaller AP 5 Types of Electrodes Used in Extracellular Recordings 1. Classical Electrode: Records a few cells at once- has few microns of metal exposed at tip 2. Matrix Electrode: Many [classical electrodes] attached a. Allows for recordings of subpopulations 3. Laminar Probe: Shows how [neural activity] differs as you go deeper in the brain a. Has multiple depth electrode contacts b. Gives info on neural activity in each layer of cerebral cortex- starting from surface 4. Utah Array: 5. Neuropixels Probe: Records thousands of neurons a. Each square of electrode records 100s of neurons at a time Classical Electrode Matrix Electrode Laminar Probe Utah Array Neuropixels probe Electrode Shape & Impedance Importance 2 Important considerations for Electrodes: 1. Size → Electrode size of [metal contact tip] determines the brain area sampled a. Smaller exposed contact tip = smaller brain area sampled= locate stimulus better- Good spatial resolution i. Smaller exposed metal contact= smaller brain area sampled → Better localized stimulus= Good Spatial Resolution 2. Electrode Impedance: How much electrode resists the flow of [electrical current]; Measure of resistance + Electrode Capacitance (ability to store charge) a. High Impedance= Easier to record AP → Small electrode b. Low Impedance: Larger electrode tip; Neurons closer to probe have greater action potential amplitude Neural Recording Methods Neural = brain/cranial Neural Recording Methods: Allow us to measure brain activity 3 Neural Recording Methods: 1. LFP 2. EEG 3. fMRI LFP LFP: Electrical signals in the brain, represent the summed membrane fluctuations of [nearby cells] High temporal resolution 2 forms of LFPs: 1. LFP From Extracellular Depth Electrode: Measure the activity of up to 1000 nearby cells a. Signal comes from neurons close to the electrode tip 2. LFP From Electrocorticography (ECoG): Records brain activity using electrodes on the surface of the brain a. Typically used for epilepsy patients to pinpoint seizure locations b. Signal is generated by activity in the superficial layers of the cerebral cortex EEG EEG: Records summed & synchronized activity of neurons that share similar [spatial orientation] → Primarily records primordial cells in the cortex [High] Temporal Resolution → Records brain activity as soon as it occurs [Poor] Spatial Resolution → Difficult to localize the source of EEG signals ○ Skull distorts EEG signal → cant record deep brains structures fMRI fMRI: Indirectly measures neural activity by [detecting changes] in blood oxygen levels (BOLD) 1. Uses MRI magnetic fields to excite hydrogen atoms in the body → Then Measures the radio frequency signals they emit a. Excitation causes BOLD levels to change 2. Reflects subthreshold membrane potentials 3. fMRI is correlated with LFPs [High] Spatial Resolution → Locates activity better [Poor] Temporal Resolution → Slower measurements, taken every 2 seconds- have lapses in recordings fMRI Spatial Resolution fMRI → High [Spatial Resolution]= Low [Temporal Resolution] Records Spatial Resolution via voxel cubes Neural/ Brain Recording Methods Neural Recording Methods: Allow us to measure brain activity, via 1. Single unit recordings 2. LFPs 3. EEG 4. fMRI Single Unit Recordings Record 1 cell Highest Spatial Resolution Invasive LFP Records summed activity of subthreshold fluctuations of 1000s of cells High Temporal Resolution EEG Records summed & synchronized activity of 1 million+ cells High Temporal Resolution Skull distorts spatial resolution fMRI Measures fluctuations in BOLD activity as an indirect measure of neural activity- of 500,000+ cells High Spatial Resolution- voxels Methods of Interpreting Neural Recordings Interpret the brain's coding of information using neural recordings: All interpretations of neural recordings analyze the spike rate! 1. Spike Rate Code: The number of spikes fired by one neuron in a given [time interval] a. Number of spikes = Reflects intensity of stimulus; More spikes= More intense stimulus b. 2. Pooled Response Code: The number of spikes fired by many neurons, combined, in a given [time interval] a. Helps reduce variability that comes from an individual neurons responses b. Provides a more stable and reliable measure of brain activity c. 3. Labeled- Line Coding: Looks at which neurons fire, and how many spikes they produce, in a given time interval a. Forms a vector (Cell 1, Cell 2) b. Spike Timing Code Spike Rate Code: The number of spikes fired by an individual neuron, in a fixed time interval Number of spikes reflects the intensity of the stimulus → More spikes= Stronger stimulus Spike Pattern Code: Focuses on the timing of spikes within a fixed interval;Each interval is divided into [smaller bins] Gives us binary code of 0s and 1s Spike-Phase Code: Relates the timing of spikes to brain network oscillations Line up spike pattern to network oscillations Decoding Neural Activity Decoding neural activity= training an algorithm on known data to recognize patterns, and predict the category of new stimuli based on brain activity Decode = Take neural response and map it back to stimulus (map response to stimulus) Spike Rate Code = EAsiest and best to decode 3 Methods of Decoding Neural Activity: 1. Pattern Classifier: Algorithm predicts the type of stimulus displayed by training a classifier the relationship between [patterns of neural activity] and displayed data 1. Training Step: A subset of data is used to “teach” the classifier a. Classifier learns the relationship between patterns of neural activity and the data displayed 2. Test Step: The classifier uses what its learned to predict the category of new, unseen neural activity data 2. N Neurons & K Images MEthod: Way to decode spike rate, by training an algorithm to recognize spike patterns of specific images Dots on graph represents the spike rates from one image presentation Decode → 1. Training Step: Algorithm is trained to recognize spike patterns that correspond to specific images 2. Test Step: When new spike data is presented, the algorithm predicts the category of the image by comparing the [new data] to the [most similar spike pattern] from the training data 3. fMRI Data: fMRI data is noisy and hard to work with → Researchers average fMRI data across [multiple trials] of the same images Creates clearer signal by minimizing random fluctuations Recording Methods Summed Signal Measures Single Unit Recordings Measures detailed information on spike rate Good spatial resolution Invasive :( LFP Measures combined activity of subthreshold membrane fluctuations of nearby cells Invasive High Temporal Resolution (can see timing well) EEG Measures electrical activity from the scalp Non invasive, portable ○ Poor spatial resolution High Temporal Resolution Requires classifier to decode brain imaging data fMRI Indirectly measures neural activity by detecting changes in BOLD Impractical, not portable Good Spatial Resolution- voxels!! Requires classifier to decode brain imaging data Neural Prosthesis Devices must have: 1. Stable, long term recordings → Record signals from many neurons over time 2. Real-Time Computational Analysis → Process neural data quickly to provide immediate feedback 3. Brain Plasticity → Brain must adapt to using prosthesis and incorporate it into typical neural processes Lecture 3, Brain Organization Brain Organization Terms NEUROSCIENCE TERM LAYPERSON SPEAK Anterior Front Posterior Back Dorsal Top Ventral Bottom Lateral Sideways Medial Middle Brain Anatomy 4 Primary Lobes in the Brain: 1. Frontal Lobe → Involved in decision making and cognition 2. Temporal Lobe → Processes auditory information 3. Occipital Lobe → Processes visual information 4. Parietal Lobe → Processes touch and spatial orientation information ++ Central Sulcus → Separates parietal and frontal lobe Sulcus and gyrus → Allow us to increase surface area of the cerebral cortex ○ Sulcus = groove; gyrus= valley Feedforward and Feedback Pathways Feedforward Pathway (post → Ant): Carry Sensory Information Directed from posterior to anterior cortical areas (back → front) Higher level information is processed anteriorly along pathway Feedback Pathway (ant → Post): Carry information about goals, attention priorities and predictions Directed from [anterior] to [posterior] regions Regulate neural activity in posterior areas → Amplify or filter sensory information based on the behavioral context Pathways for [Sensory Information] to [Primary Sensory Cortex] How senses are processed 1. Senses are processed via their corresponding sensory pathways, using feedforward or feedback pathways 2. 3 primary sensory organs → Eyes, Ears, Skins 3. Thalamus → Receives Sensory Input and directs it to the corresponding area in the sensory cortex a. First-Order Thalamic Areas: Directly receive sensory information → direct input to corresponding area in the sensory cortex b. Higher-Order Thalamic Areas: Identifies and focuses on most relevant sensory information → Amplifies relevant information Breakdown: First-Order Thalamic Areas: Directly receive sensory input and direct it to the corresponding area in the sensory cortex 1. Visual Pathway (Eyes) → Processed at [Primary Visual Cortex (V1)]- Area 17 2. Auditory Pathway (Ears) → Processed at the [Primary Auditory Cortex (A1)]- Area 41 a. Stimulus is processed multiple times along pathway before reaching the thalamus 3. Skin Pathway (Skin) → Processed at the [Primary Somatosensory Cortex (S1)] - Area 3 a. Stimulus is processed along multiple stages of the brainstem before being relayed to the thalamus Pathway from Primary Sensory Areas to Higher-Order Aeras Higher order areas → process more complex information Primary sensory areas and higher-order areas are connected via feedforward and feedback pathways First Order Thalamus: Receives sensory information Higher-Order Thalamus: Identifies and focuses on most relevant sensory information Amplifies relevant information → Allows the brain to prioritize what’s important Feedforward Pathways Sensory input → First order Thalamus → [Primary] Sensory Cortex → Higher Order Thalamus → [Secondary] Sensory Cortex → [Higher] Order Sensory Cortex Opposite for feedback pathways Parallel Pathways Across the Cerebral Cortex **Both pathways contain feedforward and feedback paths Dorsal Pathway (Top) → Where and how pathway Detects where objects are and how to interact with them Process spatial information and movement Ventral Pathway (Bottom) → What pathway Processes object features - object recognition Cerebral Cortex Breakdown Cerebral Cortex= Surface of brain Each region of CC contributes to a specific function Information flow starts at [primary areas] and moves anteriorly for higher-order processing: 1. Primary Areas- Areas for raw sensory input 2. Secondary Areas- Process data- Refine and interpret 3. Association Areas- Higher order processes; integrate data and more complex cognitive tasks Overall Trend → Higher level information is processed and represented at higher order areas Important Regions Primary Visual Cortex (V1) → Area 17 Primary Auditory Cortex (A1) → Area 41 Primary Somatosensory Cortex (S1)→ Area 3 Structural Differences Across the Cerebral Cortex Neocortex: Consists of 6 layers; different areas of the brain have different sizes of each layer, depending on their function Consists of excitatory pyramidal cells, most prominent in the brain (excitatory= depolarizes) ○ All other cells are inhibitory and hyperpolarize cells Level 4 = Receives Sensory Input → largest ○ Motor cortex does not have layer 4 COLUMNAR ORGANIZATION OF CEREBRAL CORTEX Cells in the same vertical column are interconnected and [respond] to the same types of sensory input Are repeated across the cortex; allow for efficient levels of processing ○ Cortical columns= span across all 6 cortical layers ○ Cortical minicolumns= smaller units within a column Important Layer Info 1. Layer → Sensory input area 2. Layers [2&3] → Send feedforward information to other cortical areas 3. Layer → Sends Feedforward information to subcortical areas 4. Layer → Seeds Feedback information to the thalamus and other cortical areas 5. Layer → Receives Feedback input from cortical areas and thalamus Anatomical Structure of the Brain Provides Insight into Function Structure of brain area tells about its function 3 levels of features that provide insight into structures of the brain area: Level 1: Cellular Level 1. Type of cell a. Excitatory = Depolarizes cell; activates b. Inhibitory = Hyperpolarizes; stops 2. Dendritic field a. Large dendritic field = can sample and integrate more information b. Small dendritic field = sample local populations Level 2: Circuit Level 1. Layering of cells in regions and their sizes (vertical column distribution) 2. Feedforward and feedback pathways 3. Missing connections may affect brain function Level 3: Systems Level 1. Which brain areas are connected 2. Reciprocal vs. bidirectional connections between brain areas Lecture 4, Brain Networking Basal Ganglia Basal Ganglia: Subcortical (deep) structures that regulate voluntary movements Composed of striatum Works via 2 main pathways → Direct and Indirect pathway to facilitate movement Contributes to [Action Selection] and [Reinforcement learning] Striatum: Input structure of basal ganglia Receives signals from the [cerebral cortex & thalamus] - except for primary visual and auditory cortex Helps decide which actions or movements should occur Composed of putamen and caudate nucleus ○ Bilateral structures- appear on both sides of the brain Voluntary Movement Pathways Basal ganglia helps regulate [voluntary movements] via 3 main circuits: Circuits regulate movement by regulating signals from the cortex before they reach motor output centers ○ STN = Excites GPi ○ Increased GPi= Thalamus Inhibited → = no movement ○ Thalamus inhibition= no movement 1. Direct Pathway: “GO” path- Facilitates movement → Striatum inhibits GPi, Thalamus becomes excited, excites motor cortex, movement occurs a. Striatum inhibits GPi → Reduced GPi= Removes thalamus inhibition → Thalamus becomes more active and excites motor cortex= movement occurs i. GPi= Inhibits thalamus ii. Thalamus excitation allows movement to occur 2. Indirect Pathway: “No-Go” Path- STOPS movement → Striatum inhibits GPe, STN excites GPi, movement doesn't occur a. Striatum inhibits GPe → STN becomes more active and excites GPi → GPi inhibits thalamus = movement does not occur 3. Hyperdirect Pathway: “Emergency -Brake” Path quickly suppresses movement a. Cortex sends excitatory signals to STN directly → Excites GPi → GPi inhibits thalamus = Movement stopped 3 Brain Circuits 1. Cortico-Striatal-Thalamic Loops: Processes limbic, associative, sensory and motor information a. Flow of Info = Cerebral Cortex → Striatum → Pallidum/ Nigra → Thalamus, back to CC b. Most of cerebral cortex projects onto striatum/ basal ganglia except for the primary visual and auditory cortices 2. Cortico-Cerebellar System: Plays a role in [automatic execution] after learning a skill a. Receives Efference Copies: Copies of commands the [motor] and prefrontal cortex are executing → i. Allows us to fine tune and monitor movements ii. Allow us to predict the state of the body after an action/ brace for an action like catching a ball b. Consists of motor cortex, thalamus, pontine nuclei and cerebellum 3. Cortico-Hippocampal Circuits: Hippocampus → involved in Episodic Memory and Spatial Navigation a. Episodic Memory: Storing and recalling personal events b. Spatial Navigation: Assists in forming mental maps of environment c. Consists of neocortex, thalamus, hippocampus and parahippocampal areas (parahippocampal cortex, perirhinal cortex, entorhinal cortex) Different Types of Brain Networks 3 TYPES OF BRAIN NETWORKS: 1. Regular Network: Every node is connected to its nearest neighbors in an orderly fashion a. Pro = specialized local clusters b. Con = difficult to transmit info to other sides of network 2. Random Network: Random connections between nodes a. Pros = can transmit info to other sides of network very fast b. Con = doesn't have specialized clusters 3. Small-World Networks: Some short and long range connection a. Has specialized clusters b. Can transmit information across the network very fast c. Brain = small world network Network Features and Measures 1. Node Degree: Number of connections a node has to other nodes a. More Connections= Higher Node degree 2. Path Length:Minimum number of connections (edges) it takes to go from [one node] to another a. Short path length= info travels faster 3. Clustering Coefficient/ Modules: Number of connections that exist between the nearest neighbors of a node as a proportion of the max number of possible connections a. Module= Subset of nodes with high within-module connectivity, and low inter-module connectivity Rich Club Architecture Rich Node: Has high node degree; Node with lots of connections High node degree= Hub for connections = Rich node Rich Club: Rich nodes connected to each other Rich Club ORganization: Rich nodes more likely to form clusters with one another Brain Connectivity Brain Connectivity: Ways different brain regions interact 2 Ways to Measure Brain Activity: 1. Anatomical Connections: Physical connections between neurons, through axons a. Measure via → 1. Tracer Studies: Inject tracer molecule into brain; Tracer physically moves along axons to reveal the [physical connectivity] between neurons - invasive 2. Diffusion MRI: Measures water diffusion in white matter a. Water moves along axons; diffusion shows directionality of pathways - noninvasive b. Identify hubs via DTI, white matter tracts 2. Functional Connections: Holds that Brain regions that show synchronized/ correlated neural activity are connected- even if not directly connected by axons a. If two areas are active together they likely communicate [directly] via [white matter], or indirectly through other regions b. Measure via → i. Neural Recording methods → Spike rate, LFPs, EEG, fMRI ii. Statistical Measures → Correlations between two measures 1. Two brain regions oscillate at the same frequency= connected c. Identify hubs via fMRI, BOLD synchronization Hubs: Highly connected areas in the brain that play a key role in communication Anatomical and Functional Connections utilize the same hubs Hubs= Precuneus, Posterior Cingulate Cortex, Anterior Cingulate Cortex, Superior Frontal Cortex, Insular Cortex, Lateral Parietal Cortex, Thalamus HUBS IDENTIFICATION METHODS OF ANATOMICAL AND FUNCTIONAL CONNECTIONS 1. Anatomical (physical) Connection Hubs → Identified via DTI DTI: Helps identify hubs by mapping how white matter tracts connect different brain regions 2. Functional Connection Hubs → Identified via fMRI BOLD Signals a. fMRI: Identify connected brain regions by measuring their synchronized activity → i. Brain regions show synched activity= functionally connected 1. Parcelate (divide) brain into regions of interest 2. Measure BOLD signals of brain using fMRI, calculate correlation btw ROI 3. Repeat correlation for all possible pairs Perturbed Brain Networks SCHIZOPHRENIA People with schizophrenia have different brain activity 1. More Connections to [area 37] → Overactive vision/ visual hallucinations 2. Less Connections to [area 44] → Disorganized speech 3. Healthy brains most connected and highest region= Area 44; Schizophrenia brain highest region = 37 a. Accounts for excess of visual hallucinations ALZHEIMERS Alpha-beta protein build up disrupts cellular activity → [Critical hubs] often taken out by protein build-up COMA Disorder of consciousness Have reduced connectivity in areas of [consciousness]- may explain why ppl are asleep Lecture 5, Receptive Fields and Topographic Maps Receptive Field Receptive Field: 1. Area/ size from which information is received 2. Part of the world a [neuron] responds to 3. Tells us where or what an object is, depending on RF size a. Small RF = Cover small area → identify what an object is, precisely localizes stimulus, cant detect other stimuli in broader area b. Large RF= Cover large area → Has Position Invariance, detects object in RF- can't localize exactly where 3 SENSOR SIZES OF RECEPTIVE FIELDS 1. Sensor with [BIG] Receptive Field → Good for Object Recognition a. Has Position Invariance: Can recognize objects, regardless of location in RF b. Detects objects in space- can't locate exactly where c. Built by combining small receptive fields i. Allows higher-level neurons to integrate information from multiple sources to detect more complex patterns 2. Sensor with [SMALL] Receptive Field → Good for Localizing Stimulus a. Identifies what object is- detects fine details b. Precisely located stimulus at exact spot i. If same object appears in dif part of visual field, a dif set of neurons would respond c. Found early in [sensory pathways] and near [sensory organs] – detects fine details 3. Sensor with [INTERMEDIATE] Receptive Field → Medium cover area a. Detects stimulus in 1 row- cant detect stimulus elsewhere Topographic Map: Receptive fields of sensory organs are organized into topographic maps, allowing us to 1. Localize and identify big and small objects 2. Identify objects regardless of position- position invariance Neurons x Receptive Fields Neurons Receptive Field: Specific area of the world that triggers a response from its corresponding [neuron] Different neurons respond to different parts of the body → neurons are activated if their corresponding body part receives a stimulus (light, touch, etc. ) Building Representations of our Environment at Multiple Scales Our brain represents the world at different levels of detail, using neurons with both [big] and [small] RFs Neurons with SMALL RF → Detect detailed features of objects, allow for sharpness 1. Each neuron responds to small, specific spaces- detects fine details a. Neurons have high sensitivity 2. Combine all of neuron's responses to create a representation of what we see Neurons with LARGE RF → Identify object, regardless of position in RF (position invariance) 1. Sum inputs of neurons to create large receptive field a. Nearby neurons responding to the same type of stimuli, [sum input] into [one neuron], creating a large receptive field i. Ex. 4 neurons detect smell stimuli- all send information to one other neuron ii. If 1 of the 4 neurons detects stimuli, its information is still sent to the master neuron 2. Detect information from a larger scope; help process big picture info **Small and Large Receptive Fields communicate via feedforward and feedback pathways** 2 Important Neural Receptive Fields: 1. Somatosensory Receptive Field (touch): Area of the body that a [neuron] responds to when stimulated by [touch] a. Small Somatosensory RFs → Found in [fingertips] i. Detect fine details & small touch ii. Have high sensitivity b. Largest RFs → Found in large areas like thighs and calves i. Respond to broader areas ii. Less sensitive 2. Visual Receptive Field (Vision): Areas of the visual field a [neuron] responds to when stimulated by [light] a. Small Visual RFs → Detect very small details i. Ex. few minutes of an arc b. LARGE Visual RFs → Detect large portions of the visual scene i. Ex. whole page of a book Receptive Fields can be Described Along Different Dimensions Each sensory modality has different dimensions of defining receptive fields → AKA neurons respond to different forms of stimulus depending on the sensory modality 1. Visual RF → Neurons respond to vision along 2 dimensions of space around you a. Neurons correspond to specific location in visual field 2. Olfactory RF → Neurons respond to dimensions of carbon chain length of an odor 3. Somatosensory RF → Neurons receptive fields are mapped along body surface a. Each neuron responds to a specific area of your skin 4. Numerical RF → Neurons respond to numbers Topographic Maps Topographic Map: Orderly representations of sensory space 1. Each sensory modality has different sizes of neural RFs covering their sensory space (mix of small and large RFs) 2. Allow us to localize and identify objects + Identify objects regardless of position (position invariance) 3. Neurons that process similar areas are next to each other in the brain a. Neurons that process farther apart stimuli are distant in the brain (hands and feet ex.) Features 1. Brain space in maps is distributed based on level of sensitivity required to process stimuli properly a. Require detailed processing = Occupy larger part of topographic map 2. Sensory space is mapped multiple times in the brain a. Each distinct visual brain area contains a complete representation of half of the visual space i. Visual area in left hemisphere predominantly represents the right visual field (vv) Types of Topographic Maps 3 Types of Topographic Maps: 1. Retinotopic Map: Orderly representation of Visual Space/ hemifield → Reflects organization of the Retina (processed in the visual cortex) a. Each hemifield is defined as a separate brain area → combined cover= entire visual hemisphere b. Each distinct visual brain area contains a complete representation of half of the visual space i. Primary visual cortex → left hem processes right vf and vv 2. Tonotopic Map: Orderly representation of Sound Frequency, processed in the auditory cortex a. Sound frequency processed in primary and secondary auditory cortex areas b. 3. Somatotopic Maps: Orderly representation of body surface, processed in somatosensory cortex a. Overlays parts of body that neurons respond to b. Topographic Map Analysis Advantages → Efficient design of maps allows us to [group neurons] that are highly interconnected 1. Neurons that respond to nearby regions are grouped together and interact more → grouping neurons= less wiring Challenges → Not every neuron is connected to others in the map 1. Lots of Neurons in map = Each neuron connects to fewer neurons 2. Little Neurons in map = Each neuron connects to more neurons a. Occurs because there's ‘more space’ to make connections i. Makes wiring easier and more compact ii. Allows for distant neurons to be more readily connected → Better integration of distant information Lecture 6, Coordinate Systems and Brains Babel Fish Reference Frames Reference Frame:Coordinate system used to represent the position of an object 1. Position is only meaningful with reference to known points a. Origin of coordinate system = reference point 2. Reference fram can change depending on where we set the origin 2 Types of Reference Frames 1. Egocentric Reference Frame: Desrbines position of objects relative to one's own body or [viewpoint ] a. Origin = Specific part of your body (head, eyes, etc.) b. Changes as you move c. Egocentric RF processing occurs in Parietal Cortex 2. Allocentric Reference Frame: Desrbines position of objects relative to other objects or a [fixed environment] a. Origin = fixed location in environment or Origin= Specific object b. Does not change when you move c. Allocentric RF processing occurs in hippocampus (of medial temporal lobe) Reference Frames Broken Down Egocentric Reference Frames: 1. Eye-centered (retinotopic) → Eye as origin of coordinate system a. Movement of [eye gaze] causes image of object to change positions on the retina → retina transmits info about stimuli to the brain 2. Head-Centered → Head as origin of coordinate system 3. Body-Centered → Body (or body part) as origin Allocentric Reference Frames: 1. Object Centered → Particular object as origin 2. World Centered → Fixed location in environment as origin, described using global fixed cardinal systems a. Cardinal directions (N,E,S,W) and Geographic coordinates (latitude, longitude) Retrosplenial Cortex Involved in Spatial Transformations Retrosplenial Cortex: Contains both [allocentric] and [egocentric] cells → acts as a bridge between the two reference frames Located between the [hippocampus] and [parietal cortex] Connected with anterior thalamus- Has access to head-direction information Transformations Between Allocentric and Egocentric Information Transformations between [allocentric] and [egocentric] information requires head-direction information Head-Direction Information: Signals in the brain that track which direction your [head is facing] 1. Head direction cells found in anterior thalamus 2. Each [head direction cell] fires when your head points in a specific direction- regardless of body position or orientation of head relative to body a. Ex. cell may only fire when head is pointing northwest 3. Head Direction Info + Egocentric Info = Allocentric Spatial Representations a. Allows us to recognize location no matter what way your head is facing Interactions Between Parietal Cortex and Hippocampus Allocentric Information → Processed in Hippocampus Describes position of objects based on other object or fixed location as origin Does Not change when you move Egocentric Information → Processed in Parietal Cortex Describes position of objects based on your self/ body Changes as you move Pathway between [parietal cortex] and [hippocampus] used to switch between [egocentric] and [allocentric] spatial representations during navigation → Parietal cortex, hippocampus, and retrosplenial cortex help us understand where we are and how we move through space Parietal Cortex → Hippocampus [Egocentric Info → Allocentric] 1. Parietal cortex provides egocentric spatial information to the hippocampus 2. Hippocampus integrates information with its existing allocentric map 3. Allows us to track our current position in the environment Hippocampus → Parietal Cortex 1. Hippocampus sends information back to [parietal cortex] to help plan movements 2. Allows us to decide where to go next when navigating space Sensorimotor Transformations Along the Dorsal Pathway Dorsal Pathway → Top, gives information about where an object is, and how to use it Transforms sensory information into action ○ Dorsal path connects the visual cortex to the [parietal cortex] and [motor related areas] ○ Helps us guide movements based on sensory input Sensorimotor Transformations: How brain converts [what we see] into movements needed to reach an object Brain remaps visual information into [movement instructions] so that your arm and body can reach the correct spot 1. Receive information about location of target via [eye-centered, egocentric coordinates] 2. Brain remaps visual information into [movement instructions] for arm and hand to move towards object a. Remapping can occur in one or more transition steps Lecture 7, Synaptic Transmission and Plasticity Terms Synapse: Connection between two neurons; pathway where information is transmitted Neurotransmitter: Chemical messengers sent across synapse; how neurons communicate Receptor: Protein in cell membrane (and channel) that neurotransmitters bind to Post-Synaptic Plasticity: Small change in in neurons membrane voltage, caused by ions moving through channels (due to neurotransmitter signalling Happens after presynaptic neuron sends neurotransmitters to postsynaptic neuron Can be excitatory or inhibitory Types of Synapses 3 types of synapses: 1. Axo-Dendritic: Axon connects to dendrites a. Most common type b. Plays key role in processing and integrating incoming signals 2. Axo-Somatic Synapse: Axon connects to cell body a. Influences post-synaptic neurons action potential firing 3. Axo-Axonic synapse: Axon connects to axon, which connects to the cell body of another axon a. Regulates neurotransmitter release of postsynaptic neuron Synaptic Structure Synaptic Cleft: Space between [pre-synaptic] and [post-synaptic] neuron Synaptic Vesicle: In presynaptic neuron- stores and releases neurotransmitters When action potential reaches synapse, vesicle fuses with cell membrane and releases neurotransmitters into synaptic cleft Neurotransmitters Neurotransmitters: Chemical Messengers sent across synapse, from presynaptic to postsynaptic cell Stored in presynaptic neuron vesicles 3 Types of Neurotransmitters 1. Amino Acid Neurotransmitters- Adjust post-synaptic plasticity a. GABA: Main inhibitory neurotransmitter (in cc) b. Glutamate: Main excitatory neurotransmitter (in cc) 2. Amine Neurotransmitters- Neuromodulaters, adjust how neurons respond to signals a. Dopamine b. Acetylcholine c. Epinephrine d. Norepinephrine e. Histamine f. Serotonin i. (all end in -ine or -in) 3. Peptide Neurotransmitters **Each neuron contains only one type of amino acid or amine neurotransmitter Either contains GABA or glutamate + peptides Neurotransmitter Synthesis and Storage Synthesis and storage of neurotransmitters a neuron 1. Neurotransmitter Production -2 main areas of production a. Peptide NT → Made in Cell body (Rough ER, Golgi, pushed through axon terminal) b. Amino Acid NT → Made in Axon Terminal from peptides 2. Vesicle Storage → Neurotransmitters packaged into synaptic vesicles at axon terminal Neurotransmitter Release 1. Docking → Synaptic vesicles holding NT docked at active zone of [presynaptic membrane] a. Inside cell membrane: i. SNARE proteins mediate fusion of vesicle to membrane ii. Synaptotagmin activate fusion proteins- triggers fusion process 2. Depolarization Cascade → Action potential depolarization of axon leads to Ca2+ channels [opening] a. Ca2+ enters presynaptic membrane 3. Neurotransmitter Release → Ca2+ triggers neurotransmitter release 4. Vesicle Recycled Depolarization Cascade Extended Ca2+ release in presynaptic membrane triggers the fusion of vesicles to the membrane → results in neurotransmitters being released 1. SNARE proteins control the fusion of the viscle with the cell membrane at the docking zone a. Synaptotagmin activates fusion proteins and triggers fusion process 2. Calcium Ca2+ enters cell membrane due to depolarization cascade of action potential a. Synaptotagmin acts as calcium receptor → Triggers fusion of vesicle and NT release 2 Classes of Postsynaptic Neuron Receptors 2 types of receptors on postsynaptic neuron- that receive the neurotransmitters 1. Ligand Gated Channels (Ionotropic Receptor): Opens due to binding of NT, changes membrane potential (depolarizes or hyperpolarizes) a. Contains 2 functional domains: i. Extracellular domain- where NT binds ii. Membrane spanning domain- forms ion channel b. Produce rapid postsynaptic effects- faster than GPCR 2. G-Protein Coupled Receptors (Metabotropic Receptor): NT binding activates G-Protein inside cell a. G-Protein modulates other ion channels- helps open them Different Receptor Subtypes of Each Amino Acid Neurotransmitter Agonist: NT binds to receptor and amplifies effect Antagonist: Suppresses effect Amino acid + Receptor subtypes GABA (inhibitory) 1. GABA-A → Ionotropic= associated with ligand gated channel 2. GABA-B → Metabotropic= Associated with GPCR Glutamate (excitatory) 1. AMPA- ionotropic 2. NMDA- ionotropic 3. Kainte- ionotropic Glutamate Ionotropic Receptor Subtypes Glutamate: Excitatory amino acid NT 3 receptor subtypes that respond to glutamate and amplify its effect via their corresponding agonists Receptor Subtypes: 1. AMPA receptor- AMPA agonist 2. NMDA receptor - NMDA agonist 3. Kainate receptor - Kainate agonist Breakdown When glutamate binds to receptor subtype, the corresponding agonist amplifies glutamates effect, and opens the channel The NMDA receptor only opens if the cell is already depolarized- meaning its only activated under important circumstances Drugs can bind to receptors to enhance or block synaptic transmission ○ Benzodiazepine- binds to GABA and enhances its effects Post Synaptic Responses - EPSP or IPSP Post-Synaptic Potential: Small change in the [membrane potential voltage] in response to neurotransmitter binding to its receptors Two Types of Postsynaptic Potentials: 1. Excitatory Postsynaptic Potential (EPSP): Depolarizes (+) Cell membrane → Makes action potential more likely to fire a. Occurs when excitatory neurotransmitters [bind] to receptors (Glutamate) 2. Inhibitory Postsynaptic Potential (IPSP): Hyperpolarizes (-) Cell membrane → Makes action potential less likely to fire a. Occurs when inhibitory neurotransmitters [bind] to receptors (GABA) Synaptic Integration Synaptic Integration of multiple EPSPs must be summed to allow for action potential generation or cell depolarization 2 forms of signal summations: 1. Spatial Summation: Multiple synaptic inputs are [summed] to produce a larger postsynaptic effect 2. Temporal Summation: Subsequent inputs from [one neuron] are fired in quick succession & accumulated a. Summed effects can reach threshold to trigger action potential Synaptic Plasticity- 🔺Strength of Synapse Synaptic Plasticity: Change in synapse strength, in response to brain activity 1. Adjusts how much postsynaptic neuron responds to a signal 2. Does Not change form of PFP- if excitatory or inhibitory- only changes its strength 3. Plasticity can be increased or decreased a. Increased synaptic strength= enhanced synaptic transmission = increased corresponding PSP b. Decreased synaptic strength = weakened synaptic transmission = weakened corresponding PSP 2 Types of Synaptic Plasticity 1. LTP: Strengthening of synapses, induced by high frequency activity of [presynaptic neuron] (100Hz/1s) a. More effectively transmits excitatory signals (EPSP), induced by glutamate → depolarize cell membrane b. Enhances synaptic transmission 2. LTD: Weakening of synapses, induced by low frequency activity of [presynaptic neuron] (1Hz/ 10 min) a. Less effective at transmitting signals - for either EPSPs or IPSPs 2 Timescales of Plasticity 1. Short-term Synaptic Plasticity: Synaptic strength returns to baseline without continued pre-synaptic stimulation a. Short term potential and depression 2. Long-term Synaptic Plasticity: Imp mechanism underlying learning and memory a. Long term potential and depression 3 Mechanisms for LTP Increased synaptic strength underlies learning and memory processes LTP= Primarily associated with EPSPs and Glutamate ○ + Glutamate subtype receptors AMDA, NMDA, Kainate 3 Mechanisms employed by LTP to enhance synaptic transmission 1. Increase effectiveness of AMPA receptors by adding phosphate groups to them a. Increases AMPA receptors sensitivity to glutamate → Glutamate received by postsynaptic cell will have a stronger effect due to increased sensitivity 2. Insert more AMPA receptors on synapse a. More AMPA receptors = More binding sites for glutamate= stronger signal strength between pre and postsynaptic neurons = stronger connection 3. Increase neurotransmitter release → strengthens synaptic connection = enhances communication between pre and postsynaptic neurons LTP, LTD vs. Ca2+ Large Increase in Ca2+ in [post-synaptic] cell = LTP Induced= EPSPs transmitted = Cell membrane depolarized Due to r high-frequency presynaptic cell activity → effectively transmits EPSPs Add phosphate groups to AMPA to enhance LTP synaptic transmission ○ LTP → Strengthens Synapses, Increases EPSP transmission, in response to high frequency stimulation Small Increase in Ca2+ in postsynaptic cell = LTD induced Due to low frequency presynaptic cell stimulation ○ LTD → Weakens synapses, decreases EPSP or IPSP, in response to low frequency stimulation EPSP= Depolarizes cell membrane= action potential more likely to fire Glutamate = primary excitatory nt → when it binds to postsynaptic cell it excites it, creating an EPSP in postsynaptic cell LTP= enhances EPSP transmission IPSP= hyperpolarizes cell membrane= action potential less likely to fire GABA= primary inhibitory nt → when it binds to postsynaptic cell it inhibits it, most likely creating an IPSP on postsynaptic cell Lecture 8, Types of Memory Different Types of Memory 2 Forms of Memory: 1. Declarative Memory: Memory of facts and events, consciously recalled a. 2 forms of declarative memory: 1. Episodic Memory: Memory of personal events 2. Semantic Memory: General facts about the world 2. Non-declarative MEmory: Memory of procedural skills, habits, and conditioned responses, recalled unconsciously a. Classical conditioning skills- don't require conscious recall after being learned i. Procedural memory- riding a bike, playing instrument ii. Emotional responses Memory Loss Disorders: Amnesia: Disorder of memory Occurs due to injury, stroke, lesions → if you lesion or oinjuse one brain area, other areas will be connected 1. Retrograde Amnesia: Forget past memories; can make new memories 2. Anterograde Amnesia: Can't form new memories; can recall past memories Medial Temporal Lobe Medial Temporal Lobe: Plays crucial role in forming and storing declarative memories Patient H.M- Damaged Medial Temporal lobe 1. Patient H.M had severe epilepsy and seizures 2. Underwent procedure to remove medial temporal lobe → resulted in anterograde amnesia a. Patient couldn't form new declarative memories, but could still learn procedural skills b. Patient IQ, Personality, perception and nondeclarative memory stayed the same Hippocampus Hippocampus in Medial Temporal Lobe Hippocampus: Consolidates STM → LTM, spatial navigation, episodic memory 1. Hippocampus only required for memory consolidation for certain period of time 2. Does not store old memories- Acts as indexing system to memories 3. Interacts with neocortex to consolidate and retrieve memories Neocortex: Where declarative memories are stored (in temporal lobe) 1st layer of cerebral cortex Cortico-Hippocampal Circuits Consists of: Neocortex, Thalamus, Parahippocampal areas, Hippocampus Hippocampus Functions- 1. Episodic memory- store, consolidates, retrieves 2. Spatial navigation- mental maps of environment a. Creates cognitive maps of environment b. Contains place cells which activate with you're in a specific location Neocortex- 1. 1st layer of cerebral cortex- stores declarative memories 2. Direct path from neocortex and hippocampus exists a. Interactions may involve thalamus Episodic Memory Episodic Memory: Conscious memory of [personal events] Employ mental time travel when recalling → remember when and where event happened 2 Features of Episodic Memory 1. Pattern Separation:Ability to keep similar memories distinct 2. Pattern Completion: Ability to retrieve memory from partial input a. Ex. seeing or smelling smth that remind u of a specific memory Hippocampus x Episodic Memory 2 major pathways: 1. [cortical input] to hippocampus via entorhinal cortex 2. Hippocampal output to [cortex] via subiculum/ entorhinal cortex Breakdown 1. Hippocampus binds cortical inputs into integrated memory traces via conjunctive encoding- Hippocampus combines sensory information from dif brain areas to create cohesive memory) a. Helps distinguish similar memories by encoding them as [separate events] via pattern separation 2. Hippocampus reinstates previous activity patterns via retrieval - hippocampus reactivates neural activity patterns form original experience when recalling a memory via retrieval a. If only part of the memory is accessible, hippocampus fills in missing details via pattern completion Semantic Memory Semantic Memory: Memory of general world facts Don't need to recall context that we learned information- not tied to personal experience ○ Ex. language, concepts and ideas Semantic memory relies on many distributed networks of brain areas Response Characteristics of Temporal Lobe Neurons Medial Temporal Lobe:Involved with memory, includes hippocampus, and neocortex Has sparse coding - only little amounts of neurons code information & no obvious topography organization MEdial temporal lobe contains Category Selective Cells: Cells that respond selectively to particular categories of information. Types of category selective cells: 1. Face Selective Cells: Activated by faces 2. Concept Cells: Highly specific; respond to specific concepts across different sensory modalities a. Have to consciously know/ be aware of the concept, fo the cell to respond b. Have Multimodal Invariance: Cells have similar neural responses to object regardless of its physically viewed or identified from text or speech Synaptic Plasticity in Human Temporal Lobe LTP and LTD can be induced in human temporal cortex 1. Low frequency stimulation = LTD produced 2. High frequency stimulation = LTP produced a. LTP = strengthens EPSPs= Cell depolarized= action potential more likely to fire