Behavioral Neuroscience Learning and Memory PDF
Document Details
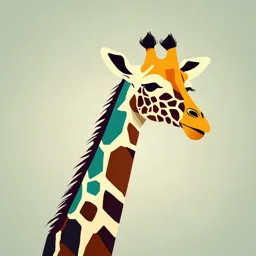
Uploaded by RoomierCloisonnism
Universität Tübingen
Tags
Summary
This document explores the topic of learning and memory, with a specific focus on the famous case study of patient H.M. The text examines different types of memory and how brain damage can affect recollection abilities. It describes the impact of the surgery on H.M.'s memory, noting his inability to create new long-term memories. The case is analyzed with regard to the relationship of learning and memory.
Full Transcript
Learning and Memory 17 Trapped in the Eternal Now Henry Molaison, known to the world as patient H.M. in a classic series of research articles, was probably the most famous research subject in the history of...
Learning and Memory 17 Trapped in the Eternal Now Henry Molaison, known to the world as patient H.M. in a classic series of research articles, was probably the most famous research subject in the history of brain science. Henry started to suffer seizures during adolescence, and by his late 20s, Henry’s epilepsy was out of control. Because tests showed that his seizures began in both temporal lobes, a neurosurgeon removed most of the anterior temporal lobes, on both sides, in 1953. Henry’s surgery relieved his epilepsy, but that relief came at a terrible, unforeseen price: Henry had lost the ability to form new memories (Scoville and Milner, 1957). For more than 50 years after the surgery, until his death in 2008, Henry could retain any new fact only briefly; as soon as he was distracted, the newly acquired information vanished. Long after the surgery, he didn’t know his age or the current date, that his parents had died years previously, or indeed any of the events that had transpired in the time since his surgery. His IQ remained a little above average (Corkin et al., 1997)—IQ tests don’t require remembering new facts for more than a few minutes—but he knew that some- thing was wrong with him. Every day is alone in itself, whatever enjoyment I’ve had, and whatever sorrow I’ve had.… Right now, I’m wondering, have I done or said anything amiss? You see, at this moment everything looks clear to me, but what happened just before? That’s what wor- ries me. It’s like waking from a dream. I just don’t remember. (B. Milner, 1970, p. 37) Henry’s inability to form new memories meant that he couldn’t construct a lasting re- lationship with anybody new. No matter what experiences he might share with someone he met, Henry would have to start the acquaintance anew the following day, because he would have no recollection of ever meeting the person before. What happened to Henry, and what does his experience teach us about learning and memory? All the distinctively human aspects of our behavior are learned: the languages we speak, how we dress, the foods we eat and how we eat them, our skills and the ways we reach our goals. So much of our own individuality depends on learning, the process of acquiring new information, and on memory, the ability to store and retrieve that information. We begin this chapter with a discussion of the major types of memory because research in the twentieth century, including the case of H.M., revealed that there are fundamentally different types of memory, relying on varying networks of brain regions. In the second part of the chapter, we delve into what we know about how learning alters the structure of brain tissue at a more fine-grained cellular level. Go to Brain Explorer bn8e.com/17.1 Functional Perspectives on Learning and Memory learning The process of acquiring new We can discover a great deal about learning and memory by examining how they and relatively enduring information, behav- fail. Clinical case studies show that memory can fail in very different ways, indicat- ior patterns, or abilities, characterized by ing that there are different forms of learning and memory, and that multiple brain modifications of behavior as a result of regions are involved. The clinical research has guided further studies, using animal practice, study, or experience. models and brain imaging; together, these diverse approaches are generating a com- memory 1. The ability to retain infor- prehensive picture of brain mechanisms of learning and memory. mation, based on the mental process of learning or encoding, retention across some interval of time, and retrieval or There Are Several Kinds of Learning and Memory reactivation of the memory. 2. The specific information that is stored in the brain. The terms learning and memory are so often paired that it sometimes seems as if one amnesia Severe impairment of necessarily implies the other. We cannot be sure that learning has occurred unless memory. a memory can be elicited later. Many kinds of brain damage, caused by disease or retrograde amnesia Difficulty in accidents, can produce highly specific types of memory impairment. We’ll start our retrieving memories formed before the survey by looking at a few classic case studies of memory impairments and the brain onset of amnesia. damage that caused them. patient H.M. A person who, because For patient H.M., the present vanished into oblivion of damage to medial temporal lobe struc- tures, was unable to encode new declara- Amnesia (Greek for “forgetfulness”) is a severe impairment of memory, usually as tive memories. Upon his death we learned a result of accident or disease. Loss of memories that formed prior to an event (such his name was Henry Molaison. as surgery or trauma)—called retrograde amnesia (from the Latin retro-, “back- anterograde amnesia The inability ward,” and gradi, “to go”)—is not uncommon. After an accident that damages the to form new memories beginning with the brain, people often have retrograde amnesia with regard to events that happened a onset of a disorder. few hours or days before the accident, or even a year. But despite dramatic depictions declarative memory A memory that you may see on TV, it is unlikely that longer-term (or “complete”) retrograde memory can be stated or described. loss has ever occurred. Patient H.M.—Henry Molaison, whom we met at the start of the chapter (FIGURE 17.1)—suffered from a far more unusual symptom: his apparent inability to retain new material for more than a brief period. The inability to form new memories after an event is called anterograde amnesia (the Latin antero- means “forward”). In Henry’s case, most old memories remained intact, but he had difficulty recollect- ing any events after his surgery. Over the very short term, Henry’s memory was normal. If given a series of six or seven digits, he could immediately repeat the list back without error. But if he was given a list of words to study and then tested on them after other tasks had inter- vened, he could not repeat the list or even recall that there was a list. So Henry’s case provided clear evidence that short-term memory differs from long-term memory—a distinction long recognized by biological psychologists on behavioral grounds (W. James, 1890) and which we will discuss in more depth later. Henry’s surgery removed the amygdala, most of the hippocampus, and some surrounding cortex from both temporal lobes (FIGURE 17.2). The memory deficit seemed to be caused by loss of the medial temporal lobe, including the hippocampus, because other surgical patients who had received the same type of damage to the amygdala, but less damage to the hippocampus, did not exhibit comparable memory impairment. But to the puzzlement of researchers, it seemed that bilateral hippo- campal lesions in laboratory animals did not produce comparable memory deficits (Isaacson, 1972; Mumby, 2001). What might account for this apparent discrepancy between humans and lab animals? An interesting finding about Henry’s memory deficit offered a clue. When Henry was given a mirror-tracing task (FIGURE 17.3A), he improved considerably over ten trials (B. Milner, 1965). The next day the test was presented again. When asked if he recognized the task, Henry said no, yet his performance was better than at the start of the first day (FIGURE 17.3B). Over 3 successive days, Henry never recognized the 17.1 PATIENT H.M. Henry Molaison task as something he had seen before, but his improved tracings showed evidence as a young man. (Courtesy of Dr. of memory, in the form of motor skill. Of course, we can’t ask lab animals if they Suzanne Corkin.) recognize a maze, so if an animal with comparable hippocampal damage shows 536 CHAPTER 17 (A) 17.2 BRAIN REGIONS CRUCIAL FOR MEMORY FORMATION (A) The hippocampus, whose name means “sea horse” in Latin, was named for its resemblance to that animal. (B) MRI scans of a healthy person (left) and Henry (right) show that Henry’s hippocampus (H) and entorhinal cortex (EC) were extensively damaged, bilaterally. Henry had a small amount Hippocampus of posterior hippocampus left, but the parahippocampal cortex (PH) was totally gone, and the cerebellum (Cer) was dramatically shrunken. (From Corkin et al., 1997.) Temporal lobe Mammillary Cerebellum body Normal H.M. (B) Ventral view H 1 cm EC Coronal view H PH Cer improvement on a task, we might prematurely conclude that the animal has normal memory. (A) The mirror-tracing task So, was Henry’s deficit simply an inability to learn verbal materi- al? Probably not. First, people with brain damage like Henry’s have difficulty reproducing or recognizing pictures and spatial designs that are not recalled in verbal terms. Second, although such indi- viduals have difficulty with the specific content of verbal material, they can learn some kinds of information about verbal material (N. ove Behavioral Neuroscience 8e.02 J. Cohen and Squire, 1980). For example, people with several kinds /16 of amnesia can learn the skill of reading mirror-reversed text but nfly Media Groupshow impaired learning of specific words (FIGURE 17.4). Subsequent research confirmed that the important distinction is not between motor and verbal performances, but between two general categories of memory: 1. Declarative memory is what we usually think of as mem- ory: facts and information acquired through learning. It is memory we are aware of accessing, which we can declare to others. This is the type of memory so profoundly impaired by Henry’s surgery. Tests of declarative memory take the form (B) Performance of H.M. on mirror-tracing task 30 Errors per trial Day 1 Day 2 Day 3 20 17.3 HENRY’S PERFORMANCE ON A MIRROR-TRACING TASK (A) Henry was given this mirror-tracing task to test motor skill. 10 (B) His performance on this task progressively improved over 3 0 successive days, demonstrating a type of long-term memory. 1 10 1 10 1 10 (After B. Milner, 1965.) Trial number LEARNING AND MEMORY 537 of requests for specific information that has been learned previously, such as a story or word list. It is the type of memory we use to answer “what” questions— and thus is difficult to test in animals. 2. Nondeclarative memory, or procedural memory—that is, memory about per- ceptual or motor procedures—is shown by performance rather than by conscious recollection. Examples of procedural memory include learning the mirror-tracing task at which Henry excelled, and the skill of mirror reading, along with more familiar skills such as riding a bicycle or juggling: things that you learn by doing. It is the type of memory we use for “how” problems, and is often (but not al- ways) nonverbal. FIGURE 17.5 illustrates this basic division of types of memory. Medial temporal lobe structures are crucial for declarative memory Publication of Henry Molaison’s case prompted an intensive effort to develop meth- ods for systematically studying declarative memory in monkeys and other lab ani- mals. To get around the inability of lab animals to verbally report their memories, 17.4 READING MIRROR-REVERSED the delayed non-matching-to-sample task (FIGURE 17.6)—a test of object rec- TEXT People with amnesia like ognition memory—was developed. In this task, monkeys must identify which of two Henry's can learn to read mirror- reversed text well, even though objects was not seen previously, with delays ranging from 8 seconds to 2 minutes they don’t remember practicing this (Spiegler and Mishkin, 1981). The important feature of this procedure is that the ani- skill. This demonstrates that their mal does not reach for the item that previously had a treat under it, because in that problem is not verbal, but rather an case the monkey might unconsciously associate reward with that object even if the inability to form declarative memo- animal had no conscious recollection of it. Instead, the monkey declares that it re- ries. members the object by reaching for the other object, the one that was not associated with a reward previously. Monkeys with extensive damage to the medial temporal lobe, and thus similar to Henry, are severely impaired on this task, especially with the longer delays. But which specific temporal lobe structures are most important? Selective removal of specific parts of the medial temporal lobes of monkeys re- vealed that the amygdala—one of the structures removed in Henry’s surgery—was not crucial for performance on tests of declarative memory. However, removal of the adjacent hippocampus significantly impaired performance on these tests and, as shown in FIGURE 17.7, the deficit was even more pronounced when the hippocam- pal damage was paired with lesions of the nearby entorhinal and parahippocampal cortex, and much worse when lesions of perirhinal cortex were added (Zola-Morgan et al., 1994). Humans similarly show larger impairments when both the hippocam- pus and medial temporal cortex are damaged (Rempel-Clower et al., 1996; Zola- Morgan and Squire, 1986), confirming that Henry’s symptoms resulted from the loss of the medial temporal lobe on both sides of the brain. nondeclarative memory Also One modern formulation of declarative memory for facts and events takes the called procedural memory. A memory that is shown by performance rather than by view that two interwoven processes are involved: recollection of the item in the spe- conscious recollection. cific context in which it was presented, and additionally the sense of familiarity with delayed non-matching-to-sample the features of the recalled item (Eichenbaum et al., 2007). Experimental evidence task A test in which, on each trial, the suggests that the two processes are served by differing components of the medial participant must select the stimulus that temporal lobe system. In this model, perirhinal cortex (see Figure 17.7A) is thought they have not seen previously. to be responsible for the sense of familiarity in memory, whereas recollection of Long-term memory Declarative: Nondeclarative (procedural): Things you know that Things you know that you you can tell others can show by doing Declarative memory can be tested Nondeclarative memory can be tested readily readily in humans because they can in other animals, as well as in humans. 17.5 TWO MAIN KINDS OF MEMORY: talk. Henry Molaison was unable to Henry was capable of this form of memory, DECLARATIVE AND form new declarative memories. exemplified by the skill of mirror tracing. NONDECLARATIVE 538 CHAPTER 17 Sample Test Food found under the nonmatching object Variable delay The monkey is originally presented with After a variable delay (seconds to Over a series of trials with different pairs of a sample object. When he displaces it, he minutes) the monkey is presented with objects, the monkey learns that food is present finds a pellet of food beneath. the original object and another object. under the object that differs from the sample. 17.6 THE DELAYED NON-MATCHING-TO-SAMPLE TASK the item is the function of the hippocampus (Lech and Suchan, 2013; Suzuki and Naya, 2014). Processing of contextual aspects of memory (including spatial cogni- tion, which we will discuss in more detail a little later) appears to depend especially on the parahippocampal cortex (Aminoff et al., 2013; Diana et al., 2013). In general, the performance of experimental animals suggests that the hippocampus acts as the final stage of convergence for adjacent regions of cortex (Squire et al., 2007; Zola et al., 2000), resulting in storage of declarative memories in the cortex. In keeping with this schema, people with specific hippocampal damage appear to have difficulties with recollection, while familiarity-based aspects of memory are spared (Addante et (A) Ventral view of monkey brain showing (B) Scores of groups with different lesions areas of different medial temporal lesions Parahippocampal Hippocampus 1.0 Normal monkeys (N) Perirhinal cortex cortex 0.5 Lesion of hippocampus (H) Entorhinal cortex H lesion extended to include the adjacent entorhinal cortex and 0.0 parahippocampal cortices (H+) Memory score H+ lesion extended –0.5 forward to include the anterior entorhinal and perirhinal cortices (H++) –1.0 –1.5 Control N H H+ H++ Lesion type 17.7 MEMORY PERFORMANCE AFTER MEDIAL TEMPORAL LOBE LESIONS (A) In this ventral view of a monkey brain, the positions of the hippocampus, ento- rhinal cortex, perirhinal cortex, and parahippocampal cortex are shown in different colors. (B) Different bilateral lesions of the medial temporal lobe yielded different results in tests of memory. (After Squire and Zola-Morgan, 1991.) LEARNING AND MEMORY 539 17.8 IMPAIRED FAMILIARITY AFTER SURGERY Patient N.B. received surgical N.B.’s surgery lesions in the left medial temporal lobe that selectively removed much of the removed much Supe of the left amygdala (red in this reconstruction of the medial temporal lobes) and perirhinal cortex (green) while completely sparing the hippocampus (blue). Following sur- rior amygdala and Anterior perirhinal cortex. gery, N.B. experienced reduced feelings of familiarity in memory tests although recollection of details was unimpaired, supporting a two-process model of medial temporal lobe function. (From Bowles et al., 2007.) al., 2012; Bowles et al., 2010). Conversely, people with surgical lesions that include Left the perirhinal cortex but not the hippocampus (FIGURE 17.8) experience impaired familiarity with stimuli despite preserved recollection (Bowles et al., 2007). Right Damage to the medial diencephalon can also cause amnesia In 1960, a young man now known to neuroscientists as patient N.A. suffered a bi- zarre accident in which a miniature sword injured his brain after entering through his nostril. He eventually recovered in most respects except that, like Henry Mo- laison, he was left with a profound anterograde amnesia, primarily for verbal ma- terial (Squire and Moore, 1979). He can give little information about events since his accident, although his memory for earlier events is near normal (Kaushall et al., 1981). Studying N.A. with MRI (FIGURE 17.9) showed damage to several components of the diencephalon (thalamus and hypothalamus) that have connections to the hip- pocampus, especially the dorsomedial thalamus, and to both mammillary bod- ies (see Figure 17.2A), as well as probable damage to the mammillothalamic tract (Squire et al., 1989). Like Henry Molaison, N.A. shows normal short-term memory but is impaired in forming declarative (but not nondeclarative/procedural) long-term patient N.A. A person who is unable to encode new declarative memories, memories. The similarity in symptoms suggests that the medial temporal region because of damage to the dorsal thala- damaged in Henry’s brain and the midline diencephalic region damaged in N.A. mus and the mammillary bodies. mammillary body One of a pair of nuclei at the base of the brain, that connect to the hippocampus and play a Anterior Posterior role in memory. A B C 17.9 THE BRAIN DAMAGE IN PATIENT N.A. Successive MRI scans show a promi- nent diencephalic lesion on the left side of the brain (yellow arrows), as well as a lesion on the floor of the third ventricle (red arrows). The mammillary bodies should be present in B and C, but they are totally absent. (From Squire et al., 1989; MRI scans courtesy of Dr. Larry Squire.) 540 CHAPTER 17 17.10 BRAIN DAMAGE IN PATIENTS are normally parts of a larger memory system. Studies of people WITH KORSAKOFF’S with surgical lesions of the fornix, which interconnects the hip- SYNDROME (Image courtesy of Dr. D. P. Agamanolis.) pocampus and mammillary bodies, confirm and extend this idea, as such individuals experience selective difficulty forming new declarative memories (Tsivilis et al., 2008). That idea is reinforced by studies of people with Korsakoff’s syndrome —named for its nineteenth-century discoverer, Rus- sian neurologist Sergei Korsakoff—who also have anterograde amnesia for declarative memories. People with Korsakoff’s syn- drome frequently deny that anything is wrong with them, and they often confabulate —that is, fill a gap in memory with a falsi- fication that they seem to accept as true. Temporal lobe structures, including the hippocampus, are typically normal in people with Korsakoff’s syndrome (Mair et al., 1979). But their brains show shrunken, diseased mammillary bodies, as well as some dam- These oval-shaped mammillary age in the dorsomedial thalamus (FIGURE 17.10). This damage is bodies are darkened as a result similar to that seen in N.A. The mammillary bodies may serve as of bleeding and cell death. a processing system connecting the medial temporal lobes (which were removed from Henry Molaison) to the thalamus via the mammillothalamic Korsakoff’s syndrome A memory tract and, from there, to other cortical sites (Vann and Nelson, 2015). Damage to the disorder, related to a thiamine deficiency, basal frontal cortex, also found in people suffering from Korsakoff’s syndrome, prob- that is generally associated with chronic ably causes the denial and confabulation that differentiates them from other people alcoholism. who have amnesia, such as Henry. confabulate To fill in a gap in memory The main cause of Korsakoff’s syndrome is lack of the vitamin thiamine (or B1). with a falsification. It often occurs in Alcoholics who obtain most of their calories from alcohol and neglect their diet often Korsakoff’s syndrome. exhibit this deficiency. Treating them with thiamine can prevent further deteriora- patient K.C. A person who sustained tion of memory functions but will not reverse the damage already done. If alcoholic damage to the cortex that rendered him unable to form and retrieve new episodic beverages were supplemented with thiamine, then many new cases of Korsakoff’s memories, especially autobiographical syndrome could be prevented (Price et al., 1987). memories. Upon his death we learned that These studies make it clear that a brain circuit that includes the hippocampus, his name was Kent Cochran. the mammillary bodies, and the dorsomedial thalamus is needed to form new de- episodic memory Memory of a clarative memories. But these case studies also clearly show that established declara- particular incident or a particular time and tive memories, formed before brain damage, are not stored in these structures. If the place. memories had been stored there, they would have been lost when the structures were damaged. So where are memories stored? Mathematical models based on the known connectivity patterns of cortical neurons indicate that the cortex is optimized for information storage (Brunel, 2016), and as we’ll see next, clinical observa- tions confirm that the cortex serves as a repository for memories. The cortex is essential for long-term storage of memories One striking case study indicates that the cortex is crucial for storing memo- ries once they are formed, and also illustrates an important distinction between two subtypes of declarative memory. Profiled in numerous scientific reports as patient K.C., Kent Cochran sustained serious brain damage in a motorcycle accident at age 30. For more than 30 years, from his accident until the day he died in 2014 at age 62, Kent was unable to retrieve any personal memory of his past, although his general knowledge remained good. He conversed easily and played a good game of chess (FIGURE 17.11), but he could not remember where he learned to play chess or who taught him the game. Detailed autobiographi- cal declarative memory of this sort is known as episodic memory: you show episodic memory when you recall a specific episode in your life or relate an 17.11 PATIENT K.C. Kent Cochran lost the ability to form or retrieve episodic memories because of brain damage sus- tained in a motorcycle accident. (From Tulving, 2002.) LEARNING AND MEMORY 541 semantic memory Generalized event to a particular time and place. In contrast, semantic memory is general- memory—for instance, knowing the ized declarative memory, such as knowing the meaning of a word without knowing meaning of a word without knowing where where or when you learned that word (Tulving, 1972). If care was taken to space or when you learned that word. out the trials to prevent interference among items, K.C. could acquire new semantic skill learning Learning to perform a knowledge (Tulving et al., 1991), and like the lead character in the film Memento, he task that requires motor coordination. understood that time passes, and that he had a past, and that he also had a future to priming Also called repetition priming. plan for (Craver et al., 2014). But even with careful training, K.C. could not acquire The phenomenon by which exposure to a new episodic knowledge. stimulus facilitates subsequent responses Brain scans of K.C. revealed extensive damage to the cerebral cortex and severe to the same or a similar stimulus. shrinkage of the hippocampus and nearby parahippocampal cortex (Rosenbaum et associative learning A type of al., 2005). As with Henry, the bilateral hippocampal damage probably accounts for learning in which an association is formed K.C.’s anterograde declarative amnesia. But that damage cannot account for the se- between two stimuli or between a stimu- lus and a response; includes both classi- lective loss of nearly his entire autobiographical memory (i.e., retrograde episodic cal and operant conditioning. amnesia), because other people with damage restricted to the medial temporal lobes lack this symptom. K.C.’s inability to recall any autobiographical details of his life, operant conditioning Also called instrumental conditioning. A form of even memories from years before his accident, may instead be a consequence of the associative learning in which the likelihood extensive damage to his frontal and parietal cortex (Tulving, 1989). that an act (instrumental response) will be There are a few rare individuals who have incredibly extensive episodic memories, performed depends on the consequences who can tell you exactly what they had for breakfast on June 20, 2002, or any other (reinforcing stimuli) that follow it. date (Price, 2008). A group of 11 such people with extreme autobiographical memory classical conditioning Also called were found to have larger temporal lobes, including a larger parahippocampal gyrus, Pavlovian conditioning. A type of associa- than controls (LePort et al., 2012). We don’t know whether these people were born tive learning in which an originally neutral with larger temporal lobes, and so had great autobiographical memory, or developed (conditioned) stimulus acquires the power great autobiographical memory, which caused their temporal lobes to enlarge. to elicit the response normally elicited by another (unconditioned) stimulus after the two stimuli are paired. A response elicited Different Forms of Nondeclarative Memory Involve by the unconditioned stimulus (US) is Different Brain Regions called an unconditioned response (UR); a response elicited by the conditioned We have seen that there are two different kinds of declarative memory: semantic and stimulus (CS) alone is called a conditioned episodic. Likewise, there are several forms of nondeclarative memory, and as we’ll response (CR). see, these depend on different neural systems. Specific functions depend on different forms of nondeclarative memory Unlike declarative memory, which we use to store the factual knowledge of our- selves and the world that we use in conversation and in our innermost thoughts, nondeclarative memories shape our abilities and responses to the world around us in ways that are difficult to express, but no less crucial for survival. Indeed, virtually every species of the animal kingdom seems capable of some sort of nondeclarative memory, even if very simple, indicating that it’s an ancient evolutionary adaptation. SKILL MEMORY In skill learning, participants learn how to perform a challenging task by practicing it over and over again. Experimental tests like the mirror-tracing task performed by Henry Molaison (see Figure 17.3) or learning to read mirror-re- versed text (see Figure 17.4) are examples of skill learning. So too are the everyday skills we acquire, like learning to ride a bike or to juggle (well, okay, maybe juggling isn’t an “everyday” skill, but you get the idea). Imaging studies have investigated learning and memory for different skill catego- ries, especially sensorimotor skills (e.g., mirror tracing), perceptual skills (e.g., learning to read mirror-reversed text), and cognitive skills (e.g., puzzles like the Towers of Ha- noi—which you can play on the website—that involve planning and problem solv- ing). All three kinds of skill learning are impaired in people with damage to the basal ganglia; damage to other brain regions, especially the motor cortex and cerebellum, also affects aspects of some skills. Neuroimaging studies in healthy people confirm that the basal ganglia, cerebel- lum, and motor cortex are important for sensorimotor skill learning (Grafton et al., 1992). For example, learning specific sequences of finger movements is associated with selective activation of motor cortex and the basal ganglia (Doyon et al., 1996; Hazeltine et al., 1997), with the cerebellum possibly providing error correction dur- 542 CHAPTER 17 ing learning (Flament et al., 1996). Often the activations shift among brain regions as performance changes during the course of learning, so learning appears to involve a complex set of interact- ing neural networks. PRIMING A change in the processing of a stimulus, usually a word or a picture, as a result of prior exposure to the same stimulus or related stimuli is referred to as priming (or repetition priming). For example, if a person is shown the word stamp in a list and later is asked to complete the word stem STA-, he or she is more likely to reply “stamp” than is a person who was not exposed to that word. Priming does not require declarative memory of the stimu- lus—Henry Molaison and other people with amnesia have shown priming for words they don’t remember seeing previously. In con- trast to skill learning, researchers have found that priming is not impaired by damage to the basal ganglia. 17.12 OPERANT CONDITIONING Animals in a Skinner box can learn to press a lever to gain food pellets In functional-imaging studies, perceptual priming (priming or to avoid footshock, a form of associative learning based on the visual form of words) has been related to reduced ac- called operant (or instrumental) conditioning. tivity in bilateral occipitotemporal cortex (Schacter et al., 1996), presumably because the priming makes the task easier. Concep- tual priming (priming based on word meaning) was associated with reduced activation of the left frontal cortex (Blaxton et al., 1996; Gabrieli et al., 1996; A. D. Wagner et al., 1997). And although it is primarily associated with de- clarative memory, evidence is mounting that the components of the medial temporal lobe memory system—notably the perirhinal cortex (Wang et al., 2010, 2014) and hippocampus (Addante, 2015)—are also involved in some forms of nondeclarative memory, including priming. ASSOCIATIVE LEARNING Learning that involves relations between events—for ex- ample, between two or more stimuli, between a stimulus and a response, or between an action and its consequence—is called associative learning. In one form of asso- ciative learning, called operant conditioning (also called instrumental conditioning), an association is formed between the individual’s behavior and the consequence(s) of that behavior. For example, lab animals can easily learn to press a lever to gain the reward of a tasty food pellet, using an apparatus like the one in FIGURE 17.12 , often called a Skinner box after its famous originator, B. F. Skinner. Many people are also familiar with another form of associative learning, termed classical conditioning (or Pavlovian conditioning), in which an initially neutral stimulus comes to predict an event. At the end of the nineteenth century, Ivan Pavlov 17.13 CLASSICAL CONDITIONING (1849–1936) found that a dog would salivate when presented with an auditory or In this famous experiment, by pair- visual stimulus if the stimulus came to predict the presentation of food. So, as il- ing food with the sound of a bell, lustrated in FIGURE 17.13, repeatedly ringing a bell before putting meat powder in Ivan Pavlov conditioned animals to a dog’s mouth will eventually cause the dog to start salivating when it hears the bell eventually salivate to the sound of the bell alone. During conditioning, Eventually the dog begins Before conditioning, the the bell is rung salivating in response to the dog reflexively salivates Before conditioning, repeatedly, and shortly bell, before the food is in response to food—an ringing of the bell Bell after each ringing, food presented, and will salivate unlearned response. produces no salivation. is presented to the dog. in response to the bell alone. Bell Response Response Response Response + Food Salivation Bell No salivation Food Salivation Salivation Unconditioned Unconditioned Conditioned Conditioned stimulus (US) response (UR) stimulus (CS) response (CR) LEARNING AND MEMORY 543 17.14 SUBTYPES OF DECLARATIVE AND Long-term memory NONDECLARATIVE MEMORY Declarative: Nondeclarative (procedural): Things you know that Things you know that you you can tell others can show by doing Episodic: Semantic: Skill learning: Priming: Conditioning: Remembering Knowing the Knowing how Being more likely Salivating when your first day capital of France to ride a bicycle to use a word you see a in school you heard recently favorite food alone. In this case the meat powder in the mouth is the unconditioned stimulus (US), which already evokes an unconditioned response (UR; salivation in the example). The sound of the bell is the conditioned stimulus (CS), and the learned response to the CS alone (salivation in response to the bell) is called the conditioned response (CR). Experimental evidence in lab animals shows that cerebellar circuits are crucial for simple eye-blink conditioning, in which a tone or other stimulus is associated with eye blinking in response to a puff of air. A PET study of human eye-blink con- ditioning (Logan and Grafton, 1995) found that in the course of conditioning, there was a progressive increase in activity in several regions of the brain, including not only the cerebellum, but also the hippocampus, the ventral striatum, and regions of the cerebral cortex. But activity in these other areas may not be essential for eye- blink conditioning in the way that the cerebellum is. For example, people with hip- pocampal lesions can acquire the conditioned eye-blink response, but people with unilateral cerebellar damage can acquire a conditioned eye-blink response only on the side where the cerebellum is intact (Papka et al., 1994). We’ll look more closely at neural mechanisms of eye-blink conditioning later in the chapter, as well as the cel- lular and molecular underpinnings of a very simple type of memory—nonassociative learning—that is formed in basic neural circuits of even the simplest animals. Breedlove Behavioral Neuroscience The8e taxonomy of memory we presented in Figure 17.5 is updated in FIGURE Fig. 17.14 07/20/16 17.14, where we have added the subtypes of declarative and nondeclarative memory Dragonfly Media Group described in this section, along with some examples. Medial temporal mechanisms keep track of positions in spatial, temporal, and social networks The caricature of the white-coated scientist watching rats run in mazes, a staple of cartoonists to this day, has its origins in the intensive memory research of the early twentieth century. The early work indicated that rats and other animals don’t just learn a series of turns but instead form a cognitive map (an understanding of the relative spatial organization of objects and information) in order to solve a maze (Tol- man, 1949). Animals apparently learn at least some of these details of their spatial environment simply by moving through it, a phenomenon that was termed latent learning (Tolman and Honzik, 1930). We now know that, in parallel with its role in other types of declarative memo- ry, the hippocampus is a crucial neural participant in spatial learning. Within the rat hippocampus are found many neurons that selectively encode spatial location (Leutgeb et al., 2005; O’Keefe and Dostrovsky, 1971). These place cells become active when the animal is in—or moving toward—a particular location (Pastalkova et al., 2008) (FIGURE 17.15). If the animal is placed in a new environment, its place cognitive map A mental representa- cell activity indicates that the hippocampus remaps to the new locations (Moita et tion of a spatial relationship. al., 2004). Lesions of this part of the hippocampus severely impair spatial learning place cell A neuron within the hippo- in rats (McNaughton et al., 1996) and humans (Bartsch et al., 2010). The Nobel Prize campus that selectively fires when the in Physiology or Medicine for 2014 was awarded to John O’Keefe and the wife-and- animal is in a particular location. husband team of May-Britt and Edvard Moser for their work on understanding hip- 544 CHAPTER 17 Closed maze door pocampal place cells. Two types of cells discovered in nearby Water bottle entorhinal cortex help the animal to learn the local spatial en- vironment. Grid cells fire selectively when the animal crosses the intersection points of an abstract grid map of the local en- vironment, acting like an innate system of latitude and longi- The neuron denoted by yellow dots fired when tude (Hafting et al., 2005). Cells that fire in the same pattern the animal was in this have also been discovered in humans (J. Jacobs et al., 2013). part of the maze. Arrival at the perimeter of the local spatial map is signaled by the activation of entorhinal border cells (Solstad et al., 2008). Evidence is mounting that beyond its roles in forming de- clarative memories and keeping track of important locations in space, the hippocampus helps us to organize networks of data across multiple domains. For example, hippocampal function has been linked to mapping our social space—the web of social The neuron denoted by green dots fired when the rat was in connections to other people that we navigate daily in our work in this part of the maze. and personal lives (Alexander et al., 2016; Tavares et al., 2015). Likewise, researchers have recently discovered hippocampal 17.15 HIPPOCAMPAL PLACE CELLS In this simple maze, cells that appear to map locations in time, another important viewed from above, each colored dot represents the loca- aspect of developing coherent memories (Eichenbaum, 2014; tion of the rat when one specific place cell fired. Thus, all Schiller et al., 2015). In other words, the hippocampus helps of the yellow dots represent one neuron, all of the green organize the what, where, when, and with whom of memory. dots another neuron, and so on. As the figure plainly shows, place cells accurately encode a specific location in space. (Courtesy of Dr. Eva Pastalkova.) SPATIAL MEMORY AND THE EVOLUTION OF HIPPOCAMPAL SIZE Careful comparisons of natural behavior and brain anatomy have revealed that for many species, their manner of making a living has left an imprint on the hippocampus. For example, not only does the monkey hippocampus contain place cells (Rolls and O’Mara, 1995), it also fea- tures spatial view cells that respond to the part of the environment that the monkey is looking at, perhaps reflecting the importance of vision for primates. As another example, species of birds that hide caches of food in spatially scattered locations have larger hippocampi than noncaching species, even when the compari- son species are very close relatives that have otherwise similar lifestyles (Krebs et al., 1989; Sherry, 1992; Sherry et al., 1989) (see Figure 6.6). Lesions of the hippo- campus impair the ability of these birds to store and retrieve caches of food (Sherry and Vaccarino, 1989). Homing pigeons also have enlarged hippocampi relative to other varieties of pigeons, presumably Breedloveserving the Behavioral spatial demands Neuroscience 8e of their prodi- Fig. 17.15 et al., 1988). A relationship between spatial gious navigational abilities (Rehkamper 07/20/16 cognition and hippocampal sizeDragonfly is evident in Group Media mammals too. Just as with the food- caching birds, Merriam’s kangaroo rat, which stashes food in scattered locations, has a significantly larger hippocampus than its noncaching cousin, the bannertail kangaroo rat (L. F. Jacobs and Spencer, 1994). (For additional fascinating examples of the relation between hippocampal volume and spatial behavior, see A Step Further: Spatial Behavior and Hippocampal Structure in Humans and Other Species on the website.) grid cell A neuron that selectively fires when an animal crosses the intersection points of an abstract grid map of the local Successive Processes Capture, Store, and Retrieve environment. Information in the Brain border cell A neuron that selectively fires when an animal arrives at the perim- The span of time that a piece of information will be retained in the brain varies. eter of a local spatial cognitive map. Evidence suggests that there are as many as four different duration categories for sensory buffer An element of the memory. The briefest memories are held in sensory buffers (for visual stimuli, type of memory that stores the sensory they are sometimes called iconic memories); an example is the fleeting impression of a impression of a scene. glimpsed scene that vanishes from memory seconds later. These brief memories are short-term memory (STM) thought to be residual sensory neural activity (FIGURE 17.16). A form of memory that usually lasts only Somewhat more durable than the sensory buffers are short-term memories for seconds, or as long as rehearsal (STMs). If you look up a phone number and keep it in mind (perhaps through re- continues, especially while being used hearsal) just long enough to make the phone call, you are using STM. In the absence during performance of a task. LEARNING AND MEMORY 545 High of rehearsal, STMs last only about 30 seconds (J. Brown, 1958; L. Sensory buffer R. Peterson and Peterson, 1959). With rehearsal, you may be able Strength of memory to retain an STM until you turn to a new task a few minutes later, Working memory but when the STM is gone, it’s gone for good. Many researchers Intermediate-term now refer to this form of memory as working memory, in rec- memory ognition of the way we use it; this is how we hold information in mind while we are working with it to solve a problem or are oth- Long-term erwise actively manipulating the information. One influential memory Low model (Baddeley, 2003) subdivides working memory into three Time complementary components: Input 1. A phonological loop that contains auditory information 17.16 TEMPORAL STAGES OF MEMORY FORMATION (such as speech); this is what you use to rehearse that phone number. 2. A so-called visuospatial sketch pad that holds visual impres- sions of stimuli; you use this to imagine the route back to your car in a parking building. working memory A type of short- 3. An episodic buffer that contains more-integrated information, spanning across term memory that holds information avail- sensory modalities, sort of like movie clips. able for ready access during performance According to this model, the flow of information into and out of working memory is of a task. supervised by a fourth module, an executive control, which we’ll discuss in Chapter 18. intermediate-term memory (ITM) Some memories last only a little longer than short-term memories. Chances are A form of memory that lasts longer than short-term memory, but not as long as good that you can remember what you had for lunch today or yesterday, but not most long-term memory. of your lunches last week. You may recall today’s weather forecast, but not that of a few days ago. These are examples of what some memory researchers identify as intermediate-term memory—that is, a memory that outlasts what we typically consider to be STM but is far from being permanent (M. R. Rosenzweig et al., 1993). A variety of brain regions are involved in different attributes of working memory Because they span verbal and nonverbal material, in multiple sensory modalities, for multiple purposes, working memories tend to have unique features or attributes. So, nce 8e for example, an individual memory may include a mix of information about space, time, sensory perception, response, and/or emotional factors. Researchers have attempted to devise memory tasks that selectively tap some of these attributes of memory, in order to assess the relative contributions of different regions of the brain. FIGURE 17.17 presents some examples of some well-known tests that researchers use to probe working memory (Kesner, 1998; Kesner et al., 1993). For testing spatial location memory, the eight-arm radial maze is commonly used (FIGURE 17.17A). To solve this task correctly and receive a food reward, rats must recognize and enter an arm of the maze that they went down shortly beforehand. Rats were tested following surgical lesions of the hippocampus, the caudate nucleus, or the extrastriate cortex (visual cortex outside the primary visual area). Only the animals with hippocampal lesions were impaired on this predominantly spatial task—a result that is consistent with the role of the hippocampus in spatial cognition that we discussed earlier. The test shown in FIGURE 17.17B was used to assess the memory of the same rats for their own motor behavior. Here the animal must use working memory to remem- ber whether it made a left or right turn a few moments previously, and it receives a food reward only if it makes a turn in the same direction on a follow-up trial. Only the animals with lesions of the caudate nucleus were significantly impaired on this task. Finally, in the test depicted in FIGURE 17.17C, the rats were required to hold in working memory the sensory attributes of presented stimuli, identifying the novel stimulus in each pair of stimuli presented. Here, only the rats with extrastri- ate lesions were significantly impaired. This impressive lack of overlap between the symptoms of the different lesions nicely illustrates how memories involving different attributes are parceled out to diverse brain regions for storage. 546 CHAPTER 17 (A) Spatial-location recognition memory 90 Mean percentage of correct responses In the study phase of Brain region lesioned 85 each trial, the rat can Hippocampus choose any of the 80 eight arms. In the test Control 75 phase, doors block all Caudate nucleus but two arms: the arm 70 entered in the study Extrastriate 65 phase and one other. visual cortex The rat obtains food 60 only if it chooses the 55 Only rats with hippo- arm it entered in the 50 campal lesions are study phase. impaired, relative to 0 controls. 0 1–4 15 30 Delay (s) 0 184 cm (B) Response recognition memory 90 Mean percentage of correct responses In the first part of each trial, the rat Brain region lesioned 85 is placed in the middle compart- Hippocampus 1 2 3 80 ment on one side (2), and it finds food if it enters the compartment Control 75 to either its right (1) or its left (3). Caudate nucleus In the second part of the trial, it is 70 placed in the middle compartment Extrastriate 65 on the other side (5), and it finds visual cortex food only if it turns to the same 60 side of its body as in the first part. 55 Only rats with 50 caudate nucleus lesions are impaired, 0 relative to controls. 0 1–4 15 30 4 5 6 Delay (s) 0 36 cm (C) Object recognition memory (non-matching-to-sample) 90 Mean percentage of correct responses In the study phase of each trial, Brain region lesioned 85 the rat obtains food by displacing a sample object over a small food Hippocampus 80 well (top). In the test phase Control 75 (bottom), the rat chooses between Caudate nucleus two objects and obtains food only 70 if it chooses the object that does 65 Extrastriate not match the sample. visual cortex 60 55 Only rats with lesions 50 of the extrastriate visual cortex are 0 impaired, relative to 0 1–4 15 30 controls. 0 35.5 cm Delay (s) 17.17 TESTS OF SPECIFIC ATTRIBUTES OF MEMORY Brain lesion experi- ments testing spatial-location recognition (A), response recognition (B), and object recognition (C)—using the setups shown on the left—yielded the results shown on the right. (After Kesner et al., 1993.) LEARNING AND MEMORY 547 Memory systems Long-term memory Short-term memory (working memory) Declarative (explicit) Nondeclarative (implicit) Skill learning Classical Nonassociative Spatial Episodic Semantic (procedural) Priming conditioning learning memory Hippocampus, Lateral and Striatum, Neocortex Amygdala Reflex Hippocampus Sensorimotor medial anterior temporal motor cortex, and pathways and cortex and prefrontal temporal lobe, cortex, prefrontal cerebellum cerebellum cortex neocortex cortex Brain regions involved 17.18 BRAIN REGIONS INVOLVED IN DIFFERENT KINDS OF LEARNING AND MEMORY (After Bartsch and Butler, 2013.) It’s perhaps not surprising that the brain regions that do the initial processing of the stimuli often also act as the memory buffers for holding the stimuli in work- ing memory—visual information in visual cortex, motor information in motor areas, and so on. One additional common attribute of working memory is the passage of time—a delay between stimulus and response during which information must be held ready for further processing. Delayed-response tasks, which tap this aspect of working memory by varying the delay between the presentation and removal of a stimulus and the response, are especially associated with activity of the prefrontal cortex—particularly the dorsolateral parts—in humans and experimental animals (Funahashi, 2006; H. C. Leung et al., 2002, 2005). Brain regions involved in learning and memory: A summary FIGURE 17.18 provides a final update to the map of memory that we’ve been dis- cussing. Several major conclusions should be apparent by now, especially (1) that many regions of the brain are involved in learning and memory; (2) that different forms of memory rely on at least partly different networks of brain mechanisms spanning several different regions of the brain; (3) that any one memory-related brain structure can be a part of the circuitry for several different forms of learning; and (4) that working memory relies on frontal and parietal cortical regions involved in sensory and motor processing. Next we’ll discuss the stages by which memories, of any sort, can be preserved for a lifetime. long-term memory (LTM) An enduring form of memory that lasts STM and LTM involve several different processes days, weeks, months, or years and has a very large capacity. As we have seen, really long-lasting declarative and nondeclarative memories—the address of your childhood home, how to ride a bike, your first kiss—are called long- primacy effect The superior perfor- term memories (LTMs), lasting from days to years. A substantial body of evidence mance seen in a memory task for items at the start of a list; usually attributed to indicates that STM and LTM, in particular, rely on different processes to store in- long-term memory. formation. A classic demonstration involves learning lists of words or numbers. If recency effect The superior perfor- you hear a series of ten words and then try to repeat them after a 30-second delay, mance seen in a memory task for items at you will probably do especially well with the earliest few words, which is termed a the end of a list; attributed to short-term primacy effect; you will also do well with the last few words, termed a recency memory. effect; and you will do less well with words in the middle of the list. FIGURE 17.19 548 CHAPTER 17 (A) 17.19 SERIAL POSITION CURVES FROM IMMEDIATE-RECALL 100 EXPERIMENTS (A) These curves show the percentage of Recency effect correct responses for immediate recall of a list of ten words. The people with amnesia (blue curve) performed as well on the most recent items (8–10) as the control group (red curve) did, but 80 they performed significantly worse on earlier items. (B) Testing Percentage of correct responses immediately after presenting the list prevents the primacy effect, Primacy effect while long delays between presentation and testing block the 60 recency effect, as those last few items are no longer in short- Control term memory. (After Baddeley and Warrington, 1970.) group 40 20 Patients with amnesia 0 1 2 3 4 5 6 7 8 9 10 Item number (B) Increasing delay 100 Mean percentage of correct responses 80 60 0 s delay 10 s 60 s 100 s delay 40 1 2 3 4 1 2 3 4 1 2 3 4 1 2 3 4 Serial position of memory item Immediate tests: Tests after short delay: Tests after longer delay: Recency effect but Both recency and Primacy effect no primacy effect primacy effects but no recency effect shows typical results from such an experiment: a U-shaped serial position curve. If the delay prior to recall is a few minutes instead of a few seconds, there is no recency effect; the recency effect is short-lived and thus attributed to working memory (or STM). The primacy effect, however, lasts longer and is usually attributed to LTM. Like humans, various experimental animals show U-shaped serial position func- tions (A. A. Wright et al., 1985)—a finding that confirms the basic distinction be- tween working memory and LTM. People with amnesia caused by impairment of the hippocampus show a reduced primacy effect but retain the recency effect—their avioral Neuroscience STM8e is intact. Pharmacological manipulations also reveal the different stages of memory, as reviewed in A Step Further: Memories of Different Durations Form dia Group by Different Neurochemical Mechanisms on the website. So how much information do we hold in LTM? It is likely that LTM evolved not to give us a perfect record of our history, but rather to record important events that we can use to shape our future behavior in adaptive ways. It would be a waste of space to record every little detail of our lives, and indeed an important aspect of our memory system is the continual pruning of unimportant memories to preserve cognitive resources (Kuhl et al., 2007). Real-life cases of rare people with perfect recall show that an inability to forget details is confusing and exhausting (Luria, 1987; Parker et al., 2006). Nevertheless, we have the capability to retain enormous amounts of information. We take this capacity for granted and barely notice, for ex- ample, that knowledge of a language involves remembering at least 100,000 pieces of information. Most of us also store a huge assortment of information about faces, LEARNING AND MEMORY 549 1 A subset of the sensory information that 2 If the information is enters sensory buffers is encoded and rehearsed or used, it may be placed into short-term memory (STM). consolidated into long-term memory (LTM), lasting for Performance minutes or up to a lifetime. Short-term memory Long-term (STM)/ Retrieval memory Sensory working (LTM) buffers memory Incoming (e.g., information iconic memory) Encoding Consolidation 3 When we probe a 4 At any stage of the participant’s process, information memory, she must may be forgotten. retrieve informa- Loss of tion from LTM and information place it into STM to perform a task, such as reporting the items in a list. 17.20 HYPOTHESIZED MEMORY PROCESSES: ENCODING, CONSOLIDATION, AND RETRIEVAL A problem at any stage can result in memory failure. encoding A stage of memory forma- tunes, odors, skills, stories, and so on. In one classic experiment, participants viewed tion in which the information entering long sequences of color photos of various scenes; several days later, the participants sensory channels is passed into short- were shown pairs of images—in each case a new image plus one from the previous term memory. session—and were asked to identify the images seen previously. Astonishingly, the participants were highly accurate for series of up to 10,000 different stimuli (Stand- ing, 1973), and researchers have since confirmed that LTM is so vast as to seem boundless (Brady et al., 2008), at least for some types of stimuli (Cunningham et al., 2015). Similar impressive feats of memory in our distant relatives, such as pigeons (Vaughan and Greene, 1984), illustrate that a great capacity for information storage is a general property of nervous systems across the animal kingdom. The system for creating and retaining memories consists of three general stages Breedlove Behavioral Neuroscience 8e Fig. 17.20 ( FIGURE 17.20): 07/20/16 1. Encoding of raw information from sensory channels into short-term memory Dragonfly Media Group Functional brain imaging indicates that while many brain areas participate in the initial processing of stimuli, fewer are associated with successful encod- ing, and these tend to reflect the specific characteristics of the stimuli. So, for example, encoding of the pictorial elements in photos involves greater activation of the right prefrontal cortex and the parahippocampal cortex in both hemi- spheres (Brewer et al., 1998). In the case of words (A. D. Wagner et al., 1998), the critical areas are the left prefrontal cortex and the left parahippocampal cortex. These results indicate that parahippocampal and prefrontal cortex are crucial for consolidation, and these mechanisms reflect the hemispheric specializations (left hemisphere for language and right hemisphere for spatial ability) that we’ll discuss in Chapter 19. Although not depicted in the figure, this model suggests that the flow of information into and out of working memory is supervised by another part of the brain, a central executive, which we will discuss in more detail in Chapter 18. 2. Consolidation of the volatile short-term memories into more-durable long-term memory We know from cases like that of Henry Molaison (who could repeat STM items like word lists) that while the medial temporal lobe apparently is not 550 CHAPTER 17 (A) Encoding (B) Retrieval of memory (C) Retrieval of before it is consolidated consolidated memory Attribute An event to store Visual in memory has Auditory various interlinked Spatial sensory attributes. Retrieval cue Retrieval cue Time 17.21 ENCODING, CONSOLIDATION, AND RETRIEVAL OF DECLARATIVE MEMORIES (A) According to this model, medial temporal lobe processes distribute the various sen- sory attributes of an event, and linkages between them, in corresponding regions of cor- tex. (B) Before consolidation is complete, retrieval involves