PRINCIPLES OF STRATIGRAPHY (GEY 212) PART A&C 2023_2024.docx
Document Details
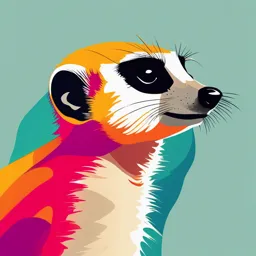
Uploaded by BrainyClover8124
Olabisi Onabanjo University
2024
Tags
Full Transcript
PRINCIPLES OF STRATIGRAPHY Course Outline Stratigraphic Principles Concepts and Procedures Breaks in Stratigraphic record Facies and facies change Sedimentary strata and the Geologic time scale Stratigraphic classification and nomenclature Sedimentary depositional environments Principles of...
PRINCIPLES OF STRATIGRAPHY Course Outline Stratigraphic Principles Concepts and Procedures Breaks in Stratigraphic record Facies and facies change Sedimentary strata and the Geologic time scale Stratigraphic classification and nomenclature Sedimentary depositional environments Principles of correlation Description and the interpretation of the stratigraphic record CONCEPTS OF STRATIGRAPHY Prior to the 1960s, the discipline of stratigraphy was concerned particularly with stratigraphic nomenclature; the more classical concepts of lithostratigraphic, biostratigraphic, and chronostratigraphic successions in given areas; and correlation of these successions between areas. Lithostratigraphy deals with the lithology or physical properties of strata and their organization into units on the basis of lithologic character. Biostratigraphy is the study of rock units on the basis of the fossils they contain. Chronostratigraphy (chrono = time) deals with the ages of strata and their time relations. The concept of depositional sequences, which are packages of strata bounded by unconformities, has gained particular prominence since the late 1970s. This concept has now become so important that we refer to study of sequences as sequence stratigraphy. Two new offshoots of stratigraphy that have made particularly important contributions to our understanding of the physical stratigraphic relationships, ages, and environmental significance of subsurface strata and oceanic sediments are seismic stratigraphy, which is the study of stratigraphic and depositional facies as interpreted from seismic data, and magnetostratigraphy, which deals with stratigraphic relationships on the basis of magnetic properties of sedimentary rocks and layered volcanic rocks. We also recognize sub-branches of stratigraphy such as event stratigraphy (correlation of sedimentary units on the basis of marker beds or event horizons), cyclostratigraphy (study of short-period, high-frequency, sedimentary cycles in the stratigraphic record, particularly by use of oxygen-isotope data), and chemostratigraphy (correlation on the basis of stable isotopes such as oxygen, carbon, and strontium). Application of these new concepts and techniques makes possible subdivision of stratigraphic successions into relatively small units. Such fine-scale stratigraphic resolution is now commonly referred to as high-resolution stratigraphy. As we begin to examine the rock record, we discover that it is preserved and exposed only in certain places, and it is full of gaps. This fact leads to such questions as: What conditions are ideal for the formation and preservation of stratigraphic sequences? How completely do these sequences record geologic history? The limited exposure of outcrops on the modern surface of the Earth means that many deposits of the same age are no longer connected. To reconstruct the geologic history that these outcrops represent, we must ask certain questions: How are these outcrops and environments related in space and time? How can they be correlated, and what is the time significance of these correlations? In addition to correlating outcrops, we can learn much by mapping and plotting the geometry of the rock units under study. Matching the sedimentary properties of rock units is only one way to correlate them. Another important method is biostratigraphy, or correlation by the fossil content of the rocks. Biostratigraphy requires another level of understanding, because the biological properties of the fossils are as important as the physical properties of the rocks that contain them. In recent years, the geophysical properties of strata have become very important in correlation, particularly in the subsurface. Well-logging uses physical properties of the rock that can be detected by devices pulled through drill holes and is the primary tool of modern subsurface correlation. Seismic stratigraphy provides information about subsurface units that could not otherwise be detected. Changes in Earth\'s magnetic field over geologic time have made it possible to correlate rocks by the way they record these changes, giving us the discipline of magnetostratigraphy. The geochemical properties of strata are equally important. The regular pattern of change of certain stable isotopes over geologic time has allowed correlation by this geochemical pattern. However, it is the decay of unstable (radioactive) isotopes that gives us numerical age estimates, rather than mere relative correlation. Integrating these numerical ages with the relative sequence of events recorded in strata is often the most difficult and challenging part of stratigraphy. The geologist not only must develop a correlation framework and put dates into it but also must be aware of the relative strengths and pitfalls of each method. Integrated chronostratigraphy requires the skillful and well-informed use of many disciplines, a training that all geologists should have. LITHOSTRATIGRAPHY Introduction Perhaps the most fundamental kind of stratigraphic study is recognition, subdivision, and correlation (establishing equivalency) of sedimentary rocks on the basis of their lithology, that is, lithostratigraphy. The term lithology is used by geologists in two different but related ways. Strictly speaking, it refers to study and description of the physical character of rocks, particularly in hand specimens and outcrops. It is used also to refer to these physical characteristics: rock type, colour, mineral composition, and grain size are all lithologic characteristics. For example, we may refer to the lithology of a particular stratigraphic unit as sandstone, shale, limestone, and so forth. Thus, lithostratigraphic units are rock units defined or delineated on the basis of their physical properties, and lithostratigraphy deals with the study of the stratigraphic relationships among strata that can be identified on the basis of lithology. However, our observation about rocks need to be set in the context of a time frame work if we are to use them to understand Earth processes and history. Stratigraphy is primarily concerned with the recognition of distinct bodies of rocks and their spatial relationship with each other. Therefore, to understand the concept of lithostratigraphic unit, some stratigraphic principles must be defined because their definition will enable a clear understanding of lithostratigraphic unit and the correlation of lithostratigraphic unit with each other as well as the correlation of rock units with a chronostratigraphic standard which is a formal time framework to which all of Earth geology can be related. Lithostratigraphy, therefore, forms the basis for making geological maps and by correlating lithostratigraphic units, it is possible to reconstruct the changing paleogeography of an area due time. Lithostratigraphy deals with the geostratigraphic change both vertically in layering of varying rock types and laterally reflecting changing environments of deposition known as FACIE CHANGE. Key elements of stratigraphy involves understanding how certain geometric changes between rock layers arise and what this geometry means in terms of depositional environment. A body of rock such as sedimentary, extrusive igneous, metasedimentary or metavolcanic rock can be distinguished by its characteristic and it stratigraphic position relative to other bodies of rock. These are LITHOSTRATIGRAPHIC UNITS. Lithostratigraphic units are the starting point of any stratigraphic study. A lithostratigraphic unit generally conform to the following laws or principles; 1\. PRINCIPLES OF SUPERPOSITION: It states that in any succession of strata not distorted or overturned since, deposition, each layer must be younger than the one below i.e. younger rocks lies above older rocks because a layer of sediment cannot accumulate if there is no substrate on which it can collect. However, sedimentary rocks that are folded intensely to the point that they are overturned violate this law. 2\. PRINCIPLE OF ORIGINAL HORIZONTALITY: This principle was propounded by Steno and it served well in the nascent days of geological sciences. It states that if sediments accumulate in a large basin, the law of gravity would deposit the beds horizontal to the surface of the Earth. If they are deposited on a slope, the sediments would be transported gradually downhill until a horizontal surface is reached. Although it is now known that not all sedimentary layers are deposited purely horizontal. For instance, coarser grain sediments such as sand may be deposited at an angle of up to 15⁰ held up by the internal friction between grains which prevent them slumping to a lower angle. This is known as the angle of repose and a prime example is the surface of sand dunes. Another example is provided by carbonate platform where the inner platform is connected to the adjacent basin by clinoforms. So when we see fold, we know that a rock has undergone deformation. 3\. PRINCIPLE OF LATERAL/HORIZONTAL CONTINUITY: It states that layers of sediments initially extend laterally in all directions, that is, a rock unit continues laterally unless there is a structure or change to prevent it extension. Rocks that are similar but are now separated by a valley or other erosional feature can be assumed to be originally continuous. 4\. PRINCIPLE OF CORSS-CUTTING RELATIONS: It states that if one geologic feature cuts another, the feature that has been cut must be older i.e any unit that has boundaries that cut across other strata must be younger than the rock it cuts. This is most commonly seen with intrusive bodies such as batholiths on a larger scale and magmatic dykes on a smaller scale. This relationship is also seen in sedimentary dykes that form by younger sediments filling a crack in older rocks. The relationship is also seen in faults. 5\. PRINCIPLE OF INCLUSION: It states that if an igneous intrusion contain fragments of another rock, the fragment must be older than the intrusion i.e. a structure that is included in another is older than the including structure. The same relationship holds true for igneous rocks that contain pieces of the surrounding country rock as Xenoliths. This relationship can be useful in determining the age relationship between rock units that are some distance apart. Pebbles of a characteristic lithology can provide conclusive evidence that the source rock type was being eroded by the time that latter unit was being deposited tens or hundreds of kilometres away. 6\. PRINCIPLE OF BAKED CONTACTS: it states that if igneous intrusions baked surrounding rocks, the rock that has been baked must be older than the intrusion. 7\. PRINCIPLE OF UNIFORMITARIANISM: It states that physical processes acting today acted in the past at more or less the same rate and are responsible for the geologic features we see today. That is, the present is the key to the past. Geologists do recognise that the rate of some processes have changed through time because of certain factors such as climate change and occasional catastrophic impacts such as meteor impacts, floods, earthquakes e.t.c. TYPES OF LITHOSTRATIGRAPHIC UNITS Lithostratigraphic units are bodies of sedimentary, extrusive igneous, metasedimentary, or metavolcanic rock distinguished on the basis of lithologic characteristics. A lithostratigraphic unit generally conforms to the law of superposition, which states that in any succession of strata, not disturbed or overturned since deposition, younger rocks lie above older rocks. Lithostratigraphic units are also commonly stratified and tabular in form. They are recognized and defined on the basis of observable rock characteristics. Boundaries between different units may be placed at clearly identifiable or distinguished contacts or may be drawn arbitrarily within a zone of gradation. Definition of lithostratigraphic units is based on a STRATOTYPE (a designated type unit), or type section, consisting of readily accessible rocks, where possible, in natural outcrops, excavations, mines, or bore holes. Lithostratigraphic units are defined strictly on the basis of lithic criteria as determined by descriptions of actual rock materials. They carry no connotation of age. They cannot be defined on the basis of paleontologic criteria, and they are independent of time concepts. They may be established in subsurface sections as well as in rock units exposed at the surface, but they must be established on the basis of lithic characteristics and not on geophysical properties or other criteria. Wheeler and Mallory (1956) introduced the term LITHOSOME to refer to masses of rock of essentially uniform character and having intertonguing relationships with adjacent masses of different lithology. Thus, we speak of shale lithosomes, limestone lithosomes, sand-shale lithosomes, and so forth. Krumbein and Sloss (1963) clarify the meaning of lithosome by asking readers to imagine the body of rock that would emerge if it was possible to preserve a single rock type, such as sandstone, and dissolve away all other rock types. The resulting sandstone body would thus appear as a roughly tabular mass with intricately shaped boundaries. These irregular boundaries would represent its surfaces of contact with erosion surfaces and with other rock masses of differing constitution above, below, and to the sides. Lithosomes have no specified size limits and may range in gross shape from thin, sheet like or blanket like units to thick prisms, or narrow, elongated shoestrings. Stratigraphic units of a single lithology rarely exist as isolated bodies. They are commonly in contact with other rock bodies of different lithology. An important part of lithostratigraphy is identifying and understanding the nature of contacts between vertically superposed or laterally adjacent bodies. Another important aspect is the identification of single lithosomes, groups of lithosomes, or subdivisions of lithosomes that are so distinctive that they form lithostratigraphic units that can be distinguished from other units that may lie above, below, or adjacent. The fundamental lithostratigraphic unit of this type is the formation. A FORMATION is a lithologically distinctive stratigraphic unit that is large enough in scale to be mappable at the surface or traceable in the subsurface. It may encompass a single lithosome, or part of an intertonguing lithosome, and thus consist of a single lithology. Alternatively, a formation can be composed of two or more lithosomes and thus may include rocks of different lithology. Some formations may be divided into smaller stratigraphic units called MEMBERS, which, in tum, may be divided into smaller distinctive units called BEDS. Beds are the smallest formal lithostratigraphic units. Formations having some kind of stratigraphic unity can be combined to form GROUPS, and groups can be combined to form SUPERGROUPS. All formal lithostratigraphic units are given names that are derived from some geographic feature in the area where they are studied. Subdivision of thick units of strata into smaller lithostratigraphic units such as formations is essential for tracing and correlating strata both in outcrop and in the subsurface. An example can be made of the Dahomey basin that consists of the Abeokuta Group and the Imo Group. Abeokuta Group sub-divided into three informal formational units namely Ise, Afowo and Araromi. The Imo Group is subdivided into Ewekoro, Akinbo, Oshosun, Ilaro formation. STRATIGRAPHIC RELATIONS (BREAKS IN STRATIGRAPHIC RECORD) Different lithologic units are separated from each other by contacts, which are planar or irregular surfaces between different types of rocks. Vertically superposed strata are said to be either conformable or unconformable depending upon continuity of deposition. CONFORMABLE strata are characterized by unbroken depositional assemblages, generally deposited in parallel order, in which layers are formed one above the other by more or less uninterrupted deposition. The surface that separates conformable strata is a CONFORMITY, that is, a surface that separates younger strata from older rocks but along which there is no physical evidence of non-deposition. A conformable contact indicates that no significant break or hiatus in deposition has occurred. A HIATUS is a break or interruption in the continuity of the geologic record. It represents periods of geologic time (short or long) for which there are no sediments or strata. Contacts between strata that do not succeed underlying rocks in immediate order of age, or that do not fit together with them as part of a continuous whole, are called UNCONFORMITIES. Thus, an unconformity is a surface of erosion or non-deposition, separating younger strata from older rocks, that represents a significant hiatus. Unconformities indicate a lack of continuity in deposition and correspond to periods of non-deposition, weathering, or erosion, either subaerial or subaqueous, prior to deposition of younger beds. Unconformities thus represent a substantial break in the geologic record that may correspond to periods of erosion or non-deposition lasting millions or even hundreds of millions of years. Contacts are also present between laterally adjacent lithostratigraphic units. These contacts are formed between rock units of equivalent age that developed different lithologies owing to different conditions in the depositional environment. Excluded from discussion here are contacts between laterally adjacent bodies that arise from post depositional faulting. Contacts between laterally adjacent bodies may be gradational, where one rock type changes gradually into another, or they may be intertonguing, that is, pinching or wedging out within another formation. Contacts between Conformable Strata Contacts between conformable strata may be either abrupt or gradational. Abrupt contacts directly separate beds of distinctly different lithology. Most abrupt contacts coincide with primary depositional bedding planes that formed as a result of changes in local depositional conditions, thus, the contacts are commonly very sharp. In general, bedding planes represent minor interruptions in depositional conditions, Such minor depositional breaks, involving only short hiatuses in sedimentation with little or no erosion before deposition is resumed, are called DIASTEMS. Abrupt contacts may be caused also by post depositional chemical alteration of beds, producing changes in colour owing to oxidation or reduction of iron-bearing minerals, changes in grain size owing to recrystallization or dolomitization, or changes in resistance to weathering owing to cementation by silica or carbonate minerals. Conformable contacts are said to be GRADATIONAL if the change from one lithology to another is less marked than abrupt contacts, reflecting gradual change in depositional conditions with time. Gradational contacts may be of either the progressive gradual type or the intercalated type. PROGRESSIVE gradual contacts occur where one lithology grades into another by progressive, more or less uniform changes in grain size, mineral composition, or other physical characteristics. Examples include sandstone units that become progressively finer grained upward until they change to mudstones, or quartz-rich sandstones that become progressively enriched upward in lithic fragments until they change to lithic arenites. Intercalated contacts are gradational contacts that occur because of an increasing number of thin interbeds of another lithology that appear upward in the section. Contacts between Laterally Adjacent lithosomes In addition to vertical boundaries delineated by contacts, stratigraphic units also have finite lateral boundaries. They do not extend indefinitely laterally but must eventually terminate, either abruptly as a result of erosion or more gradually by change to a different lithology. Some sedimentary units are laterally discontinuous in the sense that lateral changes in lithology may occur within single outcrops or at least within a local area. Many non-marine deposits, such as alluvial-fan deposits, exhibit such lateral discontinuity. Lateral changes may be accompanied by progressive thinning of units to extinction-PINCH-OUTS (they are lateral splitting of a lithologic unit into many thin units that pinch-out independently) intertonguing or progressive lateral gradation, similar to progressive vertical gradation. However, when a sedimentary unit do not terminate within a single outcrop or within a local area, it is said to be laterally continuous, although if we still trace it far enough, we would discover that the units also eventually terminate. Many marine strata such as shelf sandstones, limestones, and central-basin evaporites exhibit lateral continuity. Unconformable Contacts Contacts between strata that do not succeed underlying rocks vertically in immediate order of age are called UNCONFORMITIES. Four types of unconformable contacts (unconformities) are recognized: (1) angular unconformity, (2) disconformity, (3) paraconformity, and (4) nonconformity. The first three types of unconformities occur between bodies of sedimentary rock. Nonconformities occur between sedimentary rock and metamorphic or igneous rock.  Angular Unconformity: An angular unconformity is a type of unconformity in which younger sediments rest upon the eroded surface of tilted or folded older rocks; that is, the older rocks dip at a different, commonly steeper, angle than do the younger rocks. The unconformity surface may be essentially planar or markedly irregular. Angular unconformities may be confined to limited geographic areas (local unconformities) or may extend for tens or even hundreds of kilometres (regional unconformities). Some angular unconformities are visible in a single outcrop. By contrast, regional unconformities between stratigraphic units of very low dip may not be apparent in a single outcrop and may require detailed mapping over a large area before they can be identified. Disconformity: An unconformity surface above and below which the bedding planes are essentially parallel and in which the contact between younger and older beds is marked by a visible, irregular or uneven erosional surface is a disconformity. Disconformities are most easily recognized by this erosional surface, which may be channelled and which may have relief ranging to tens of meters. Disconformity surfaces, as well as angular unconformity surfaces, may be marked also by \"fossil\" soil zones (paleosols) or may include lag-gravel deposits that lie immediately above the unconformable surface and that contain pebbles of the same lithology as the lithology of the underlying unit. Disconformities are presumed to form as a result of a significant period of erosion throughout which older rocks remained essentially horizontal during nearly vertical uplift and subsequent downwarping. Paraconformity: A paraconformity is an obscure unconformity characterized by beds above and below the unconformity contact that are parallel and in which no erosional surface or other physical evidence of unconformity is discernible. The unconformity contact may even appear to be a simple bedding plane. Paraconformities are not easily recognized and must be identified on the basis of a gap in the rock record (because of non-deposition or erosion) as determined from paleontologic evidence such as absence of faunal zones or abrupt faunal changes. In other words, rocks of a particular age are missing, as determined by fossils or other evidence. Non-conformity: An unconformity developed between sedimentary rock and older igneous or massive metamorphic rock that has been exposed to erosion prior to being covered by sediments is a nonconformity. Non-conformity surfaces probably represent an extended period of erosion. Significance of Unconformities The presence of unconformities has considerable significance in sedimentological studies. Many stratigraphic successions are bounded by unconformities, indicating that these successions are incomplete records of past sedimentation. Not only do unconformities show that some part of the stratigraphic record is missing, but they also indicate that an important geologic event took place during the time period (hiatus) represented by the unconformity-an episode of uplift and erosion or, less likely, an extended period of non-deposition. VERTICAL AND LATERAL SUCCESSIONS OF STRATA Nature of Vertical Successions Conformities and unconformities divide sedimentary rocks into vertical successions of beds, each characterized by a particular lithologic aspect. Different types of beds can succeed each other vertically in a great variety of ways, and distinctions can be drawn between rock units characterized by lithologic uniformity, lithologic heterogeneity, and cyclic successions. Rock units that have complete lithologic uniformity are rare, although many beds may display a high degree of uniformity in colour, grain size, composition, or resistance to weathering. Beds that are most likely to be uniform are fine-grained sediments deposited slowly under essentially uniform conditions in deeper water, or coarser sediments that have been deposited rapidly by some type of mass sediment transport mechanism such as grain flow. By contrast, heterogeneous bodies of sedimentary strata are characterized by internal variations or irregularities in properties. Heterogeneous units may include strata such as extremely poorly sorted debris-flow deposits, as well as thick units broken internally by thinner beds characterized by differences in grain size or bedding features. Cyclic Successions Many stratigraphic successions display repetitions of strata that reflect a succession of related depositional processes and conditions that are repeated in the same order. Such repetitious events are referred to as cyclic sedimentation or rhythmic sedimentation. Cyclic sedimentation leads to the formation of vertical successions of sedimentary strata that display repetitive orderly arrangement of different kinds of sediments. The term cyclic sediment has been used for a wide variety of repetitious strata including such small-scale features as presumed annually deposited VARVES in glacial lakes as well as large-scale sediment cycles caused by long-period, recurring migration of depositional environments. Other common examples of repetitive deposits include rhythmically bedded turbidites, laminated evaporite deposits, limestone-shale rhythmic successions, coal cyclothems (repeated cycles involving coal deposition), black shale deposits, and chert deposits. Cyclic successions occur on all continents in essentially every stratigraphic system. They are produced by processes that range in geographic scope and duration from very local, short-term events-such as seasonal climatic changes that generate varves-to global changes in sea level that may involve entire geologic periods. On the basis of the mechanisms that form cyclic deposits, two kinds of cyclic successions are recognized: autocyclic and allocyclic. AUTOCYCLIC successions are controlled by processes that take place within the basin itself, and their beds show only limited stratigraphic continuity. Examples include non-periodic storm beds and turbidites. ALLOCYCLIC successions are caused mainly by variations external to the depositional basin. Fundamentally, these variations are caused by changes in climate and tectonic movements. For example, climate can influence sea level, waxing and waning of continental glaciers, and sedimentary processes such as deposition of evaporites. ASSIGNMENT- Differentiate between first, second and third order cyclic deposits SEDIMENTARY DEPOSITIONAL ENVIRONMENTS A depositional sedimentary environment is a geomorphic setting in which sediments accumulate. Such a setting is characterized by a unique set of physical and biological processes operating at a specified rate and intensity that impact sufficient imprint on the sediment to produce a characteristic deposit. The character of the sediment so produced is determined by the intensity of the formative processes operating on it and by the duration through which such action is continued. Therefore, deposition in a given environmental sedimentary setting produces a sedimentary facies characteristic of that specific environment. Sedimentary rocks occur in a wide range of environment on the Earth's surface and the simplest and broadest of such environment is into Continental, Transitional and Marine. Although this classification is too simplistic, each classes is sub divisible into several sub environment and environmental variance as shown below: ENVIRONMENTAL ASSOCIATION ENVIRONMENT SUB-ENVIRONMENT/ENVIRONMENTAL VARAINTS --------------------------- ---------------------------------- ------------------------------------------------------------------------------------------------------------------------------------------------------------ CONTINENTAL Desert Wadi, e.g., interdunes, playa, Duricrusts Alluvial fan Fan head channel, proximal fan, distal fan, suprafan lobe Alluvial plane and riverine cone Braided channel, meandering channel, levee, crevacae splay, flood plain Lake Saline, temperate stratified, tropical stratified, glacial, delta plain, lake terrace, shoreline, slope, basin, delta Glacial and periglacial Supraglacial (flow tilt), subglacial (lodgement till), intraglacial (melt and till), morainic complex, outwash fan, glacial fan, glacial lakes, eskers COASTAL SHELF Delta Distributory channel, tidal channel, back swamp, bay, mouth bar, pro delta Estuary Estuarine channel, marginal flats, flow tidal delta Linear elastic shoreline Beach, near shore, offshore, barrier, lagoon, tidal flats, tidal delta, tidal inlets, coastal aeolian dunes Carbonate-evaporite Sabkha, algal marsh, tidal flat, beach, lagoon, tidal delta, platform margin Shoreline and shelf basin Marginal build ups, deep basins, evaporite basins Clastic shelf (tide dominated, weather dominated), various tidal bedforms, sand ribbons, linear tidal ridges, sand waves, shoal retreat massifs, buried channels, scarps OCEANIC Passive margin Continental slope, continental rise, abyssal plain, submarine fans, submarine channels Active margin Trench, subduction complex, perched basin, fore-arc basin, back-arc basin fan Oceanic pelagic Mid-oceanic ridge, ridge flank, abyssal plain (hypersaline ocean, euxinic ocean) This subdivisions of environment into sub classes are often broadly correlative to facie model, that is, each facie model is a general summary of a given depositional system. A clear relationship thus exists between sedimentary environment and the resulting sedimentary facies. This relationship is basically that of cause and effect or that of a series of processes resulting in identifiable product  In facies analysis, especially in environmental diagnosis, several lines of evidence are critically evaluated although not all the desirable data are usually available especially in subsurface studies based on well log. More direct data is often available from outcrop studies. In diagnosing environment of deposition, that is, identifying how a sediment was deposited, the basic approach is to observe, characterize or document the defining parameters of the facies. These defining parameters include and not limited to: Geometry Lithology Sedimentary structures Paleocurrent Fossils Texture These observations are interpreted by comparing with modern environmental settings. The information obtained thereafter will allow for the prediction of the attributes of economic materials of interest. GEOMETRY: The geometry of a sedimentary facie is the overall shape which is a function of the depositional topography, sediment preservation potential and tectonic deformation. LITHOLOGY: This has to do with composition or mineralogy. For example, you may have sediment of chemical composition e.g. limestone or clastic composition e.g. sandstone or shale. In some cases, component mineral are diagnostic. For example, Glauconite in sandstone indicate marine while kaolinite suggest continental environment. Limestone often contain fossil and other evidence of marine composition. SEDIMENTARY STRUCTURES: This is invariably generated Insitu. They can indicate bathymetry, energy of environment or flow direction. In some cases, they may consist of physical, chemical and biogenic substances that are environment diagnostic. PALEOCURRENT PATTERNS: This is usually derivative rather than observational. This parameter is measured from directional characteristics and is structured into paleocurrent map and integrated with other lines of evidence to derive depositional environment and paleogeography. This is severally restricted in subsurface studies. However, dip metre data may yield paleocurrent evidence. FOSSILS: This is often diagnostic and they are particularly useful in subsurface analysis. Many fossils indicate various environmental parameters such as bathymetry, temperature, salinity, climate and current turbulence. The presence of ammonite, foraminifera and nannoplanktons is suggestive of marine. TEXTURE: This has to do with the grains. In clastics, the vertical diminution or increase in grain size is often indicative of the depositional setting. CORRELATION OF LITHOSTRATIGRAPHIC UNITS In the simplest sense, stratigraphic correlation is the demonstration of equivalency of stratigraphic units. Correlation is a fundamental part of stratigraphy, and much of the effort by stratigraphers that has gone into creating formal stratigraphic units has been aimed at finding practical and reliable methods of correlating these units from one area to another. A broader interpretation of correlation allows that equivalency may be expressed in lithologic, paleontologic, or chronologic terms. The 1983 North American Stratigraphic Code recognizes three principal kinds of correlation: 1\. Lithocorrelation, which links units of similar lithology and stratigraphic position 2\. Biocorrelation, which expresses similarity of fossil content and biostratigraphic position 3\. Chronocorrelation, which expresses correspondence in age and chronostratigraphic position Lithostratigraphy and correlation Correlation in stratigraphy is usually concerned with considering rocks in a temporal framework, that is, we want to know the time relationships between different rock units -- which ones are older, which are younger and which are the same age. Correlation on the basis of lithostratigraphy alone is difficult because lithostratigraphic units are likely to be diachronous. If we can draw a time-line (imaginary lines drawn across and between bodies of rock which represent a moment in time) across our rock units, or, more usefully, a time-plane through an area of different strata, we would be able to reconstruct the distribution of palaeoenvironments at that time across that area. To carry out this exercise of making a palaeogeographic reconstruction we need to have some means of chronostratigraphic correlation, a means of determining the relative age of rock units which is not dependent on their lithostratigraphic characteristics. Radiometric dating techniques provide an absolute time scale but are not easy to apply because only certain rock types can be usefully dated. Biostratigraphy provides the most widely used time framework, a relative dating technique that can be related to an absolute time scale, but it often lacks the precision required for reconstructing environments and in some depositional settings appropriate fossils may be partly or totally absent (in deserts, for example). Palaeomagnetic reversal stratigraphy provides timelines, events when the Earth's magnetism changed polarity, and may be applied in certain circumstances. The concept of sequence stratigraphy provides an approach to analysing successions of sedimentary rocks in a temporal framework. The composition of sediments is variable, so differences in the mineralogical and chemical character of sedimentary rocks are potentially a way of discriminating and correlating deposits. This approach, known as chemostratigraphy, will work in circumstances where there is variation in the mineralogy, and hence chemistry, of sediment being supplied to the area of sedimentation, that is, there are changes in provenance. Lithologic Similarity and Stratigraphic Position Lithologic similarity can be established on the basis of a variety of rock properties. These properties include gross lithology (e.g., sandstone, shale, or limestone), colour, heavy mineral assemblages or other distinctive mineral assemblages, primary sedimentary structures such as bedding and cross-lamination, and even thickness and weathering characteristics. The greater the number of properties that can be used to establish a match between strata, the stronger the likelihood of a reliable match. A single property such as colour or thickness may change laterally within a given stratigraphic unit, but a suite of distinctive lithologic properties is less likely to change. DESCRIPTION AND THE INTERPRETATION OF THE STRATIGRAPHIC RECORD SEISMIC, SEQUENCE AND MAGNETIC STRATIGRAPHY Seismic stratigraphy is thus the study of seismic data for the purpose of extracting stratigraphic information. Seismology is the study of earthquakes and the structure of Earth on the basis of the characteristics of seismic waves. Sequence stratigraphy is an outgrowth of seismic stratigraphy, although the practice of sequence stratigraphy is not limited to study of seismic records. A sedimentary sequence is a stratigraphic unit composed of a relatively conformable succession of genetically related strata that is bounded a t its top and base by unconformities or their correlative conformities. A sequence represents one cycle of deposition bounded by non-marine erosion, deposited during one significant cycle of rise and fall of base level. Because base level in marine basins is controlled by sea level, a sequence is thus the product of a cycle of rise and fall of sea level. Magnetostratigraphy is also a relatively new branch of stratigraphy. It is based on the principle that iron-bearing minerals such as magnetite become magnetized at the time they crystallize in a magma or lava flow. The magnetized minerals acquire magnetic polarity (north and South Pole alignments) in keeping with Earth\'s magnetic polarity, a property called remanent magnetism. Patterns of magnetic reversals in ancient sedimentary rocks constitute a powerful tool for stratigraphic subdivision of these rocks, which can be applied to a variety of geologic problems such as correlation, geochronology (study of time in relation to Earth history), and paleoclimatology. Seismic Sequence Analysis The term sequence is often used informally by geologists to refer to any grouping or succession of strata. Sequence is also used in a more restricted sense to identify distinctive stratigraphic units that are commonly bounded by unconformities (i.e., similar to allostratigraphic units. Sloss (1963) considered sequences to be major rock-stratigraphic units of interregional scope that are separated and delimited by interregional unconformities. The sequence concept was subsequently extended and redefined by Mitchum, Vail, and Thompson (1977). These authors define a depositional sequence as \"a stratigraphic unit composed of a relatively conformable succession of genetically related strata and bounded at its top and base by unconformities or their correlative conformities.\" Sequences as thus defined differ from Sloss\'s sequences in that they may be much smaller rock units (a few tens of meters to as much as a thousand meters; Wilson, 1992). Also, because they are bounded by interregional unconformities and their equivalent conformities, they may be traceable over major areas of ocean basins as well as continents. The strata that make up a depositional sequence may be either CONCORDANT, that is, essentially parallel to the sequence boundary, or DISCORDANT, lacking parallelism with respect to the sequence boundaries. Concordant relations can occur at either the upper or the lower boundary of a sequence and may be expressed as parallelism to an initially horizontal, inclined, or uneven surface. Discordance is the most important physical criterion used in determining sequence boundaries. Strata may display discordance with overlying beds (top discordance) or underlying beds (base discordance). EROSIONAL TRUNCATION is the lateral termination of strata because erosion has cut them off from their original depositional limits. Truncation occurs at the upper boundary of a sequence and may be of either local or regional extent. TOPLAP is lapout (lateral termination of strata against a boundary at their original depositional limit) at the upper boundary of a depositional sequence, for example, the lateral termination updip of the foreset beds of a deltaic complex. Toplap is evidence of a nondepositional hiatus. TOPLAP is believed to be the result of a depositional base level, such as sea level, being too low to permit the strata to extend farther updip, thus allowing sedimentary bypassing and possibly minor erosion to occur above base level while prograding strata are deposited below base level. BASELAP occurs at the lower boundary of a depositional sequence and may itself be of two types. Onlap is baselap in which an initially horizontal or inclined stratum terminates against a surface of greater inclination. Downlap is baselap in which an initially inclined stratum terminates down dip against an initially horizontal or inclined surface. Onlap and downlap indicate non-depositional hiatuses and not erosional breaks in deposition. In seismic stratigraphic analysis, however, two kinds of discontinuities are recognized: 1\. Erosional unconformity surfaces that represent a significant hiatus owing to subaerial or subaqueous erosional truncation 2\. Unconformable surfaces called downlap surfaces, which are marine surfaces representing a hiatus but without evidence of erosion ASSIGNMENT Draw a sketch of a seismic section showing the following: toplap, onlap, downlap, erosional truncation, offlap. FUNDAMENTAL PRINCIPLES IN SEQUENCE STRATIGRAPHY The entire three-dimensional assemblage of lithofacies enclosed within sequence boundaries is referred to as a depositional system. Depositional systems, in tum, are made up of smaller stratigraphic units: system tracts and parasequences (which are arranged into parasequence sets). Sequence stratigraphy sets out to place these stratal units into a predictable, chronostratigraphic framework by demonstrating how their generation is related to accommodation space. Accommodation space is the space available at any point in time in which sediments can accumulate. Fundamentally, it is the space between a conceptual equilibrium surface that separates erosion from deposition in which potential sediment can accumulate. In the marine realm, this equilibrium surface, called base level, is sea level. Accommodation in marine settings is governed by eustasy (sea-level rise and fall) and tectonism (subsidence/uplift). Relative rise in sea level or subsidence of the seafloor creates more sedimentation space. Fall in sea level or elevation of the seafloor decreases accommodation. Parasequences are the smallest facies units of depositional sequences and range in thickness from about 10 to 100 m. A parasequence is a small-scale succession of beds or bed sets bounded by flooding surfaces, which are surfaces that separate younger strata from older strata, across which there is evidence of an abrupt increase in water depth. They commonly form in coastal environments where the rate of increase of accommodation space is less than the rate of sediment supply. System tract: a subdivision of a depositional system. Four main kinds are recognized: highstand (sediment deposited during high sea level), falling-stage (sediment deposited as sea falls from high to low), lowstand (sediment deposited during low sea level and early rising sea level), and transgressive (sediment deposited during rising sea level). Parasequence Set- a succession of genetically related parasequences that form a distinctive stacking pattern that is bounded, in many cases, by major marine flooding surfaces and their correlative surfaces. Parasequence-a relatively conformable succession of genetically related beds or bedsets (within a parasequence set) bounded by marine flooding surfaces or their correlative surfaces. Marine flooding surface-a surface that separates younger from older strata, across which there is evidence of an abrupt increase in water depth. Under different conditions of relative sea-level rise and fall, parasequences may advance in a landward direction to form retrogradational parasequence sets or build vertically to generate aggradational parasequence sets. ASSIGNMENT Define the following terms in sequence stratigraphy Flooding surface, Maximum flooding surface, transgressive surface, regressive surface of erosion, aggradation, progradation. BIOSTRATIGRAPHY The characterization and correlation of rock units on the basis of their fossil content is called biostratigraphy. Stratigraphy based on the paleontologic characteristics of sedimentary rocks is also referred to as stratigraphic paleontology, the study of fossils and their distributions in various geologic formations. The concept of biostratigraphy is based on the principle that organisms have undergone successive changes throughout geologic time. Thus, any unit of strata can be dated and characterized by its fossil content. That is, on the basis of its contained fossils, any stratigraphic unit can be differentiated from stratigraphically younger and older units. Concept of Stage d\'Orbigny defined stage as groups of strata containing the same major fossil assemblages. He named these stages after geographic localities with particularly good sections of rock that bear the characteristic fossils on which the stages are based. Using the stage concept, he was able to divide the rocks of the Jurassic system into ten stages and the Cretaceous rocks into seven stages, each characterized strictly by its fossil fauna. The boundaries of d\'Orbigny\'s stages were defined at intervals marked by the last appearance, or disappearance, of distinctive assemblages of life forms and their replacement in the rock record by other assemblages. Concept of Zone The stage concept of d\'Orbigny permitted subdivision of strata into major successions on the basis of fossils. What they did not provide was a method by which fossiliferous strata could be divided into small-magnitude, clearly delimited units. Oppel introduced the concept of zone in 1856 and thereby altered for all time the practice of biostratigraphy. He conceived the idea of small-scale units defined by the stratigraphic ranges of fossil species irrespective of lithology of the fossil-bearing beds. Oppel noted that the vertical ranges of some species were very short; that is, the species existed for only a very short time geologically. Others were quite long, but most were of some intermediate length. a zone represents the time between the appearance of species chosen as the base of the zone and the appearance of other species chosen as the base of the next succeeding zone, recognition of zones thus permits delineation of clear-cut, small-scale time units. Each of Oppel\'s zones was named after a particular distinctive fossil species, called an INDEX FOSSIL, or index species, which is but one fossil species in the assemblage of species that characterize the zone. Principal Categories of Zones Revisions to the 1983 North American Stratigraphic Code subdivides biostratigraphic units into five principal kinds of biozones: range biozones (two kinds), interval biozones (two or more kinds), lineage biozones, assemblage zones, and abundance biozones. Each of these zones is distinguished by different criteria, as explained below. A taxon-range biozone is a body of rock representing the known stratigraphic and geographic range of occurrence of a single taxon. A concurrent range biozone is a body of rock that includes the concurrent, coincident, or overlapping part of the ranges of two specified taxa. An interval biozone, or subzone, is the body of strata between two specific, biostratigraphic surfaces. The features on which biohorizons are commonly based include lowest occurrences however, they may also include distinctive occurrences and changes in the character of individual taxa, such as changes in the direction of coiling in foraminifers or in number of septa in corals. A lineage biozone is a body of rock containing species representing a specific segment of an evolutionary lineage. An assemblage biozone is a body of rock characterized by a unique association of three or more taxa, the association of which distinguishes it in biogeographic character from adjacent strata. An assemblage biozone may be based on a single taxonomic group, for example, trilobites, or on more than one group, such as acritarchs and chitinozoans. An abundance biozone is a body of rock in which the abundance of a particular taxon or specified group of taxa is significantly greater than in adjacent parts of the section. Other terminologies First appearance- members of a new species increasing in numbers, so that they may eventually become abundant and widespread enough to show up in the geologic record. Last appearance- is when the species is no longer able to adjust to shifting environmental conditions and its members decrease and eventually disappear. Extinction- refers to the disappearance by death of every individual member of a species or higher taxonomic group so that the lineage no longer exists. Paleontologists recognize also that a species may experience pseudoextinction. Pseudoextinction, or phyletic extinction, refers to an evolutionary process whereby a species evolves into a different species. Thus, the original species becomes extinct, but the lineage continues in the daughter species. Particularly important and useful fossils for biostratigraphic purposes are called GUIDE FOSSILS or INDEX FOSSILS. Ideally, index fossils should be (1) independent of their environment, (2) fast evolving, (3) geographically widespread, (4) abundant, (5) readily preserved, and (6) easily recognizable. ASSIGNMENT What is mass extinction? Give a very brief but concise information about the major mass extinction episodes with the probable causes and number of species (or genera) affected.