Respiratory Physiology PDF
Document Details
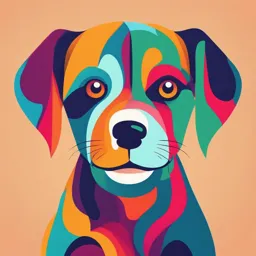
Uploaded by EruditeTurquoise9432
Tags
Summary
These lecture notes detail respiratory physiology, encompassing the introduction to the respiratory system, the organization of the respiratory system with a focus on the upper airways, trachea, and lungs, and the importance of alveoli. The notes emphasize the functions of the respiratory system, the respiratory membrane, and the process of gas exchange. This document is a set of lecture notes.
Full Transcript
Respiratory Physiology Lecture 1 recording 1: Introduction to the Respiratory System ∙ Respiratory Physiology: ▪ Respiratory physiology is the study of how oxygen is brought into the lungs and then delivered to the tissue and how carbon dioxide is eliminated from the...
Respiratory Physiology Lecture 1 recording 1: Introduction to the Respiratory System ∙ Respiratory Physiology: ▪ Respiratory physiology is the study of how oxygen is brought into the lungs and then delivered to the tissue and how carbon dioxide is eliminated from the tissue and from the system ∙ Functions of the Respiratory System: ▪ Functions: o Provides oxygen (O2) and eliminates carbon dioxide (CO2) (Homeostatic regulation of blood gases) → gas exchange from the periphery to the external environment o Protects against microbial infection and toxic agents that can be inhaled during each respiratory cycle (Filtering action) o Regulates blood pH (In coordination with the kidneys - renal system) o Contributes to phonation - the passage through the vocal cords of air allowing for speech formation o Contributes to olfaction - sense of smell → when air passes through the nasal cavity, it allows for the molecules to come into contact with the epithelium contributing to olfaction o Is a reservoir for blood (hosts large volume of blood in a given respiratory cycle that is available for gas exchange)→ continuous exchange of the gasses oxygen and carbon dioxide between the alveoli and the blood system ∙ Organization of the Respiratory System: ▪ Respiratory system contains components which are found outside the lungs ▪ Lungs have a very ramified, branched airway system that leads air up to the alveoli - Upper airways are gateway to environment - Air enters through the nasal and oral cavities and passes through the pharynx and larynx → trachea then lungs - Bronchi –> bronchioles → alveoli o Fundamental unit of the respiratory system – alveoli The alveoli are embedded in a dense network and tissue characterized by the presence of smooth muscle tissue, smooth muscle cells and connective tissue → pulmonary circulation Large number of capillaries in lungs - Muscle of respiration allow for continuous change in pressure and volume - Rib cage and pleura protects the lungs and give more stability allowing for more changes in pressure and volume - Part of CNS that regulates respiration (mainly located in brainstem) → influences activity of respiratory muscles ∙ Upper Airways: ▪ Air enters through the nasal cavity and oral cavity in the upper airway → air is warm and humidified (composition changes after) ▪ Pharynx: composed of the nasopharynx and the laryngopharynx ▪ Larynx: contains the vocal cords (phonation - production of speech sounds) ▪ Trachea ∙ Trachea & Lungs: ▪ Air passage: larynx → trachea → two primary bronchi → lungs ▪ Trachea and primary bronchi: o Are characterised by a structure that is semi-cartilaginous C-shaped ring (made of cartilage) in front (anteriorly) and smooth muscle in the back (posteriorly) o This provides protection for the airway and gives elasticity ▪ Bronchi: o Still have some cartilaginous structures but the air pathways are no longer C shaped C-shaped rings of cartilage are replaced by plates of cartilage and smooth muscle characterizes the shape and the elastic properties - After further division, there are no more cartilage ▪ Bronchioles: o Structure is provided by smooth muscle Lecture 1 recording 2: Respiratory and Conducting Zones ∙ Respiratory and Conducting Zones: o At each branch there is a reduction in the bronchiole size o The tracheobronchial tree can be classified and organized into two areas: conducting zone and respiratory zone Conducting zone → contains the trachea, primary bronchi (splitting of primary bronchi and beginning of branchi), bronchioles and terminal bronchioles ❖ Called the conducting zone because in these areas there are no alveoli and as a result no gas exchange occurs at the level of the conducting zone ❖ This area is called an anatomical dead space because it is not available for gas exchange Respiratory zone → further down in the branching of the airways and contains the alveoli ❖ Alveoli: initially sparse and not very numerous but become more and more numerous with the ramification or the branching ❖ Contains the respiratory bronchioles, alveolar ducts and alveolar sacs ❖ Where gas is exchanged ✔ Air that enters the respiratory zone is available for gas exchange o Terminal bronchioles → smallest airways without alveoli o Respiratory bronchioles → have occasional alveoli ∙ Respiratory and Conducting Zones: o Respiratory bronchioles → sparse occasional alveoli o Alveoli sacs → contain a large number of alveoli ∙ Changes in Cross-sectional Area of Airways through Successive Generations of Branching: (You do not need to memorize the details of this chart, only what is in the text below) ▪ Tracheobronchial tree: o Each branching is called a generation o Begin at generation 0 at the trachea and arrive at generation 23 at the level of the alveolar sacs o Progression of generations in both the diameter and the length of the airways at each generation decrease Diameter and length decrease from the level of the trachea to the alveolar sacs o The number of branches at each specific generation level tends to increase Airways are smaller but more numerous towards the alveolar sacs o Total cross sectional area, or the surface area, increases towards the alveolar sacs → increases surface area for gas exchange Lecture 1 recording 3: The Alveoli ∙ Alveoli in the Lung: ▪ Alveoli: - Very important for the function of the respiratory system o Tiny sacs with a very thin wall o Highly vascularised → many capillaries that contact the alveolar surface o Amount of blood in capillaries is variable and changes with metabolic demand - Netting around the sacs → netting made of capillaries that is ready to continue to contact the alveolar surface - 500 million alveoli in the human lung, each ~⅓ mm in diameter, presence of capillaries is extremely intense at the level of alveolar sacs - 280 billion capillaries in the lung (at rest they contain 70mL of blood, 200 mL during physical activity) → amount of blood will increase with physiological demand (when exercising because need for more oxygen and elimination of carbon dioxide) - Amount of blood is variable and changes with metabolic demand ∙ Alveoli: - Cross section of alveolus - Cells that line the alveolar sac are very flat (type 1) ▪ Type I alveolar cells: o Flat epithelial cells o The internal surface of the alveoli is lined with liquid that contains a surfactant Surfactant is very important for stabilization of the alveolar sac o Do not divide; susceptible to inhaled or aspirated toxins ▪ Type II alveolar cells: o Not found frequently in the alveoli o Functions: 1. Produce the surfactant which is very important for respiratory function - Structure of alveolus with just a single layer of cells is very fragile - If toxic agents survived in the alveoli through inhalation, the type one alveolar cells could easily be damaged 2. Can act as progenitor cells - have the ability to replicate and differentiate into Type I alveolar cells, which are produced in the late stage of development and stop replicating; they cannot be replaced when damaged by inhaled toxic agents ❖ Potential for fixing damaged alveoli ∙ Alveoli: ▪ Highly vascularized o Capillaries (that are in between the alveolar ducts/sacs) are very small (allow only a single RBC at a time) o Estimated that in less than 1 second a RBC passes through the pulmonary capillary system - RBC does not spend much time in the process of respiration - During this time, it traverses 2-3 alveoli o This is sufficient time for oxygen and carbon dioxide diffusion between the alveoli and the capillary cells ∙ O2 and CO2 Transfer between Alveolar Air and Blood: ▪ Oxygen and carbon dioxide diffuse through the respiratory membrane (0.2 - 0.5 uM thick) o Oxygen diffuses from the alveoli to the bloodstream and carbon dioxide diffuses from the bloodstream to the alveoli (gas exchange) ▪ Respiratory membrane → respiratory surface made of the alveolar epithelial cell (Type I alveolar cells) and the pulmonary capillary endothelial cell o Alveolar fluid (with surfactant), alveolar epithelium, basement membrane of alveolar epithelium, interstitial space, basement membrane of capillary endothelium, capillary endothelium o Very thin, easily damaged - High blood pressure will tend to distant at the level of respiratory circulation, distance capillaries, if epithelial breaks, plasma and RBC can enter lungs and affect respiration - If intubation is needed, do not want air pushing with too much pressure because could create extreme distension of the alveoli and damage its surface allowing plasma and RBC to enter the lungs ▪ Pneumocyte → one of the cells lining the alveoli of lung (type I and type II alveolar cells) Lecture 1 recording 4: Steps of Respiration ∙ Steps of Respiration: ▪ Respiration: o Ventilation → consists of movement of the gas from the atmosphere to the alveoli by bulk flow, independent of the gas composition Movement is generated due to changes in volume and pressure that will push, or will promote, movement from areas of high pressure to areas of low pressure o Exchange of oxygen and carbon dioxide between the alveoli and the blood system by diffusion Occurs at the level of the respiratory membrane due to changes in pressure of oxygen and carbon dioxide between the alveoli and the blood o Transport of oxygen and carbon dioxide through pulmonary and systemic circulation by bulk flow o Exchange of oxygen and carbon dioxide between blood in tissue capillaries and cells in tissues by diffusion The differential pressure between the blood and the peripheral tissue will drive oxygen from the blood to the peripheral tissue and carbon dioxide will move from the peripheral tissue to the blood to be eliminated at the next respiratory process o Cellular utilization of oxygen and production of carbon dioxide during cellular respiration ∙ How is Respiratory Airflow (Ventilation) Produced?: o Ventilation: - Movement of gas from the environment to the alveoli CNS sends an excitatory drive to respiratory motor neurons that innervate the respiratory muscles Respiratory muscles contract rhythmically and in a very organized pattern Changes the thoracic volume, the thoracic pressure, and intrapulmonary pressures ❖ These changes involving pressure allow for gas movement Air flows in and out with the different muscle contractions and relaxations ∙ Respiratory Muscles: ▪ 3 categories of muscles involved in respiration: pump muscles, airway muscles and accessory muscles o Pump muscles: make changes in pressure and volume at the level of the lungs 2 classes which allow air to come in and out: ❖ Inspiratory (INS) → active throughout inspiration ✔ Main muscle: diaphragm - Diaphragm, external intercostals, parasternal intercostals ❖ Expiratory (EXP) → active throughout expiration - Internal intercostals, abdominals - Brain must coordinate the activity of various muscle groups to produce efficient breathing o Airways muscles: muscles located at the level of the airways and have an important role in keeping the upper airways open Mostly inspiratory but there are some that are active in expiration - INS: tongue protruders (genioglossus), alae nasi, muscles around airways (pharynx, larynx) - EXP: muscles are airways (pharynx, larynx) - Accessory muscles o Accessory muscles: facilitate respiration during (eg) exercising, when there is an increased metabolic drive - INS: sternocleidomastoid, scalene ▪ Brain coordinates the activity of various muscle groups for breathing Lecture 1 recording 5: Respiratory Muscles ∙ Respiratory Muscles: (This figure lists muscles of inspiration and expiration. The muscles you will need to know will be discussed in the rest of the slides for this section.) ∙ Inspiratory Pump Muscles: Diaphragm ▪ Diaphragm: o Most important muscle for respiration Active during inspiration: dome-shaped structure, separates the lungs from the abdominal content - Flattens during contractions (INS), abdominal contents are forced down and forward and rib cage is widened o When the diaphragm contracts, it moves down, allowing the abdominal content to be pushed down, and the rib cage to be pushed outward, or widened; overall effect is an increase in the thoracic volume when it contracts ∙ Inspiratory Pump Muscles: External Intercostal Muscles ▪ External intercostals: o Inspiratory pump muscles o Contract and lift the rib cage, and this action promotes a lateral increase in the thoracic volume → pushes abdominal contents down and expands the thoracic cage Contract and lift the rib cage to promote a lateral increase in the thoracic volume ❖ Contribute to the expansion of the thorax The motion of the rib cage caused by contraction of the external intercostal muscles is very similar to the motion of a bucket handle, and is called the bucket handle motion ∙ Inspiratory Pump Muscles: Parasternal Intercostal Muscles: ▪ Parasternal intercostals: - Very close to the sternum o Inspiratory pump muscles o Contract and pull sternum forward, increasing anterior posterior dimension of the rib cage o Pump handle motion → similar to movement of water pump ∙ Expiratory Pump Muscles: Abdominals: (you do not need to memorize the names of the abdominal muscles….just “abdominal muscles” as expiratory pump muscles) ▪ Inspiratory pump muscles: active all the time during inspiration (during inspiration at rest or during exercise) ▪ Expiratory pump muscles: o Do not contract during expiration at rest and passive during inspiration o Active during the expiratory phase when you are making an effort to breathe in and out (ie. During stress, exercise, coughing) ▪ Deeper, faster breathing requires active contraction of abdominal & internal intercostal muscles to return the lung to its resting position (Exercise) ∙ Expiratory Pump Muscles: Internal Intercostal Muscles: ▪ Internal intercostals: o Expiratory pump muscles o Relaxed at rest and recruited during forced expiration o Push the rib cage down to reduce the amount of air or reduce the volume of the thoracic cage ∙ Accessory Inspiratory Muscles: (You do not need to know the 2 muscles and what each specifically does, just what is below) ▪ Not commonly active during resting breathing; active during exercise and forced respiration Lecture 1 recording 6: Inspiration and Expiration ∙ Inspiration at Rest and During Forced Respiration: ▪ Quiet inspiration (rest): diaphragm (contracts, pushing the abdominal content down and expanding the thorax as air comes in) - operated in conjunctions with the external intercostals and parasternal intercostals ▪ During exercising or deep breathing: stronger contraction of the diaphragm and recruitment of the accessory muscles, further expanding the thoracic cavity ∙ Expiration at Rest and During Forced Expiration: ▪ Quiet expiration (rest): at resting level, the abdominal and intercostal muscles are not active o During quiet resting expiration, no muscles are recruited (no active contraction of the respiratory muscles) The inspiratory muscles relax and air moves out of the lungs because of the recoil of the lungs ▪ During forced expiration, abdominal muscles contract intensely o This causes the abdominal content to be pushed upward so that the diaphragm is moved even higher than its resting level and more air is expelled o Internal intercostal muscles contract and push the rib cage down ∙ Upper Airway Muscles: ▪ Most of the upper airways muscles are inspiratory; only a few are also expiratory o Upper airway muscles are usually active during inspiration o Main function is to keep the upper airways open o Maintain the airway and allow for gas exchange - When closed, can still contract but no gas exchange would occur - Several muscles contribute to opening the upper airways and reduce airway resistance (You do not need to memorize the muscles listed on the slide) ∙ Obstructive Sleep Apnea: ▪ Upper airway muscles: o State-dependent: when you sleep, the activity of these muscles tends to be depressed o Healthy patient: Air passes through the nasal and oral cavities and the tone of the tongue is maintained o Obstructive sleep apnea: Tone of the upper respiratory muscles is depressed and they become a floppy muscle Reduction in upper airway patency during sleep, or a reduction in the openness of the airway Air cannot go in and out, especially during sleep, resulting in snoring and large drops in oxygen saturation in the blood - occlusion continue Consequences of this pathology include daytime sleepiness, changes in oxygen saturation in the blood (leading to cognitive impairment) and cardiovascular risks, such as hypertension ▪ Obstructive sleep apnea occurs as the result of a problem with the neural control of breathing o Due to lack of excitatory drive, which is needed to maintain the tone of the respiratory muscles, as well as an anatomical defect (seen in children where the tonsils are relatively large, facial malformations or accumulation of fat around the neck) o No drugs available for treatment Mechanical device delivers a continuous positive airway pressure through a nasal or full facial mask to gently push air into the airways to keep the upper airway passages open Lecture 1 recording 7: Filtering Action and the Muco-ciliary Escalator ∙ Filtering Action: Conducting Zone & Muco-ciliary Escalator: (secondary function) ▪ Regions involved in the filtering action: o Conducting zone and at the level of the alveoli The filtering action in the conducting zone is called the muco-ciliary escalator ❖ Two types of cells line the surface of the trachea: ✔ Goblet cells → sparse; produce mucus; no cilia ✔ Ciliated cells → layer of cells with cilia on the apical surface * ❖ Both cell types function in a coordinated manner to entrap inhaled biological and inert particulates and remove them from the airways ∙ Removal of Deposited Particles in the Tracheobronchial Tree: ▪ Effective clearance of deposited particles in the tracheobronchial tree requires both ciliary activity and respiratory tract fluids ▪ Ciliated cells: o Cilia are able to continuously move o Ciliary movement has preferential direction o Produce periciliary fluid that has a very low density (quite liquid) The fluid sits on the top of the ciliated cells and is called the SOL layer The low density of the fluid allows the cilia to move freely in this fluid ∙ Removal of Deposited Particles in the Tracheobronchial Tree: ▪ Goblet cells: o Produce a very dense, thick mucus called the gel layer Distributed in patches o Trap particulates that enter the respiratory system during inhalation o How do we eliminate this mucus rich in particulates? Eliminate it through the cilia movements; the cilia move in the SOL layer and the tip of the cilia touches the mucus and pushes it continuously in one direction ❖ Cilia movement is downward in the nasopharynx (above the glottis) and upward at the level of the trachea to eliminate mucus through the esophagus because we want to eliminate from the deeper respiratory tract through the esophagus) ▪ Changes in the composition or in the thickness of the sol layer and the gel layer will affect the muco-ciliary escalator o Smoking reduces the activity of the cilia and increases the number of mucus producing goblet cells ∙ Filtering Action: Macrophages in Alveoli: ▪ Macrophages in the alveoli (sparse): o Last defence for eliminating particulates o Smallest particulates in the alveoli attract the macrophages which phagocytose these particulates, digesting them and eliminating the risk of infection o Silica dust or asbestos: Very fine particulates (sharp crystals) Macrophages recognize silica dust or particulates as a foreign object and phagocytose them, but they cannot digest these Silica dust and asbestos then break/ kill the macrophages which then disintegrate, releasing chemotactic factors and promoting recruitment of fibroblasts into the alveoli ❖ This increases the introduction of collagen, a protein that gives stiffness to the lungs ❖ Inhaling silica dust and asbestos results in the development of pulmonary fibrosis ❖ Lungs that are not able to expand lose their elastic properties Lecture 2 recording 8: Lung Volumes and Capacities ∙ Spirometry: ▪ Spirometry → a pulmonary function test that determines the amount and the rate of inspired and expired air o Pressure transducer measures pressure and detects or quantifies the amount of air that is inspired and expired at each breath ∙ Lung Volumes and Capacities: (These definitions are found on the next slide. Make sure you know the different volumes and capacities) ▪ Tidal volume → the volume of air moved IN OR OUT of the respiratory tract (Breathed) during each ventilatory cycle (breathe in and breathe out) ▪ Expiratory reserve volume → the additional volume of air that can be forcibly exhaled following a normal expiration; it can be accessed simply by expiring maximally to the Maximum Voluntary Expiration (breathe out as much as they can) ▪ Inspiratory reserve volume → the additional volume of air that can be forcibly inhaled following a normal inspiration; it can be accessed simply by inspiring maximally, to the Maximum Possible Inspiration (breathe in as much possible) ▪ Residual volume → the volume of air remaining in the lungs after a Maximal Expiration; it cannot be expired no matter how vigorous or long the effort; RV cannot be measured with a spirometry test; RV = FRC - ERV o Always a small volume of air remaining in the lungs – prevents collapsing of the alveoli or atelectasis ∙ Lung Volumes and Capacities: (This slide defines the different volumes, which we looked at in the previous slide) ∙ Lung Volumes and Capacities (4 slides): (Notes for the next 4 slides looking at capacities are found in this one section) ▪ Capacities: o Measurements of lung volume o Correspond to the sum of 2 or more lung volumes o Vital capacity (VC) → the maximal volume of air that can be forcibly exhaled after a Maximal Inspiration; VC = TV + IRV + ERV o Inspiratory capacity (IC) → the maximal volume of air that can be forcibly inhaled; IC = TV + IRV o Functional Residual Capacity (FRC) → the volume of air remaining in the lungs at the end of a normal expiration; FRC = RV + ERV o Total Lung Capacity (TLC) → the volume of air in the lungs at the end of a Maximal Inspiration; TLC = FRC + TV + IRV = VC + RV ∙ Lung Volumes and Capacities: ▪ We cannot measure residual volume with the spirometry test and as a result we cannot measure all the capacities that have residual volume as a component of the capacity itself o Cannot measure (with the spirometry test) the functional residual capacity or the total lung capacity Lecture 2 recording 9: Lung Volumes and Capacities ∙ Lung Volume and Flow: (For the numbers on this slide, know that the TV = 500 mL. You do not need to memorize the rest of the numbers in the calculations, but know how to calculate tidal/minute ventilation or alveolar ventilation, and that alveolar ventilation is less once calculated ie. know that total/minute ventilation = tidal volume x respiratory frequency) ▪ Tidal volume: o The volume of air moved IN OR OUT of the respiratory tract (Breathed) during each ventilator cycle (at rest) o 500 mL ▪ Part of the volume that enters the lungs, or enters the respiratory system, does not reach the alveoli but remains in the conductive zone (has no alveoli and there is no gas exchange) ▪ Total or minute ventilation → the amount of air that is exchanged within a rate time, or within a minute o Total/minute ventilation = tidal volume x respiratory frequency = 0.5 L x 15/min = 7.5 L/min o Not all of this volume of air is available for gas exchange; we must take into consideration the anatomical dead space ▪ Alveolar ventilation: o (0.5 - 0.15) L x 15/min = 5.25 L/min o Subtract the anatomical dead space of 150 mL (0.15 L) from the tidal volume of 0.5 L and multiply by the respiratory frequency of 15 per minute o The alveolar ventilation of 5.25 L/min is smaller than the minute ventilation of 7.5 L/min because the anatomical dead space, the conducting zone, must be taken into consideration ∙ Ventilation: ▪ Tidal volume → the volume of inspired air at each breath, and is equal to approximately 0.5 litres ▪ Total or minute ventilation → the total amount of air moved into the respiratory system per minute o Calculated from the tidal volume multiplied by the respiratory frequency ▪ Alveolar ventilation → the amount of air moved into the alveoli per minute o Less than the minute ventilation as it depends on the anatomical dead space o Calculated by subtracting the anatomical dead space volume from the tidal volume, and multiplying by the respiratory frequency ∙ Alveolar Ventilation (VA): ▪ Tidal volume, or the air that comes into the respiratory system, is 500 mL or 0.5 L ▪ Conducting airways have a volume of about 150 mL (volume of air that is left over from previous breath; no gas exchange in this region) o About 1/3 of a normal breath is not available for gas exchange ▪ Exchange of gases with the blood occurs only in the alveoli (not in the 150 mL of the airways - volume of conducting airways left over in the previous breath) ▪ At the end of expiration the lungs still have a specific amount of air (b/w 1-2L). When air goes in during an inspiratory effort, some of this air will enter the respiratory zone and will be available for gas exchange. o This air is indicated as 350 mL in volume; it enters the respiratory zone and is available for gas exchange; a portion of this fresh atmosphere air from this inspiration will stay in the conductive airways (150mL) and will not be available for gas exchange. ▪ When expiration occurs, there is elimination of the 350 mL of air that was present in the lungs and it was available for gas exchange, so it will be low in oxygen content and high in carbon dioxide levels when it is exhaled. ▪ In contrast, the first part of the exhaled air, the 150 mL, will still be similar to the atmospheric air because it did not go through the gas exchange ▪ Alveoli ventilation (VA) is given by the difference of the tidal volume and the anatomical dead space multiplied by the frequency o VA = (VT – VD) x frequency o If you take either a small breath or a big breath, the conductive airways have the same volume The volume of the anatomic dead space is constant, independent from the volume inhaled at each respiration ∙ Effect of Breathing Pattern on Alveolar Ventilation: (You will not be asked to reproduce the numbers but understand why increased depth of breathing is more effective at increasing alveolar ventilation than an increase in rate of breathing) ▪ How do we improve alveoli ventilation? o Divers inhale slowly and very deeply; this improves ventilation of the lung ▪ Shallow and fast breathing: no air available for alveoli ventilation → all the air breathed in stays in the conducting zone because the tidal volume of 150 mL is very small, no air available for alveolar ventilation) ▪ Deep and slow breathing: Due to the fact that you are breathing in so much air during a single event, this will increase the alveoli ventilation; the majority of the minute ventilation is dedicated to or available for gas exchange ▪ Deep breathing is more effective at increasing alveolar ventilation than is fast, shallow breathing Lecture 2 recording 10: Spirometry Tests to Determine Obstructive/Restrictive Diseases ∙ Spirometry Test – FEV1: ▪ Spirometry is used to determine the forced expiratory volume in 1 second and the forced vital capacity o A patient is asked to make a maximal inspiration and then make an expiratory effort to exhale as much as they can and as fast as they can Two volumes can be determined: ❖ Forced expiratory volume in 1 second (FEV-1) → forced expiratory volume is how much of the vital capacity volume that can be expelled in 1 second ❖ Forced vital capacity (FVC) → forced vital capacity is much the same as the vital capacity described earlier in the regular spirometry test ∙ Spirometry Test: ▪ Forced vital capacity (FVC) is about 5 litres in a healthy person o A normal healthy person is able to expel in 1 second almost all of this volume ▪ 3 factors can be looked at in this test: o Forced expiratory volume in 1 second (FEV-1): a healthy patient can normally expel most of the air from the lungs within 1 second o Forced vital capacity (FVC): the total amount of air that is blown out in one breath after a max inspiration as fast as possible o Ratio between the FEV-1 and FVC The ratio of FEV-1/FVC represents the proportion of the amount of air that is blown out in 1 second ∙ A Spirometry Test Shows One of Three Main Patterns: ▪ A spirometry test is useful to diagnose a patient for either an obstructive disease or a restrictive disease ∙ Obstructive Pattern: ▪ Obstructive lung disease: o Patients affected by obstructive lung disease have shortness of breath due to difficulty in exhaling all the air from their lungs o Problem is with expiration, or with the ability to blow out the air from the lung. o Because of damage to the lungs or narrowing of the airways inside the lungs, exhaled air comes out more slowly than normal; at the end of a full exhalation, an abnormally high amount of air may still linger in the lungs o Seen in patients affected by bronchial asthma, chronic obstructive pulmonary disease or cystic fibrosis o Spirometry test: FEV-1 is significantly reduced in comparison to a normal calculation Process of expiration is also much slower, indicated by a lower slope of the graph in patients with obstructive pattern FVC, depending on the seriousness of the disease, can be normal or slightly reduced Ratio between FEV-1/FVC is also reduced ( PIP (PTP > 0) in order to maintain the lungs expanded in the thorax ▪ PTP is a static parameter which does not cause airflow, but determines lung volume (VL) ▪ PALV is a dynamic component that determines air flow ∙ Inspiration: ▪ During an inspiratory effort: o CNS sends an excitatory drive to the muscles of inspiration (diaphragm, intercostals and parasternals) → muscles contract and generate an increase in the thoracic volume → intrapleural pressure becomes more subatmospheric or more negative → transpulmonary pressure (which is dependent on the intrapleural pressure) increases → increase in lung volume → decrease the alveoli pressure → alveoli pressure will be smaller than the atmospheric pressure → a difference in pressure generates movement of gas → gas will move from a region of high pressure to a region of low pressure → alveolar pressure is smaller so air will move from the atmosphere into the lungs (Graph is to show visually how the pressures and lung volume changes during inspiration) ∙ Expiration: ▪ Relaxation of the inspiratory muscles → chest wall recoils (goes back to its resting state) → intrapleural pressure moves back to a pre-inspiratory value→ as transpulmonary pressure is equal to the alveolar pressure minus the intrapleural pressure, the transpulmonary pressure will also will be reduced as the intrapleural pressure returns to pre-inspiratory values → as transpulmonary pressure is also linked to lung volume, lungs recoil and a reduce volume, generating compression of the gas molecules inside the alveoli → increase in alveolar pressure so it is greater than the atmospheric pressure → air will move from a region of high pressure to a region of low pressure, flowing out of the lungs to the environment (Graph is to show visually how the pressures and lung volume changes during expiration) Lecture 3 recording 13: Resistance to Air Flow ∙ Airway Resistance: ▪ Flow = ∆P/R ▪ Airway resistance to flow is generally small – a small ∆P produces flow ▪ Forces that can affect resistance to air flow: o Inertia of the respiratory system (minimal) o Friction forces 1. Friction between the different alveolar sacs 2. Friction between the lung and the chest wall (intrapleural fluid significantly reduces this friction) 3. Majority of the resistance that is generated is caused by the resistance that the airflow incurs when it enters the airway ❖ 80% of total airway resistance ∙ Airflow Patterns Influence the Resistance to Airflow: ▪ Airflow resistance is even more sensitive to changes in radius when flow is NOT laminar ▪ Laminar airflow: o The subject invests relatively little energy in airflow RESISTANCE; characteristic to the small airways that are distal to terminal bronchioles (in small airways like the terminal bronchioles we have laminar airflow where gas particles move in a linear fashion) Laminar flow (top) -linear fashion Turbulent flow (bottom) -not smooth ∙ Airflow Patterns Influence the Resistance to Airflow: ▪ Transitional airflow: o It takes extra energy to produce vortices → the resistance increases; airflow is transitional throughout most of the bronchial tree, at the ramifications or branches of the bronchial tree ∙ Airflow Patterns Influence the Resistance to Airflow: ▪ Turbulent flow: o Found in larger airways, such as the trachea, the larynx and the pharynx o Turbulent = no longer smooth and laminar o These airways are larger and the velocity of the gas molecule is higher resulting in turbulent flow ∙ Airflow Patterns Influence the Resistance to Airflow: ▪ Turbulent airflow: o Turbulent flow can be found in larger airways, such as the trachea, the larynx and the pharynx Airways are larger and the velocity of the gas molecule is higher leading to turbulent flow ∙ Resistance to Airflow in the Respiratory Tract: ▪ Small airways have the highest resistance to the airflow and have laminar flow ▪ Resistance is determined according to Poiseuille’s law ▪ For laminar flow Poiseuille’s law: R = 8ηl/πr4 o Based on this formula, if the radius of the airway is reduced, resistance will increase ▪ Resistance to airflow is highly sensitive to changes in airway radius ∙ Airway Resistance in the Respiratory Tract: ▪ Minimal resistance in the first part of the conducting zone where there are medium sized bronchi ▪ Resistance falls along the curve and is minimal in the respiratory zone and is lowest in the small airways ∙ Airway Resistance in the Respiratory Tract: ▪ In very small airways resistance should be high: o Each small airway has a high individual resistance (By Pousielle’s law resistance is inversely proportional to the radius raised to the fourth power) o However, the numerous terminal bronchioles have a much lower resistance compared to the few large airways As we go down in generations, or branching, we have an increased number of airways at the same generation These numerous airways will not be disposed or arranged in series but will be arranged in parallel ❖ For airways arranged in series, the resistance is simply the sum of the individual resistances ❖ For airways arranged in parallel, the resistance at each specific generation, when there is respiratory airflow, will be given by the inverse of each specific resistance; as a consequence, the respiratory resistance, at the level of the small airways at the level of the respiratory zone, is minimal ∙ Airway Resistance in Disease Conditions: ▪ Respiratory resistance is minimal in the smaller airways, but the small airways are also the most important in pathological conditions because their overall resistance can change significantly in pathological conditions o Small airways can easily be occluded: As they are surrounded by smooth muscle, contraction of the smooth muscle will increase resistance Edema may alter resistance as the presence of fluid in the small airways can reduce the space available for airflow to move in or for the air to flow into the alveolar sacs Mucus accumulation can reduce the alveolar space at the level of the bronchioles Lecture 3 recording 14: Lung Compliance ∙ Lung Compliance: ▪ Lung compliance can be determined both as a dynamic property or a static property because it can be measured in the presence of airflow or in the absence of airflow ▪ Lung compliance → a measure of the elastic properties of the lungs and a measure of how easily the lungs can expand ▪ Lung compliance is given by the magnitude of the change in lung volume produced by a given change in transpulmonary pressure ▪ Determined from a plot of transpulmonary pressure (x-axis) and lung volume (y-axis) o Compliance is the slope of the curve ∙ Static Compliance of the Lung: ▪ Static compliance: o Represents the lung compliance, or the elastic properties of the lungs, when no air is flowing through o To measure static lung compliance in clinical practice, a patient is asked to make a maximal inspiration and then release a small amount of air, at which time lung volume and transpulmonary pressure are measured This continues in intervals or steps, where the patient exhales another small amount or air and then stops, and the measurements are made again The curves illustrate the ability of the lungs to expand and how much effort, or work is put into inhaling or increasing lung volume at each respiratory breath ▪ Pulmonary fibrosis: o Lung compliance is low o Overproduction of collagen makes the lungs stiff which means that the patient has to make a big effort to expand the chest wall in order to increase the transpulmonary pressure that is responsible for changing the lung volume o A large change in transpulmonary pressure will result in a small change in lung volume Slope of the curve (dashed line) is reduced in patients with low compliance ▪ Emphysema: o Lung compliance is high o Gradual reduction in lung elastic components (seen in seniors) o A small change in transpulmonary pressure will result a large change in lung volume Slope of the curve (dashed line) is increases in patients with high compliance o Floppy lungs have lost alveolar tissue resulting in less surface respiratory membranes available for gas exchange ∙ Dynamic Compliance of the Lung: ▪ Dynamic compliance of the lung: oMeasured during periods of gas flow, either during inspiration or expiration oTakes into consideration not only the elastic properties of the lungs, or the lung stiffness, but also the airway resistance that the air encounters during inspiration or expiration that will potentially reduce the expansion of the lungs oUsually less or equal to the static compliance because of the effect of airway resistance oFalls when either lung stiffness or airway resistance increases oAt FRC, the slope of the curve will be reduced in the case of dynamic compliance and it will be increased in the case of static compliance; it is not just a case of movement up and down the curve but it is the slope of the curve that is important ∙ Pressure-volume Relationship & Hysteresis (2 slides): ▪ Curve for deflation is different from the curve for inflation ▪ In a collapsed lung: o Medium and small airways will be collapsed and there will be little gas exchange o The lung volume will be very low o As you try to inflate the lung at the beginning and transpulmonary pressure progressively increases, the lung volume will not change much, simply because all these airways are collapsed Flat section of the inflation curve (number 1) o Once all the airways are popped open, there will be an exponential increase in lung volume (number 2) All the airways are open and air flows into the alveolar sacs, and this will progressively increase the transpulmonary pressure o There will then be a medium increase in lung volume due to the fact that the alveoli will expand until basically the elastic properties of the lungs do not allow for further expansion (number 3) o The curve will then reach a plateau (number 4) at the limit of airway inflation ∙ Hysteresis: ▪ Lung volume versus the transpulmonary pressure is measured during an inspiration and during an expiration ▪ Hysteresis → defines the difference between the inflation and the deflation compliance path o This has to do with the elastic properties of the lung o Hysteresis defines the different behaviour of the inflation and the deflation curves in pathological conditions as well as in physiological conditions o It exists because more pressure is needed to open an airway rather than keep open an airway that is already open Lecture 3 recording 15: Lung Compliance and Elastic Components ∙ Lung Compliance: ▪ Lung compliance is determined by: o Elastic components of lungs that are part of the anatomic structures of the lungs and the airway tissue (elastin and collagen) o Surface tension at the air-water interface within the alveoli ∙ Elastic Component of the Airways: ▪ Elastin and collagen are important in the lungs: o Localized in alveolar walls, around blood vessels and bronchi o These molecules can influence how elastic or how stiff the lung is o Dynamic molecules - the amount of these proteins varies considerably with different changes in life ▪ Geometric arrangement of these molecules affects lung compliance Elastin is able to give the lung high extensible properties because of its spring shape and improve the ability of the lungs to stretch Collagen gives properties of more stiffness ❖ An increase in collagen causes fibrosis in the lung, resulting in low compliance, as well as the necessity of generating high transpulmonary pressures in order to inflate the lung at low volumes There is a general degeneration or reduction in the amount of both elastin and collagen in the lungs with aging, and this is associated with an increase in lung compliance and floppy lungs ∙ Emphysema: ▪ Elastin and collagen can influence lung compliance ▪ Healthy lung tissue: o Well organized with very regularly shaped alveoli and a dense network of alveoli within the lung tissue ▪ Emphysema: o A few alveoli are similarly shaped to a healthy lung but there are many large alveolar spaces and therefore a reduction in the surface that is available for gas exchange o Lungs will be floppy because the majority of elastin is eliminated, damaging the architecture of the alveoli o Increased compliance in which little changes in pressure will generate large changes in volume o With many large holes, the amount of surface that is available for gas exchange is extremely reduced ∙ Pulmonary Fibrosis: ▪ Pulmonary fibrosis: o Associated with silica dust inhalation and the accumulation of collagen in the lungs o Lungs are extremely stiff and there is a reduction in lung compliance o A very big effort must be made to inhale in order to increase lung volume o Deposition of collagen may damage the alveolar structure ▪ The respiratory membrane is thicker making gas diffusion extremely difficult → strong reduction in lung compliance (stiffness) Lecture 3 recording 16: Lung Compliance and Surface Tension ∙ Lung Compliance: ▪ Lung compliance is determined by elastic components and surface tension o Surface tension → property or phenomenon that occurs at the level of the interface between the surface and the air Makes the lungs collapse or gives the lungs elastic recoil Surface tension decreases lung compliance ∙ Alveolar Surface Tension: ▪ Surface tension is a property of the molecules of the fluid that are in contact with the air/fluid interface. o Molecules in the bulk of the fluid will tend to interact with each other, especially if they have polarity o A water molecule that is deeply embedded within the bulk of the liquid will interact with the other molecules in all directions, creating strong bonds o Water molecules at the surface do not have the same molecules on all sides they are in contact with other water molecules and also with gas molecules oWater molecules at the surface will have very little interaction with the gas, but will create strong bonds with the water molecules that are close to the surface This creates a very strong attractive force at the level of the air interface between the molecules of water ▪ Surface tension → a measure of the attracting forces acting to pull a liquid’s surface molecules together at an air-liquid interface ∙ Surface Tension: (This slide shows examples of when we see surface t ension…you do not need to know these examples) ∙ Factors Affecting Pressure-volume Relation: ▪ What is the role of surface tension in the lungs? o Type I alveolar cells line the surface of the alveolar walls They are in contact with fluid that contains surfactant The internal surface of the alveoli is lined with a thin layer of fluid, and air continuously comes in and out of the alveoli o Surface tension is seen at all air-fluid boundaries and arises as a result of hydrogen bonding of water molecules o Importance of surface tension is clear in P-V curve in which surface tension is eliminated with saline-filled lung If you fill an isolated deflated lung with liquid or saline so there is no air in the lung, the pathway of lung compliance is much steeper; this has to do with the fact that the surface tension is not involved in the process o The effect of surface tension is to “cause” the surface to maintain as small an area as possible o No hysteresis and much lower inflation pressures o The surface tension is what influences the movement towards the right of the lung compliance curve ∙ Alveolar Surface Tension: ▪ Air entering the lungs is humidified and saturated with water vapour at body temperature ▪ Water molecules cover the alveolar surface ▪ Surface water molecules create substantial surface tension o The water molecules that are aligned on the surface will have a stronger attractive force that operates in between the molecules of water and create substantial surface tension ∙ Alveolar Surface Tension: ▪ The molecules of water create inward recoil and influence the collapse of the alveoli ▪ The surface tension itself acts like a belt in order to reduce the surface of our ideal sphere o The effect of surface tension will be to reduce the volume of the ideal sphere, or the alveoli ∙ Alveolar Surface Tension: ▪ At any given time, a given pressure must be exerted in order to keep the alveoli open o At equilibrium, the pressure that is necessary for keeping the alveoli open counterbalances the tendency for the alveoli to collapse that is provided or given by the surface tension ▪ Laplace’s equation describes this equilibrium o P = 2T/r (T =surface tension, r = radius) ▪ The smaller the bubble’s radius, the greater the pressure needed to keep the bubble inflated (different sizes of alveolies will require different pressure to counterbalance the tendency to collapse) ∙ Alveolar Surface Tension: ▪ Sizes of alveoli in our lungs are not identical ▪ Alveoli are not isolated but are all communicating with each other ▪ Assume that the surface tension, or T, in the different sized alveoli is similar o According to Laplace’s equation, the pressure that is necessary to keep open large alveoli is smaller than the pressure that is necessary to keep open the smaller alveoli o This means that the pressures in the two alveoli are not equal, and there is a region of higher pressure in the smaller alveoli and a region of lower pressure in the larger alveoli What is the result of this pressure difference? ❖ As a result of surface tension, the gas that is present in the smaller alveoli will move to the large alveoli, moving from a region of higher pressure to a region of lower pressure ❖ The smaller alveoli will collapse and we will no longer have a multi-faceted, multi-alveolar lung (now only have a bubble of air) ❖ This does not occur physiologically due to the presence of surfactant Lecture 4 recording 17: Surface Tension and the Role of Surfactant ∙ Lung Compliance: ▪ Alveolar Surfactant: o Produced by Type II alveolar cells o Functions to reduce the surface tension at the level of the alveoli o The overall effect is improved lung compliance allowing a reduction in the work of breathing o Makes the alveoli stable against collapse o Allows alveolar communication between alveoli of different sizes without collapsing ∙ Surfactant and Surface Tension: ▪ Surfactant is a mixture of phospholipids (do not need to memorize exact composition of surfactant) o Characterized by having a hydrophilic head and a hydrophobic tail Hydrophobic tail will sit in the alveolar air and the hydrophilic head will dip into the water ❖ At the air/water interface o The overall effect of positioning the surfactant at the interface is that it breaks the strong attractive forces that occur between the molecules of water at the surface o Surfactant reduces the surface tension of the water, increases lung compliance and makes it easier to breathe ∙ Alveolar Stabilization by Surfactant: ▪ Assume that there is a nearly constant amount/concentration of surfactant in alveoli of different sizes - Surfactant layer continues to change in size due to inspiration and expiration ▪ Surfactant, which is always present on the surface of the air/water interface in the alveoli, will be closer together or further apart according to the different phase of the respiratory cycle o The equal amount/concentration of surfactant in alveoli of different sizes will be differently organized depending on the size of the alveoli In smaller alveoli, surfactant is found closer together In larger alveoli, surfactant is further apart or more sparse Surface tension, or T from LaPlace’s equation, is therefore increased in the large alveoli and reduced in the small alveoli When surfactant is more concentrated on the surface, you will have lower surface tension, as is seen in the smaller alveoli ▪ A more dense, or more concentrated, surfactant in the smaller alveoli, will equalize the pressure that is necessary to keep the smaller alveoli open o No pressure gradient will exist between the alveoli of different sizes and air will not flow from the small alveoli to the big alveoli and, as a consequence, the small alveoli will not collapse into the large alveoli (equalization) ▪ The dynamic properties of surfactant permit the alveolar tension to change with inflation and deflation and the thickness of the surfactant layer varies inversely with the surface area ∙ Surfactant Improves Compliance and Stabilizes Alveolar Size: ∙ Functions of surfactant: ▪ Reduces surface tension of the alveolar fluid, improves lung compliance ▪ Stabilizes alveoli ∙ Surfactant stabilizes the expansion and maintenance of the alveolar sacs ∙ Premature babies have problems with respiration because surfactant is produced in the last stages of pregnancy ▪ They do not have enough surfactant in their lungs and, as a consequence, have to make a very large inspiratory effort in order to have an increase in lung volume ▪ Infant respiratory distress syndrome o Administer artificial surfactant to the airways in the lung which reduces the surface tension and improves breathing and reduces the work of breathing Lecture 4 recording 18: Regional Differences in Ventilation ∙ Regional Differences in Ventilation Due to Gravity and Posture: ▪ Ventilation is not equal in the different regions of the lungs ▪ Inhalation test with radioactive xenon looks at the regional ventilation of the lung o Test shows that ventilation is higher in the lower zone of the lung (at the bottom of the lung) and is lower in the upper zone ▪ If a patient is lying flat, the highest amount of ventilation, or the highest amount of radioactivity, is measured at the back of the lungs, towards his back, whereas the front of his lungs, towards the chest wall, will have much less lung activity ▪ Regional ventilation changes have to do with gravity and posture ∙ Regional Differences in PIP: ▪ How do we explain the difference in ventilation between different regions of the lungs? o There is a negative pressure in the intrapleural space described as subatmospheric which changes between the top of the lung and the bottom of the lung Intrapleural pressure (PIP) is much more negative at the top of the lung than at the bottom Intrapleural pressure is generated by the balance between the elastic recoil of the lung and the elastic chest wall The lungs have weight and are affected by gravity; this weight increases pressures, or makes the intrapleural pressure less negative, in regions at the bottom of the lung; pressures at the top of the lung decrease, or become more negative ▪ Curve of lung changes versus intrapleural pressure o At a less negative pressure, there will be a large increase in lung volume o With a larger, more subatmospheric value of intrapleural pressure, the change in lung volume will be reduced ▪ At the beginning of an inspiration, the alveoli that are at the bottom of the lungs start their inspiratory effort being more deflated because the transpulmonary pressure that generates the lung volume is influenced by the values of intrapleural pressure; when inflation occurs, the alveoli at the bottom of the lung are in an advantageous position because little changes in intrapleural pressure will generate large changes in lung volume ▪ Since alveoli at bottom are starting more deflated, they are able to expand more o Bottom regions of lung receive a larger portion of the inspired air (receive more ventilation) Lecture 4 recording 19: Gas Exchange (Make sure you know the different laws we will talk about and what they state) ∙ Gas Exchange: ▪ Gas exchange – gas exchange between the alveoli and the capillary system (2nd step in respiratory process) o Involves the diffusion of gas molecules from the alveoli to the capillary system and the diffusion of gas molecules from the capillary system to the alveoli ∙ Partial Pressure of Gases: ▪ Gas molecules are constantly in motion and this generates pressure ▪ The pressure generated is dependent on: temperature and the concentration of the gas molecules ▪ Dalton’s law calculates the overall pressure that the mixture of gas molecules generates o Dalton’s law states that in a mixture of gas, such as air, each gas has its specific pressure and the total pressure of this mixture of gas is given by the sum of the individual pressures Oxygen, nitrogen, carbon dioxide and water are found in the air we breathe The percentage of water in inhaled air will increase because air, once inhaled, enters the nasal and oral cavities, is warmed and becomes humidified. ❖ The percentage of water in alveolar air will be increased as a result ∙ Partial Pressures of Gases in the Atmosphere at Sea Level: (You will not be asked to re-produce these numbers, the percentages or partial pressures, but we will use them in our lectures as we look at respiration) ▪ At sea level, the atmospheric pressure is around 760 mm Hg ∙ Diffusion: How Gas Gets Across the Blood-gas Barrier: ▪ Gas exchange occurs at the level of the respiratory membrane o Respiratory membrane: a very thin layer of tissue that contains the fluid, epithelial cells in the alveoli, interstitial space, basement membrane of the capillary epithelium and the epithelium o Thinness is advantageous for the diffusion of gases from the alveoli to the capillary system and vice versa (thickness is very minimal) o The process of diffusion of gases through the respiratory membrane follows Fick’s law o Fick’s law: the rate of transfer of a gas (V; L/min) through a sheet of tissue per unit of time is proportional to the surface area of the membrane (A) and depends on the difference in partial pressures between the two environments, or (P1 - P2) and inversely proportional to the thickness (T) of the membrane (D = diffusion constant) Vgasα A D (P1 - P2) T ▪ Changing surface area and thickness will alter rate of transfer of a gas (V) ∙ Solubility of Gases in Liquids: ▪ Diffusion constant (D) o The amount of gas transferred between the alveoli and the blood per unit time is also proportional to the gas solubility in fluids or in tissue Diffusion constant is proportional to the solubility of the gas and inversely proportional to the square root of the molecular weight The solubility of the gas in the fluid will influence the diffusion process ▪ Carbon dioxide is much more soluble than oxygen o Carbon dioxide will have a faster diffusion process compared to oxygen due to its higher solubility ∙ Diffusion of Gases in Liquids: ▪ Henry’s Law: the amount of gas dissolved in a liquid is directly proportional to the partial pressure of gas in which the liquid is in equilibrium ∙ Diffusion of Gases in Liquids: ▪ The concentration of gas molecules in a liquid is determined by its partial pressure and solubility o If we compare two conditions in which PO2 and PCO2 are the same, the concentration of CO2 in the liquid will be much higher than the concentration of O2 in the liquid due to CO2 having a higher solubility than O2 ▪ All of the gas that is dissolved in the solution will contribute to the calculation of partial pressure o Oxygen is soluble in plasma but oxygen bound to hemoglobin will not contribute to the calculation of partial pressure in plasma Lecture 4 recording 20: More on Gas Exchange ∙ Diffusion of Gases in Liquids: ▪ Partial pressure of water is variable and depends on how saturated the air is o When the air enters the alveoli, there is warming up of the air and humidification because the air comes in contact with mucous membranes that are wet A higher percentage of water in alveolar air ▪ At the level of the alveoli o Partial pressure of oxygen is reduced o Partial pressure of carbon dioxide is increased ∙ Partial Pressure of Gases: ▪ Venous blood returns to the heart from the systemic circulation, and then travels by the pulmonary arteries to the lung capillaries o Mixed venous blood containing oxygen and carbon dioxide returns to the lung capillaries available for the gas exchange PO2 in the atmosphere is 160 mmHg; PO2 at the level of the alveoli is 105 mmHg When the mixed venous blood enters the pulmonary circulation, PO2 is 40 mmHg ❖ The diffusion process from the alveoli to the lung capillaries is very fast and oxygen will move from the alveoli to the lung capillaries as soon as air enters the alveoli ❖ Blood exiting the lung is equivalent to the gas that is present at the level of the alveoli ✔ PO2 in blood exiting the pulmonary capillaries is 100 mmHg (similar to 105 mmHg seen in the alveoli) ∙ Partial Pressure of Gases: ▪ Why is the PO2 in the air in the alveoli (105 mmHg) not the same as the PO2 in the atmosphere (160 mmHg)? o Air enters the respiratory system and reaches the alveoli where it is warmed up and humidified; the partial pressure of water will increase and the partial pressure of oxygen will decrease o As soon as the air enters the alveoli, where there is lots of blood available for diffusion or gas exchange; this also decreases the PO2 o Another factor that affects the reduction of PO2 from the atmosphere to the alveoli is the fact that once the air enters the alveolar network, it will mix with the air that constitutes the functional residual capacity - the air that is already there at the level of the lung that did not move out with the previous expiratory process; decrease the partial pressure of oxygen in the alveoli The 350mL of air that enters the lungs during inspiration will immediately mix with the air that is already present in the lungs, because the lungs at the end of an inspiratory process are not completely empty This air that is already present in the lungs sits in the alveoli for several respiratory cycles, and as air moves into the lungs it continuously mixes with the new air ∙ Partial Pressure of Gases in Alveoli: ▪ Determinants of alveolar PO2: o PO2 in the atmosphere At higher altitudes, the percentage of oxygen in that air is still 21 % but as the overall atmospheric pressure is reduced, then the PO2 is reduced proportionally o Alveolar ventilation ❖ Alveolar ventilation indicates how much air is exchanged per unit of time ❖ An increase in alveolar ventilation means that more air will be continuously exchanged at the level of the respiratory system ❖ The air at the level of the alveoli will be more similar to the air at the level of the atmosphere o Metabolic rate Exercising will result in a lower PO2 in the mixed venous blood that arrives at the respiratory membrane; this will promote an increased differential in pressure between the alveoli and the blood system and, as a consequence, PO2 at the level of the alveoli will be decreased o Lung perfusion A change in cardiac output will alter the amount of blood that passes through the respiratory system, influencing the alveolar PO2 ▪ Determinants of alveolar PCO2: o PCO2 in the atmosphere (essentially zero) When the blood is in contact with the respiratory membrane, a large amount of CO2 moves out or diffuses to the alveoli and alveolar PCO2 is much higher than atmospheric PCO2 o Alveolar ventilation A decrease in alveolar ventilation will lead to less exhalation of CO2 from the alveoli to the atmosphere and therefore PCO2 will be increased at the level of the alveoli. o Metabolic rate An increase in metabolic rate will increase carbon dioxide production, and there will be a higher level of PCO2 that diffuses into the alveoli o Lung perfusion ∙ Alveolar Gas Pressures: Effect of VA: ▪ Increasing alveolar ventilation (VA) will increase alveolar PO2 and decrease alveolar PCO2 o Due to increased ventilation, there will be an increase in PO2 because the alveolar air will be more similar to the air that is present in the atmosphere o When the ventilation is increased, more CO2 is eliminated ▪ Reducing alveolar ventilation will reduce the amount of gas exchange between the alveoli and the atmosphere, resulting in an increase in alveolar PCO2 and a decrease in alveolar PO2 ∙ Alveolar Gas Pressures: Effect of Metabolic Rate: ▪ Increasing metabolic rate (increases O2 consumption and CO2 production) will decrease alveolar PO2 and increase PCO2 ▪ A reduction in metabolic rate will decrease alveolar PCO2 and increase PO2 (sleeping) Lecture 5 recording 21: Gas Exchange and the Pulmonary Circulation ∙ Gas Exchange between Alveoli and Blood: ▪ Diffusion of gases is very fast o Blood gasses equilibrate very quickly on their way through the lung o Increase in PO2 occurs within the first third of the capillary length; advantageous in a disease condition as the diffusion process can continue for an extra length of time or length of the capillary compared to a healthy person ▪ Partial pressure of gas in alveoli determines arterial levels ∙ Perfusion of the Lung: (Review of the systemic and pulmonary circuits. You will only need to know what is in the study notes) ▪ Pulmonary circulation is a low pressure system (because blood only moves from the heart to the lungs) o An important reason why we need to have a low pressure system at the level of the pulmonary circulation is because the respiratory membrane is extremely fragile High blood pressure (eg. pulmonary hypertension) can damage the respiratory membrane and edema and an influx of plasma or red blood cells could move into the lungs and affect respiratory function ∙ Pulmonary Circulatory System: ▪ Pulmonary circulatory system: o Low pressure system to prevent rupturing of the respiratory membrane and edema formation - Low pressure because needs to pump blood only to the top of the lung o Low resistance system Resistance defined by Poiseuille’s law ❖ Length of the vessels and the fourth power of the radius affects resistance ❖ Short, wide vessels consistently reduce the resistance of the pulmonary circulation - Resistance is less than 1/10 of that in the systemic circulation o High compliance system Thin-walled vessels Vessels have very little smooth muscle (not much contraction) and small changes in pressure result in a large expansion of the vessel ❖ Vessels will dilate in response to modest increases in arterial pressure - Can load the pulmonary circulatory with large amounts of blood (advantageous) ∙ Pulmonary Capillaries: (You do not need to memorize the volumes, sizes and times in this slide) ▪ At the level of the capillaries or where gas exchange occurs amount of blood can also increase dramatically because of the high compliance vessel ▪ The capillary system creates a very strong network within the alveolar structures and this has the advantage of having a very large respiratory membrane, or a very large surface that is available for gas exchange ▪ Blood passes quickly through the capillaries, but can pass even quicker if there is an increase in cardiac output → more blood per unit of time arrives at the pulmonary system ▪ Alveolar capillaries are collapsible: if the capillary pressure falls below alveolar pressure, the capillaries close off, diverting blood to other pulmonary capillary beds with higher pressures (advantageous) Lecture 5 recording 22: Ventilation-perfusion Relationship ∙ Ventilation-perfusion Relationship: ▪ Inspired air must be delivered to regions of the lung where the blood is going and vice versa ▪ The ventilation/perfusion (V/Q) ratio is the balance between the ventilation (Bringing O2 in to/removing CO2 from the alveoli) and the perfusion (Removing O2 from the alveoli and adding CO2) ▪ The ratio between the ventilation and the perfusion is one of the major factors affecting the alveolar (And therefore arterial) levels of O2 and CO2 ∙ Ventilation-perfusion Relationship: ▪ With increased ventilation, there is a large amount of air per unit of time that enters the alveoli o The greater the ventilation, the more the PO2 and PCO2 of the alveoli will be similar to the atmospheric pressures ▪ Perfusion changes can also affect alveolar PO2 and PCO2 o With a greater perfusion the composition of the alveolar air, at the region where there is a higher perfusion, will be more similar to the mixed venous blood with a reduction in PO2 and an increase in PCO2 ∙ Ventilation-perfusion Relationship: ▪ At normal/optimal ventilation/perfusion ratios: o PO2 ~ 100 to 105 mmHg o PCO2 ~ 40 mmHg ∙ Ventilated Alveoli That Lack Perfusion: ▪ If we look at the ventilation/perfusion (V/Q) ratio, in order for the ratio to be high, there must be an increase in ventilation, V, or a decrease in perfusion, Q ▪ A high ventilation/perfusion ratio: o Seen when there is a collapsing of the lung capillaries, pleurisy or other diseases that affect the vasculatory system o Blood flow is obstructed or occluded o Alveoli are normally ventilated but there is very little gas exchange that occurs across the respiratory membrane because there is no blood available for gas exchange o The PO2 at the level of the alveoli will be increased because there is no oxygen that passes through the lungs to the vasculature (not a strong differential pressure between the alveoli and blood system) o The PCO2 at the level of the alveoli will be decreased because there is no CO2 that is delivered and diffuses from the capillary system to the alveoli o The alveolar space that is in contact with this occlusion of vessels becomes very much similar to the atmospheric air - values for PO2 and PCO2 in the alveoli are the same as the values in atmospheric air o Alveolar dead volume (alveolar physiological dead space) → the region of the lungs where there is a high ventilation/perfusion ratio because of a pathological condition o Region is over-ventilated or under-perfused and does not contribute to gas exchange ▪ Anatomical dead volume → different from the alveolar dead volume; reflects the conductive zone (not properly perfused) ∙ Perfused Alveoli That Are Not Ventilated: ▪ Low V/Q ratio = airway obstruction o Obstruction: collapsed bronchi or bronchioles No gas exchange between the alveolar air or alveolar space and the atmosphere Ventilation to perfusion ratio will decrease The blood will still move through this alveolar space and will be in contact with the alveolar space through the respiratory membrane - Differential pressure between oxygen in the alveoli and the blood system will equilibrate because there is not enough fresh oxygen being delivered from the alveoli to the blood In the alveolar space there will be a decrease in PO2 and an increase in PCO2 The amount of blood that passes through this system will be defined as a shunt → portion of the venous blood that does not get oxygenated, that is not ava ilable for gas exchange because of alveolar occlusion Lecture 5 recording 23: More on the Ventilation-perfusion Relationship ∙ Regional Differences in Lung Perfusion: ▪ There are regional differences in both lung perfusion and lung ventilation ▪ The local ventilation-perfusion ratio (VA/Q) determines the local alveolar PO2 and PCO2 ∙ Regional Differences in Lung Perfusion: ▪ The weight of the lungs increases pressure in regions near bottom (Makes PIP less negative) therefore less pressure pulling it open than regions at top of lung ▪ Since alveoli at bottom are starting more deflated, they are able to expand more; bottom regions of lung receive a larger portion of the inspired air ∙ Regional Differences in Lung Perfusion: ▪ Changes in lung perfusion can be measured by injecting a patient with radioactive xenon o Patient holds breath as soon as the radioactive material is injected to measure radioactivity in the different regions of the lung o Perfusion is higher at the level of the base of the lung o This is associated with gravity and posture o Changing position will affect not only lung ventilation but also lung perfusion ∙ Ventilation-perfusion Relationship is Not Uniform through a Healthy Lung: ▪ Lung ventilation and lung perfusion are higher at the bottom or base of the lung compared to the top of the lung ▪ Both blood flow (perfusion) and ventilation decrease from the bottom of the lungs to the top of the lungs o The slope of the two lines representing perfusion and ventilation is different o This leads to the ventilation/perfusion ratio being lower at the bottom of the lungs and higher at the top of the lungs ▪ If we have an ideal ventilation/perfusion ratio at the base of the lung we have 0.6 times of the ideal ventilation/perfusion ratio o There will be a slightly reduced PO2 level and slightly increased PCO2 level at the bottom of the lung compared to ideal values ▪ At the top (apex) of the lung, there is 3 times the ideal ventilation/perfusion ratio o At the level of the top of the lung, alveolar PO2 will be increased and alveolar PCO2 will be decreased compared to the ideal values ∙ Ventilation-perfusion Matching: ▪ The respiratory system can maintain appropriate ventilation and perfusion in different regions of the lung o Homeostatic mechanism that tends to eliminate the continuous mismatch between ventilation and perfusion ∙ Ventilation-perfusion Matching: ▪ Bronchoconstriction in an alveoli: o Makes diameter of the airway smaller leading to a reduction in local ventilation of that area o This will increase alveolar PCO2 and decrease alveolar PO2 o Decreasing alveolar PO2 will reduce the diffusion process of O2 from the alveoli to the capillary system This reduction in PO2 will have a local effect to cause vasoconstriction of the arterioles Physiological response of reduced ventilation is a reduced perfusion locally Blood will be diverted to the regions where ventilation is still effective ∙ Matching of Ventilation and Perfusion in Alveoli: ∙ Homeostatic responses within the lungs in response to both decreased air flow and decreased blood flow. ∙ Decreased airflow to a region of the lung → decreased alveolar pressure and decreased PO2 → decreased partial pressure for oxygen at the level of the alveoli will influence the pulmonary blood PO2→ decrease PO2at the level of the arterial blood → vasoconstriction of the pulmonary vessels → vasoconstriction of the pulmonary vessels will decrease blood flow → local adaptive effect to this decreased airflow to a region of the lungs is to decrease blood flow → blood will be diverted to regions where ventilation is improved or is still effective ∙ Decreased blood flow to region of the lung → increase alveolar PO2and decrease alveolar PCO2 → bronchoconstriction → less air moves into the region that has decreased blood flow → diversion of blood flow from a region that has low ventilation to a region that has high ventilation to match a local decrease in perfusion ∙ Continuous crosstalk between ventilation and perfusion to maintain optimal levels of gas exchange Lecture 5 recording 24: Transport of Oxygen in the Blood ∙ Oxygen Transport in the Blood: ▪ Oxygen: o Carried in blood in 2 forms: Dissolved in plasma (2 %) of oxygen is found dissolved in the plasma Combined with hemoglobin (98%) o Low solubility: Only a portion of oxygen will be dissolved in plasma At the level of the pulmonary capillaries oxygen diffuses from the alveoli into red blood cells