CAIE IGCSE Physics PDF
Document Details
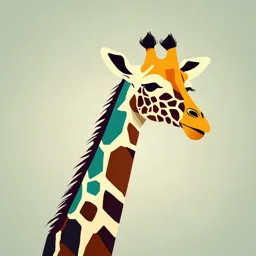
Uploaded by PicturesqueNeumann
Scholastica
CAIE
Tags
Summary
This document is a set of physics notes covering topics such as motion, forces, energy, and thermal physics. It describes concepts like physical quantities, scalar vs. vector, pressure, kinetic energy, and energy transfers. This summary of the first part of the document provides a basic overview of the key physics concepts.
Full Transcript
# CAIE IGCSE PHYSICS ## 1. Motion, Forces and Energy ### 1.1. Physical Quantities and Measurement Techniques #### Units and Basic Quantities - A standard or unit must be chosen before a measurement can be made. - The size of the quantity to be measured is found using an instrument with a scale m...
# CAIE IGCSE PHYSICS ## 1. Motion, Forces and Energy ### 1.1. Physical Quantities and Measurement Techniques #### Units and Basic Quantities - A standard or unit must be chosen before a measurement can be made. - The size of the quantity to be measured is found using an instrument with a scale marked in the unit. - Three basic quantities are measured in physics: length, mass, and time. - Units for other quantities are based on length, mass, and time. - The SI (Système International d'Unités) system is a set of units used in many countries. #### Length - Unit of length: metre (m) - 1 decimetre (dm) = 10-¹ m - 1 centimetre (cm) = 10-² m - 1 millimetre (mm) = 10-³ m - 1 micrometre (µm) = 10-⁶ m - 1 nanometre (nm) = 10-⁹ m - Multiples for large distances: - 1 kilometre (km) = 10³ m - 1 gigametre (Gm) = 10⁹ m - Many length measurements are made with rulers/meter rule. - For any length less than a meter, we use a tape measure. #### Area - The area of a square with sides 1 cm long is 1 square centimetre (1 cm²). - Area formula: area = length × breadth. - SI unit of area: square metre (m²), which is the area of a square with sides 1 m long. #### Volume - Volume is the amount of space occupied. - Unit of volume: cubic metre (m³). - Commonly used unit for volume: cubic centimetre (cm³). - Volume of a cylinder: $V = πr²h$. - A measuring cylinder can measure the volume of a liquid. Ensure the cylinder is upright and the eye is at bottom level of the meniscus. #### Time - Unit of time: second (s). - Time-measuring devices use oscillations. - Choose a timer that is precise enough for the task (e.g., a stopwatch for the pendulum period or a millisecond timer for measuring the speed of sound). #### Scalars and Vectors - Scalar quantity: has magnitude (size) only. - Examples: distance, speed, time, mass, pressure, energy, temperature. - Vector quantity: described by both magnitude and direction. - Examples: force, gravitational field strength, electric field strength, weight, velocity, acceleration, momentum. - Representing Vectors: a straight line with length indicating the magnitude and an arrow showing direction. - Adding scalars: ordinary arithmetic. - Adding vectors: geometrically, considering both magnitude and direction. - For two vectors, FX and FY, at right angles: - The magnitude of the resultant $F = √(FX² + FY²)$. - Angle θ between FX and F $tan θ = FY/FX$. ## 1.2. Motion ### Distance and Displacement - Distance is a length a body travels between two points. It is a scalar quantity. - Displacement is similar to distance but as it is a vector quantity, direction is also considered. ### Distance-Time Graph Examples - At rest. - Constant speed. - Non-constant speed. ### Speed - Speed is the distance travelled by a body in unit time. - When the distance travelled is (s) over a short time period (t), the speed (v) is given by: $υ = s/t$. - Key definition: Speed is the distance travelled per unit time. - General formula: $υ = s/t$. ## 1.3. Mass and Weight ### Mass - The mass of an object is the measure of the amount of matter in it. It is a measure of the quantity of matter in an object at rest relative to an observer. - The standard unit of mass is the kilogram (kg), with the gram (g) being one-thousandth of a kilogram: 1g = 10-³kg = 0.001kg. - Mass is different from weight, which is a gravitational force on an object with mass. ### Weight - Weight is the gravitational force acting on an object that has mass. - The weight of an object can vary with location due to differences in gravitational field strength. - The unit of force is the Newton (N). Weight is measured in newtons and can be determined using a spring balance. ## 1.4. Density - Density (ρ) is the measure of mass per unit volume $ρ = m/V$. - Standard units for density include kilograms per cubic meter (kg/m³) or grams per cubic centimeter (g/cm³). ## 1.5. Forces ### Force - A force is a push or a pull that can change the motion, speed, or shape of an object. - It can cause objects at rest to move or alter the direction of moving objects. ### Extension in Springs - Springs follow Hooke's Law, where extension is proportional to the stretching force up to the limit of proportionality. - Symbolically, extension × stretching force. ### Spring Constant - The spring constant (k) measures the force needed to cause a unit extension in a spring. - $k = F/x$, where (F) is the force applied and (x) is the resulting extension. ### Load-Extension Graphs - Used to graphically represent the relationship between applied force (load) and resulting extension in materials like springs. - Non-linear graphs beyond the limit of proportionality indicate permanent deformation. ### Forces and Resultants - Forces have magnitude and direction, represented by arrows in diagrams. - Multiple forces acting on an object can be balanced (e.g., weight and support force) or have a resultant force. - The resultant force is the single force that has the same effect as all forces acting together. - A resultant force can change the velocity of an object by altering its speed or direction of motion. ### Newton's First Law - An object remains at rest or continues to move at a constant speed in a straight line unless acted upon by a resultant force. - This means that no force is required to maintain constant velocity if no external forces act on the object. ### Friction and Air Resistance - Forces like friction and air resistance cause objects to slow down and eventually come to rest. - In their absence, objects would continue moving indefinitely with constant speed. ### Newton's Second Law - States that the acceleration of an object is directly proportional to the force acting on it and inversely proportional to its mass. - Mathematically expressed as: $F = ma$, where (F) is the resultant force in newtons (N), (m) is the mass in kilograms (kg), and (a) is the acceleration in meters per second squared (m/s²). ## 1.6. Energy, Work and Power ### Types of energy stores - Chemical Energy: Energy stored in chemical bonds of substances like food, fuels (oil, gas, coal, wood). - Gravitational Potential Energy: Energy an object possesses due to its position relative to a reference point (usually the Earth's surface). - Elastic Strain Energy: Energy stored in an object when it is compressed, stretched, or deformed. - Kinetic Energy: Energy possessed by a moving object. - Electrostatic Energy: Energy stored in charged objects due to their separation in an electric field. - Nuclear Energy: Energy stored in the nucleus of an atom. - Internal (Thermal) Energy: Total energy stored in the microscopic motions and interactions of particles within a substance. ### Energy Transfers - Mechanical Working: Transfer of energy by the action of a force, like lifting a weight. - Electrical Working: Transfer of energy by an electric current, such as in batteries or electric motors. - Waves (Electromagnetic and Sound): Transfer of energy through waves, like light or sound waves. - Heating: Transfer of energy through thermal processes, like heating water in a boiler. ### Principle of Conservation of Energy - Energy cannot be created or destroyed, only transformed from one form to another. Thus the total amount of energy is constant. ### Energy Forms - Kinetic Energy (Ek): Energy possessed by an object due to its motion. - $Ek = ½mv²$, where (m) is mass and (v) is velocity. - Potential Energy (Ep):Energy an object has due to its position or condition or configuration. - $Ep = mgh$, where (m) is mass, (g) is acceleration due to gravity, and (h) is height. ### Work - Work (W) is done when a force (F) displaces a body through a distance (d) in the direction of the force. - Formula: $W = F ⋅ d$. - Unit: The unit of work is the joule (J), where 1 J=1N ⋅m. ### Power - The power of a device is the work it does per second, or the rate at which it does work. - Power also represents the rate at which energy is transferred from one store to another. - Formula: - $power = work done/time taken$. - $P = W/t$ where W is the work done in time t. - $P = ΔE/t$ where ΔE is the energy transferred in time t - Key definition: Power is the work done per unit time and the energy transferred per unit time. - Unit of power: watt (W), where 1 W = 1 J/s. ### Efficiency - % Efficiency formula for energy $Efficiency (%) = (Useful Energy Output/Total Energy Input) × 100%$ - % Efficiency formula for power $Efficiency (%) = (Useful Power Output/Total Power Input) × 100%$ ### Sankey Diagrams - Sankey diagrams are used to represent energy transfers and efficiencies visually. - They show how input energy is divided into useful output energy and wasted energy. - The width of the arrows in a Sankey diagram is proportional to the amount of energy they represent. - A wide arrow represents a large amount of energy, while a narrow arrow represents a small amount. ## 1.7. Pressure - Pressure is the force per unit area. - Formula: $pressure = force/area$. - Key definition: Pressure is the force per unit area. - Unit of pressure: pascal (Pa), where 1 Pa = 1 N/m² - Greater area over which a force acts results in less pressure. ### Liquid Pressure - Pressure in a liquid increases with depth because the further down you go, the greater the weight of liquid above. - Pressure at one depth acts equally in all directions. - Pressure depends on the density of the liquid; the denser the liquid, the greater the pressure at any given depth. - The change in pressure Δp at a depth Δh below the surface of a liquid with density p is determined by considering a horizontal area A. - Force acting vertically downwards on area A equals the weight of the liquid column of height Δh and cross-sectional area A above it. - Volume of the liquid column: ΔhA. - Mass of the liquid column: m = (density × volume) = pΔhA (mass). - Weight of the liquid column: mg = pΔhAg. - Force on area A: pΔhAg. - Pressure due to the liquid column: $pressure = force/area = pΔhAg/A = pgh.$ - Formula: $Δp = pgh.$ - Δp is the change in pressure beneath the surface of the liquid at depth Δh due to the weight of a liquid of density p. - g is the gravitational field strength. - This pressure acts equally in all directions at depth Δh and depends only on Δh and p. - Value will be in pascals (Pa) if Δh = is in meters (m) and (p) is in kilograms per cubic meter (kg/m³). ## 2. Thermal Physics ### 2.1. Kinetic Particle Model of Matter #### States of Matter - Matter consists of tiny particles like molecules and atoms. - Matter exists as solids, liquids, or gases, each with different characteristics. - Solids have a definite shape and volume, and particles are close together in fixed positions. - Liquids have a definite volume and take the shape of their container. The particles are further apart and can slide over each other. - Gases have no definite shape or volume, and particles move much further apart and freely. #### Brownian Motion - Describes random motion of particles in fluids (liquids and gases) and is caused by collisions with smaller, faster-moving particles.. #### Temperature and kinetic energy - Particles in solids vibrate in fixed positions at room temperature. - Heating solids increases particle vibrations, raising their average kinetic energy. - Cooling a solid reduces particle vibrations until absolute zero (-273°C or 0 K) stops all motion. - Absolute zero is the lowest possible temperature achievable. #### Pressure and Kinetic Energy - Absolute zero, at -273°C or 0 K, is the lowest possible temperature. - Kelvin scale temperatures are derived by adding 273 to Celsius temperatures: $T(K) = θ(°C) +273$. - In the Kelvin scale, all temperatures are always positive and directly proportional to the average kinetic energy of particles. ### 2.2. Thermal properties and temperature #### Thermal expansion of solids, liquids and gases - Solids and Liquids: When heated, particles vibrate more, causing them to push apart slightly, resulting in expansion. - Gases: Heating increases particle speed and collisions with container walls, which causes container expansion to maintain pressure. #### Applications - Bimetallic Strips: Made from metals with different expansion rates (e.g., copper and iron). Used in: - Fire Alarms: Bends to complete an electrical circuit when exposed to heat, triggering alarms. - Thermostats: Maintains temperature by bending to break or complete electrical circuits. #### Internal Energy and Heating - Internal energy increases when an object is heated. - Different materials require varying amounts of heat to raise their temperatures due to differences in specific heat capacity. - Specific heat capacity (c) measures the amount of heat required to raise the temperature of a substance by 1 degree Celsius per unit mass. #### Temperature and Thermal Energy - Temperature is related to the average kinetic energy of particles. - Thermal energy is the total energy of particles in a substance, and more particles can hold more total thermal energy even if they have lower individual particle energies. #### Heat Transfer and Equilibrium - Heat transfers from higher to lower temperature bodies until thermal equilibrium is reached. - This transfer is caused by collisions between particles, making their average kinetic energies equal. #### Specific Heat Capacity - Specific heat capacity (c) is defined as the energy required per unit mass per unit temperature increase, measured in joules per kilogram per degree Celsius (J/(kg°C) - The formula relating heat energy (ΔE), mass (m), specific heat capacity (c), and temperature change (Δθ) is: ΔE = mcΔθ - Specific heat capacity quantifies how much heat energy is needed to raise the temperature of a substance. - Materials with higher specific heat capacities require more heat energy per unit mass to achieve the same temperature change. #### Change of State - Heating can change a solid to a liquid (melting) and a liquid to a solid (freezing). - Pure substances melt and freeze at specific temperatures, such as water at 0°C. - Melting involves particles of a solid overcoming intermolecular forces to become a liquid. - Solidification (freezing) involves the transfer of potential energy from particles to surroundings as a liquid becomes solid. - Vaporisation requires substantial energy to overcome intermolecular forces in a liquid to become gas (vapour). - Condensation involves gas particles losing potential energy to their surroundings as they return to a liquid state. #### Evaporation - Higher temperatures, larger surface areas, and wind or draughts increase the rate of evaporation. - Energy is transferred to the surroundings from the liquid during evaporation, cooling the liquid. - Evaporation cools the body through sweat, helping to maintain a constant temperature. #### Differences between boiling and evaporation - Boiling occurs at a specific temperature. Bubbles of vapour form within the liquid. Requires sufficient heat to reach boiling point. Rapid compared to evaporation. Happens throughout the entire volume of the liquid. - Evaporation occurs at any temperature below the boiling point. Occurs at the surface of the liquid. Requires less heat and occurs due to energetic particles escaping. Slower compared to boiling. Happens only at the liquid's surface. ## 2.3. Transfer of thermal energy ### Conduction - Conduction is heat transfer through matter from hot to cold without moving matter. - Metals conduct heat well (e.g., copper, aluminum); insulators (wood, plastic) are poor conductors. - Metals feel colder due to rapid heat transfer from the hand compared to insulators at the same temperature. - Liquids and gases conduct heat slowly because the particles are further apart and need time to transfer energy to each other. - Metals transfer heat via fast-moving free electrons, raising temperatures in cooler areas and lattice vibrations. - Non-metals transfer heat through slower atomic or molecular vibrations, lacking free electrons. ### Convection - Heat transfer method in fluids like liquids and gases. - Transfer of thermal energy by movement of the matter itself. ### Convection Currents - Warm fluids rise because they expand and become less dense. - Cooler, denser fluids sink and replace the rising warm fluid. - This movement of fluids due to temperature differences is known as a convection current. ### Radiation - A method of thermal energy transfer which occurs without matter, even in vacuum. - Emits as electromagnetic waves, travels at speed of light. ### Absorption and Reflection - Surfaces vary in radiation absorption.. - Black surfaces absorb more than shiny white ones. ### Greenhouse effect - Greenhouse gases trap heat similar to glass in a greenhouse. - Balance between incoming solar radiation and emitted Earth radiation crucial for climate stability. - Increased carbon dioxide and methane upset this balance and absorb more infrared which cannot escape. ## 3. Waves ### 3.1. General Properties of Waves - Progressive waves carry energy from one place to another without transferring matter. ### Two Types of Progressive Waves - Transverse waves - Longitudinal waves ### Reflection of a wave at a plane surface - Straight water waves (transverse waves) hit a metal strip in a ripple tank at æ° angle. - Angle of incidence (i) and angle of reflection (r)are both x°. - Angle of incidence equals angle of reflection: (i = r) - Same wavelength as reflected wave - Same wave speed ### Refraction - Continuous straight waves in shallow water have shorter wavelengths than in deeper water. - Waves in shallower water have a smaller speed and smaller wavelength. - When waves move from shallow to dense regions, they bend towards the normal, and when they move from dense to shallow regions, they bend away from the normal. ### Diffraction - Straight water waves meet narrow gaps and create circular wavefronts. - Diffraction can also occur at the edges of obstacles causing wave spreading. ### 3.2. Reflection and Refraction of Light - Light travels in a path called a ray. - A beam is a stream of light shown by several rays. - Beams can be parallel, diverging, or converging. ### Speed of Light - The speed of light is about 1 million times faster than the speed of sound. - The speed of light is 3 × 10⁸ meters per second. ### Reflection of light against a plane mirror - The normal is perpendicular to the mirror at the point where the incident ray strikes. - The angle of incidence (i) is between the incident ray and the normal. - The angle of reflection (r) is between the reflected ray and the normal. - The law of reflection states that the angle of incidence is equal to the angle of reflection. ### Real and Virtual Images - A real image can be produced on a screen and is formed by rays that pass through the screen. - A virtual image cannot be formed on a screen. - A virtual image is produced by rays that seem to come from it but do not pass through it. - The image in a plane mirror is virtual. Rays from an object are reflected at the mirror and appear to come from a point behind the mirror where the rays would meet when extrapolated (extended) backward. ### Refractive Index - Light refracts due to speed change when entering a different medium. - In air, light travels at 300,000 km/s (3 × 10⁸ m/s) - In glass, it slows to 200,000 km/s (2 × 10⁸ m/s) - Refractive index (n) is the ratio of light speed in air to light speed in the medium. - For glass: $n = 300,000km/s/200,000km/s = 1.5$. - Refractive index is the ratio of speeds of a wave in two different regions. - Experimentally it is true that $n = sin(i)/sin(r)$ where (i) is the angle in air and (r) is the angle in glass. - Higher refractive index means greater bending of light as it slows down more. ### Critical Angle - When light passes from an optically denser to an optically less dense medium at small angles of incidence, there is a strong refracted ray and a weak reflected ray. - Increasing the angle of incidence increases the angle of refraction. - Critical angle (c) occurs when the angle of refraction is 90°. - For angles of incidence greater than (c), light undergoes total internal reflection. - Total internal reflection means that the light does not cross the boundary and reflects inside the denser medium. - For the critical angle: $sin(c) = 1/n$ where n is the refractive index. ## 3.3. Lenses ### Converging and Diverging Lenses - A converging (or convex) lens is thickest in the center and bends light inwards. - A diverging (or concave) lens is the thinnest in the center and spreads the light out. - The center of a lens is its optical center (C) and the line through C at right angles to the lens is the principal axis. ### Principal focus - When a beam of light (parallel to the principal axis) passes through a converging lens, it refracts to converge at a point called the principal focus (F). - The principal focus of a converging lens is a real focus. - A diverging lens has a virtual principal focus behind the lens, from which the refracted beam appears to diverge. - A lens has two principal focuses, one on each side, each equidistant from the optical center (C). - The distance (CF) is the focal length (f) of the lens. ### Ray diagrams - A ray parallel to the principal axis is refracted through the principal focus (F). - A ray through the optical center (C) is undeviated (not refracted) for a thin lens. - A ray through the principal focus (F) is refracted parallel to the principal axis. - The intersection of rays (in one beam) after refraction gives the location of the image. ## 3.4. Dispersion of Light ### Refraction by a Prism - In a triangular glass prism, a ray bends due to refraction at each surface. - The bending at the first surface combines with the bending at the second surface. - This combined change in direction is called the deviation. - Unlike in a parallel-sided block, where the emergent (exiting) ray remains parallel to the incident ray, these bendings do not cancel out in a prism. ### Dispersion - When white light passes through a triangular glass prism, it separates into a band of colors known as a spectrum. - This separation of colors is called dispersion and occurs because the refractive index of glass varies with the wavelength of light. - White light consists of many colors with different wavelengths, and the prism separates them based on their refractive indices. - The colors of the visible spectrum, from longest to shortest wavelength, are: red, orange, yellow, green, blue, indigo, and violet - Red light, with the longest wavelength and lowest frequency, is refracted the least by the prism. - Violet light, with the shortest wavelength and highest frequency, is refracted the most by the prism. ## 3.5. Electromagnetic Spectrum ### Light waves and Electromagnetic Spectrum - Light is part of the electromagnetic spectrum, which extends beyond visible light in both directions (with greater wavelength vs. smaller wavelength) - The spectrum includes gamma rays, X-rays, ultraviolet, infrared, microwaves, and radio waves. - Wavelength increases from gamma rays to radio waves, while frequency increases from radio waves to gamma rays. ### Properties of Electromagnetic Waves - Electromagnetic waves travel at the speed of light in a vacuum, approximately 3 × 10⁸ m/s. - They can undergo reflection, refraction, and diffraction and are transverse waves. - They follow the wave equation $v = fλ$, where (v) is the speed of light, (f) is the frequency, and (λ) is the wavelength. - Higher frequency means smaller wavelength and therefore more energy carried. ### Radio Waves and Microwaves - Radio waves are the longest-wavelength electromagnetic waves used for communication, radio and television transmission, astronomy, and radio frequency identification. - Microwaves have shorter wavelengths than radiowaves and are used in telecommunications, satellite communication, radar, and microwave ovens. ### Infrared Radiation - Detected as heat by the body; used in thermal imaging, heating, and remote controls. - Also used in communication (optical fibers), electric grills, and intruder alarms. - However, high-intensity infrared can cause burns and eye damage. ### Visible Light - Red light has the longest wavelength, and violet light has the shortest. - Monochromatic light consists of one color (single frequency), where frequency is more responsible than wavelength to express the colour. - Visible light enables vision and is used for illumination and photography. - Optical instruments like microscopes and telescopes use light properties to form images. ### Ultraviolet Radiation - Shorter wavelengths than visible light; causes sunburn and skin damage. - Used in fluorescent applications (e.g., security marking, water treatment, artificial skin-tanning) and sterilising water. - Can be harmful in high doses. ### X-rays and Gamma Rays - X-rays have smaller wavelengths than ultraviolet rays and are used in medical imaging, security screening, and industrial inspection. - Gamma rays are highly penetrating (smallest wavelength and largest frequency) and used in cancer detection cancer treatment to kill cells, sterilization, and material inspection. ### Communication Systems - Below are the differences between digital and analog signals: | Aspect | Digital Signals | Analog Signals | |-----------------|-----------------|-----------------| | Signal Type | Discrete, binary (0s and 1s) | Continuous, varying amplitude and frequency | | Transmission Rate | Higher transmission rates | Limited by bandwidth and signal degradation over distance | | Examples | Digital data, internet signals, computer memory | Audio signals, analog television, older telephone systems | ### Infrared Optical Fibers - Infrared optical fibers use the principle of total internal reflection to make infrared or light travel along the fiber without much loss. - Used for long-distance data transmission, offering high bandwidth and low signal loss compared to electrical transmission. ## 3.6. Sound ### Longitudinal Waves - Sound waves are longitudinal - Particles in the medium (like air or water molecules) vibrate back and forth parallel to the direction of wave propagation. - This creates areas of compression (where molecules are closer together) and rarefaction (where they are far apart) as the wave travels through the medium. - The movement of particles transfers energy through the medium. - This vibration causes the sound to be heart. ### Compressions and Rarefactions - Sound waves are made of compressions (C) and rarefactions (R) as they move through a medium. - Compressions are regions where air molecules are densely packed together and the regions have higher pressure. - Rarefactions are regions where air molecules are less densely packed and the regions have lower pressure. ### Frequency and Wavelength - Frequency (f) of a sound wave is the number of complete wave cycles per second and is measured in Hertz (Hz). - Higher frequencies mean higher-pitch sounds, while lower frequencies mean lower-pitch sounds. - Wavelength (λ) is the distance between two consecutive compressions or rarefactions in a sound wave. - The speed of sound (v) in a medium is determined by the product of its frequency and wavelength: (v = fλ). ### Limits of hearing - Humans can only hear sound frequencies ranging from about 20Hz (low pitch) to 20,000Hz (high pitch). - The upper limit of audibility decreases with age due to changes in the sensitivity of the ear. - Audibility can also be affected by the intensity (loudness) of the sound which is determined by amplitude. ### Reflection of sound (Echo) - Sound waves reflect off hard and flat surfaces like how light reflects off a mirror. - When sound reflects, it creates an echo, which is a repetition of the original sound heard after a short delay. ### Speed of Sound - The speed of sound in air is approximately 330-350 meters per second (m/s) at room temperature. - In other materials, such as water (with a speed of approx. 1500m/s) or steel (with a speed of approx. 5100 m/s), the speed of sound may vary due to differences in the density. - Temperature affects the speed of sound in air and it increases with temperature because warmer air molecules move faster. ### Measurement of the Speed of Sound - Echo Method - Stand at a known distance (like more than 300 meters) from a large wall. - Clap hands or produce a sharp sound and start a stopwatch simultaneously. - Wait for the distinct echo from the surface and stop the stopwatch when you hear it. - Calculation: Use the formula (v = 2d/t), where (d) is the distance to the surface and (t) is the time interval measured with the stopwatch. ### Note: Twice the distance is used because the sound travels away from you and then back again, covering the entire distance two times. ### Direct Method - Place two microphones a known distance (like 10 meters) apart. - Connect microphones to an oscilloscope to detect the sound. - Produce a sound source equidistant from both points and start timing when the sound is produced. - Stop timing when the sound is detected at the second point. - Use the formula (v = d/t), where (d) is the known distance between the two microphones and (t) is the measured time interval between detection at the two points. ### Musical Notes - The pitch of the note is determined by the frequency of the sound wave (higher frequencies produce higher pitch notes). - Loudness is determined by the amplitude of vibrations ( greater amplitude produces louder sounds) - Quality (timbre) of a sound is its unique shape or texture, caused by the instrument's construction. ### Ultrasound - Ultrasound refers to sound waves with frequencies above the upper limit of human hearing (> 20,000Hz). - It is used in medical imaging and industrial applications for precision and non-destructive testing. - Ultrasound waves behave similarly to audible sound waves but can penetrate materials and provide detailed imaging without harmful effects. ## 4. Electricity and Magnetism ### 4.1. Simple Phenomena of Magnetism #### Magnetic Materials - Ferromagnetic materials like iron can be made into magnets. - Magnetic materials are naturally attracted to magnets even when not magnetized.. #### Magnetic Poles - Magnetic poles attract magnetic materials and are found near the ends of magnets. - Poles always come in pairs: north and south. - Every magnet has a North Pole (N) and a South Pole (S). - The North Pole of a magnet points towards the Earth's geographic North Pole. #### Law of Magnetic Poles - Similar poles (N – Nor S - S) repel each other. - Opposite poles (N - S) attract each other. - The attraction or repulsion decreases as poles move farther apart. #### Induced Magnetism - Magnetic materials can become magnetized when near a magnet. #### Magnetisation of Iron and Steel - Iron nails and steel paper clips can be magnetised by hanging them from a magnet. - Each nail or clip magnetises the next in a chain, with unlike poles attracting each other. - Removing an iron chain by pulling the top nail causes it to collapse because iron shows temporary magnetism. - Steel chains do not collapse when removed because they have permanent magnetism. - Soft materials (e.g. iron) are easily magnetised but lose magnetism quickly. - Hard materials (e.g. steel) are harder to magnetise but remain magnetised longer. #### Magnetic and Non-magnetic Materials - Magnetic materials (iron, steel, nickel, cobalt) are attracted to magnets and can be magnetised. - Non-magnetic materials (e.g., aluminium, wood) are not attracted to magnets and cannot be magnetised. #### Magnetic Fields - A magnetic field is the region around a magnet where magnetic forces act. - Field strength is higher where magnetic field lines are closer together and lower where they are further apart. - Magnetic fields are shown using lines of force, showing the direction from North to South poles. - The density of these lines indicates field strength: closer lines represent stronger magnetic fields. #### Electromagnets - They are formed from a coil of wire through which an electrical current passes. - Magnetism is temporary and can be switched on and off, unlike permanent magnets. - They contain a core of soft iron that only becomes magnetised when current flows through the coil. #### Factors Affecting Electromagnet Strength - Current Increase: Higher current in the coil results in stronger magnetism. - More Turns: Increasing the number of turns in coils around the core increases magnet strength. - Closer Poles: Moving the magnetic poles closer together increases electromagnet strength. ## 4.2. Electrical quantities ### Electric Charge - Like/same charges (+ and + or - and -) repel, while unlike charges (+ and -) attract. ### Force Between Charges - The force between electric charges decreases as their separation increases. - Positive charges repel other positive charges and attract negative charges. - Negative charges repel other negative charges and attract positive charges. ### Charges, Atoms, and Electrons - Atoms consist of a central nucleus with protons (positive) and electrons (negative) orbiting around it. - Protons and electrons have equal but opposite charges, making atoms electrically neutral overall. ### Production of Charges - Charges are produced by friction, which transfers electrons between materials. - Electrons move between materials during rubbing; protons remain in the nuclei and do not move. ### Units of charge - Charge is measured in coulombs (C) and defined in terms of the ampere (A). - The charge on an electron is (e = 1.6 × 10-¹⁹) C. ### Electrons, Insulators, and Conductors - Insulators: Electrons are firmly bound to atoms; rubbing can charge them statically. - Conductors: Electrons can move freely; they require insulation to hold a charge. | Type | Description | Examples | |-------------------|---------------------------------------------------------------------------|---------------------------------------------------------------------------| | Insulators | Electrons are firmly bound to atoms; rubbing can charge them statically. | Plastics (polythene, cellulose acetate), Perspex, nylon | | Conductors | Electrons can move freely; require insulation to hold a charge. | Metals, carbon | ### Electric Fields - When charges are near each other, they experience a force known as the electric force. - Electric field is a region where a charge feels a force due to nearby charges. - Uniform electric field exists between oppositely charged parallel metal plates, shown by evenly spaced lines perpendicular to the plates. - Electric field direction is indicated by arrows, showing the force acting towards a small positive charge (acting away from negative charges). ### The Ampere and the Coulomb (units of current and charge) - Electric Current is defined as charge passing a point per unit time, symbolized as $I = Q/t$. - Unit of current is the ampere (A), with one milliampere ( mA) equal to one-thousandth of an ampere and is measured by an ammeter. - Unit of charge is the coulomb (C), defined as the charge passing a point when a steady current of 1 ampere flows for 1 second (1C = 1As). ### Charge Calculation - $Q=Ixt$ where Q is charge, I is current, and t is time in seconds. ### Conventional Current - Conventional current flows from positive to negative terminals of a battery, opposite to electron flow. - Circuit diagrams show conventional current direction with arrows, while electrons move in the opposite direction. ### Direct and Alternating Current | | Direct Current (d.c.) |