Drug Design Strategies PDF
Document Details
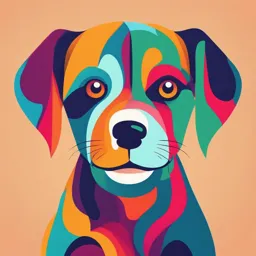
Uploaded by MagicFrancium
Tags
Related
- Organic Chemistry - Drug Distribution & Computer Aided Drug Design PDF
- Organic Pharmaceutical Chemistry Lecture 4 - Drug Metabolism PDF
- Organic Pharmaceutical Chemistry - Third Stage - PDF
- Advanced Medicinal Chemistry Past Paper PDF - GUJARAT TECHNOLOGICAL UNIVERSITY - Winter 2023
- Introduction to Medicinal Chemistry PDF
- Pharmaceutical Organic Chemistry Answer Key PDF
Summary
This document discusses drug design strategies, focusing on drug distribution and oral administration. It explains how drugs are distributed in the body after oral administration, considering factors like chemical structure, particle size, and crystal form. The document also touches on prodrugs, parenteral administration, and the importance of metabolism and excretion.
Full Transcript
Drug Design Strategies 1. DRUG DISTRIBUTION A drug is a chemical molecule. Following introduction into the body, a drug must pass through many barriers, survive alternate sites of attachment and storage, and avoid significant metabolic destruction before it reaches the site o...
Drug Design Strategies 1. DRUG DISTRIBUTION A drug is a chemical molecule. Following introduction into the body, a drug must pass through many barriers, survive alternate sites of attachment and storage, and avoid significant metabolic destruction before it reaches the site of action, usually a receptor on or in a cell. Distribution after Oral Administration When the drug is administered orally, the drug must go into solution to pass through the gastrointestinal mucosa. Even drugs administered as true solutions may not remain in solution as they enter the acidic stomach and then pass into the alkaline intestinal tract. The ability of the drug to dissolve is governed by several factors, including: 1. Its chemical structure. 2. Variation in particle size and particle surface area. 3. Nature of the crystal form. 3 4. Type of tablet coating. 5. Type of tablet matrix. By varying the dosage form and physical characteristics of the drug, it is possible to have a drug dissolve quickly or slowly. Chemical modification is also used to a limited extent to facilitate a drug reaching its desired target. Any compound passing through the gastrointestinal tract will encounter a large number and variety of digestive and bacterial enzymes, which, in theory, can degrade the drug molecule, therefore, a new drug molecule under investigation will likely be dropped from further consideration if it cannot survive in the intestinal tract or its oral bioavailability is low, leading to necessitating an alternate route, including parenteral, buccal, or transdermal, when there is no effective alternative for this new drug, or this new drug is more effective than existing products and can be administered by one of these alternate routes. An example is olsalazine, used in the treatment of ulcerative colitis. This drug is a dimer of the pharmacologically active mesalamine (5-aminosalicylic acid). The latter is not effective orally because it is metabolized to inactive forms before reaching the colon. The dimeric form passes through a significant portion of the intestinal tract before being cleaved by the intestinal bacteria to two equivalents of mesalamine. In contrast, these same digestive enzymes can be used to be advantageous. Example, chloramphenicol is water soluble enough (2.5 mg/mL) to come in contact with the taste receptors on the tongue, producing an unpalatable bitterness. To mask this intense bitter taste, the palmitic acid moiety is added as an ester of the primary hydroxyl of chloramphenicol (i.e. chloramphenicol palmitate). This reduces the parent drug’s water solubility (1.05 mg/mL), enough so that it can be formulated as a suspension that passes over the bitter taste receptors on the tongue. Once in the intestinal tract, the ester linkage is hydrolyzed by the digestive esterases to the active antibiotic chloramphenicol and the very common dietary fatty acid palmitic acid. 4 Olsalazine and chloramphenicol palmitate are examples of prodrugs. Most prodrugs are compounds that are inactive in their native form but are easily metabolized to the active agent. Olsalazine and chloramphenicol palmitate are examples of prodrugs that are cleaved to smaller compounds, one of which is the active drug. Occasionally, the prodrug approach is used to enhance the absorption of a drug that is poorly absorbed from the gastrointestinal tract. Enalapril is the ethyl ester of enalaprilic acid, an active inhibitor of angiotensin-converting enzyme (ACE). The ester prodrug is much more readily absorbed orally than the pharmacologically active carboxylic acid. Unless the drug is intended to act locally in the gastrointestinal tract, it will have to pass through the gastrointestinal mucosal barrier into venous circulation to reach the site of the receptor. This journey involves distribution or partitioning between: 1. The aqueous environment of the gastrointestinal tract, 2. The lipid bilayer cell membrane of the mucosal cells, 3. Possibly the aqueous interior of the mucosal cells, 4. The lipid bilayer membranes on the venous side of the gastrointestinal tract, 5. The aqueous environment of venous circulation. 5 Parenteral Administration Many times, there will be therapeutic advantages in bypassing the intestinal barrier by using parenteral (injectable) dosage forms. These include: 1. Patients who, because of illness, cannot tolerate or are incapable of accepting drugs orally. 2. Some drugs are so rapidly and completely metabolized to inactive products in the liver (first-pass effect) that oral administration is precluded. This is does not mean the drug administered by injection is not opposed by some of the obstacles which can occur regardless to route of administration. These are: 1. Intravenous administration places the drug directly into the circulatory system, where it will be rapidly distributed throughout the body, which can put the drug (in addition to the receptors) in to many unwanted places including tissue depots and the liver (where most biotransformations occur). 2. In comparison to intravenous administration, subcutaneous and intramuscular injections show slow distribution of the drug, because it must diffuse from the site of injection into systemic circulation. These parenteral routes produce a depot in the tissues from which the drug must reach the blood or lymph. Once in systemic circulation, the drug will undergo the same distributive phenomena as orally and intravenously administered agents before reaching the target receptor. 3. The blood-brain barrier, BBB, permeation: like other route of administration, parenterally administered drugs may not cross the BBB which is composed of membranes of tightly joined epithelial cells lining the cerebral capillaries which protects the brain from exposure to a large number of metabolites and chemicals. The net result is that the brain is not exposed to the same variety of compounds that other organs are. This makes it is possible to bypass the BBB by injecting the drug directly into specific organs or areas of the body. Intraspinal (ex. local anesthetics) and intracerebral routes will place the drug directly into the spinal fluid or brain, respectively. The prodrug approach can also be used to alter the solubility characteristics, which, in turn, can increase the flexibility in formulating dosage forms. For example, the solubility of methylprednisolone can be altered from one to another form as follow: 1. Methylprednisolone: it is slightly water-insoluble and is normally found in tablets. 6 2. Methylprednisolone acetate: it is essentially water-insoluble. This acetate ester is found in topical ointments and sterile aqueous suspensions for intramuscular injection. It is hydrolyzed to the active methylprednisolone by the patient’s own systemic hydrolytic enzymes (esterases). 3. Methylprednisolone sodium succinate: it is water-soluble sodium salt of succinate ester. It is used in oral, intravenous, and intramuscular dosage forms. The succinate esters are hydrolyzed to the active methylprednisolone by the patient’s own systemic hydrolytic enzymes (esterases). Another example of how prodrug design can significantly alter biodistribution and biological half-life illustrated by the two drugs based on the retinoic acid structure used systemically to treat psoriasis, a nonmalignant hyperplasia. Etretinate has a 120- day terminal half-life after 6 months of therapy. In contrast, the active metabolite, acitretin, has a 33-96 hour terminal half-life. Both drugs are potentially teratogenic. Acitretin, with its shorter half-life, is recommended for a woman who would like to become pregnant, because it can clear from her body within a reasonable time frame. 7 Protein Binding Once the drug enters the systemic circulation, it can undergo several events. It may stay in solution, but many drugs will be bound to the serum proteins, usually albumin. The drug can remain in systemic circulation bound to albumin for a considerable period and not be available to the sites of biotransformation, the pharmacological receptors, and excretion. Protein binding can have a profound effect on the: 1. Drug’s effective solubility. A drug with such poor water solubility that therapeutic concentrations of the unbound (active) drug normally cannot be maintained still can be a very effective agent. The albumin–drug complex acts as a reservoir by providing large enough concentrations of free drug to cause a pharmacological response at the receptor. 2. Drug’s biodistribution. Protein binding may also limit access to certain body compartments. The placenta is able to block passage of proteins from maternal to fetal circulation. Thus, drugs that normally would be expected to cross the placental barrier and possibly harm the fetus are retained in the maternal circulation, bound to the mother’s serum proteins. 8 3. Drug’s half-life in the body and drug’s duration of action. The albumin– drug complex acts as a reservoir by providing large enough concentrations of free drug to cause a pharmacological response at the receptor. The drug–protein complex is too large to pass through the renal glomerular membranes, preventing rapid excretion of the drug. Protein binding limits the amount of drug available for biotransformation and for interaction with specific receptor sites. For example, the large, polar trypanocide suramin remains in the body in the protein-bound form for as long as 3 months (t1/2 = 50 days). The maintenance dose for this drug is based on weekly administration. At first, this might seem to be an advantage to the patient, but when the patient have serious adverse reactions, a significant length of time will be required before the concentration of drug falls below toxic levels. 4. Interaction with other drugs. The drug–protein binding phenomenon can lead to some clinically significant drug–drug interactions that result when one drug displaces another from the binding site on albumin. For example, a large number of drugs can displace the anticoagulant warfarin from its albumin- binding sites. This increases the effective concentration of warfarin at the receptor, leading to an increased prothrombin time (increased time for clot formation) and potential hemorrhage. Tissue Depots The drug can also be stored in tissue depots. Neutral fat constitutes some 20% to 50% of body weight and constitutes a depot of considerable importance. The more lipophilic the drug, the more likely it will concentrate in these pharmacologically inert depots. The ultra–short-acting, lipophilic barbiturate thiopental’s concentration rapidly decreases below its effective concentration following administration. It disappears into tissue protein, redistributes into body fat, and then slowly diffuses back out of the tissue depots but in concentrations too low for a pharmacological response. Thus, only the initially administered thiopental is present in high enough concentrations to combine with its receptors. The remaining thiopental diffuses out of the tissue depots into systemic circulation, in concentrations too small to be effective, which then is metabolized in the liver, and is excreted. In general, structural changes in the barbiturate series that favor partitioning into the lipid tissue stores decrease duration of action but increase central nervous system (CNS) depression. Conversely, the barbiturates with the slowest onset of action and longest duration of action contain the more polar side chains. This latter group of 9 barbiturates both enters and leaves the CNS more slowly than the more lipophilic thiopental. Drug Metabolism All substances in the circulatory system, including drugs, metabolites, and nutrients, will pass through the liver. Most molecules absorbed from the gastrointestinal tract enter the portal vein and are initially transported to the liver. A significant proportion of a drug will partition or be transported into the hepatocyte, where it may be metabolized by hepatic enzymes to inactive chemicals during the initial trip through the liver, by what is known as the firstpass effect. Lidocaine is a classic example of the significance of the first-pass effect. Over 60% of this local anesthetic antiarrhythmic agent is metabolized during its initial passage through the liver, resulting in it being impractical to administer orally. When used for cardiac arrhythmias, it is administered intravenously. This rapid metabolism of lidocaine is useful when stabilizing a patient with cardiac arrhythmias. Lidocaine should be administered intravenously in too much quantities where toxic responses will tend to decrease because of rapid biotransformation to inactive metabolites. An understanding of the metabolic labile site on lidocaine led to the development of the primary amine analog tocainide. In contrast to lidocaine’s half-life of less than 2 hours, tocainide’s half-life is approximately 15 hours, with 40% of the drug excreted unchanged. A study of the metabolic fate of a drug is required for all new drug products. Where we have different situation: 1. Active parent drug converted to in active metabolites. Example lidocaine. 10 2. An inactive parent drug that is converted to an active metabolite ex. the nonsteroidal anti-inflammatory agent sulindac being reduced to the active sulfide metabolite, the immunosuppressant azathioprine being cleaved to the purine antimetabolite 6-mercaptopurine, and purine and pyrimidine antimetabolites and antiviral agent acyclovir being converted to their nucleotide form acyclovir triphosphate. 3. Often both the parent drug and its metabolite are active. Example about 75% to 80% of phenacetin (now withdrawn from the U.S. market) is converted to acetaminophen. In the tricyclic antidepressant series, imipramine and amitriptyline are N-demethylated to desipramine and nortriptyline, respectively. Although a drug’s metabolism can be a source of hindrance for the medicinal chemist, pharmacist, and physician and lead to inconvenience and compliance problems with the patient, it is fortunate that the body has the ability to metabolize foreign molecules (xenobiotics). Otherwise, many of these substances could remain in the body for years especially certain lipophilic chemical pollutants, including the once very popular insecticide dichlorodiphenyltrichloroethane (DDT). After entering the body, these chemicals reside in body tissues, slowly diffusing out of the depots and potentially harming the individual on a chronic basis for several years. Excretion The main route of excretion of a drug and its metabolites is - Through the kidney. - For some drugs, enterohepatic circulation, in which the drug reenters the intestinal tract from the liver through the bile duct and be excreted in the feces. - Milk of nursing mothers and so they must be worried, because drugs and their metabolites can be excreted in human milk and be ingested by the nursing infant. Several variables make dosing regimens must be more frequent: - If the situation does not favor formation of the drug–receptor complex, higher and usually more frequent doses must be administered. - If partitioning into tissue stores or metabolic degradation and/or excretion is favored, it will take more of the drug and usually more frequent administration to maintain therapeutic concentrations at the receptor. 11 The Receptors Pharmacological response consists of a drug binding to a specific receptor. Many drug receptors are the same as those used by endogenously produced ligands. Example cholinergic agents and synthetic corticosteroids interact with the same receptors as acetylcholine and cortisone bind to them. Binding of drugs to receptors may produce desired or undesired effects. This is depending on 1. The biological distribution of these receptors. Example, the nonsteroidal anti- inflammatory drugs combine with the desired cyclooxygenase receptors at the site of the inflammation and the undesired cyclooxygenase receptors in the gastrointestinal mucosa, causing severe discomfort and sometimes ulceration. 2. Biological distribution of drugs, i.e. the organs and tissues that can be reached by the drug and contain these receptors. Unlike the first generation antihistamines, the second-generation antihistamines, like fexofenadine, are claimed to cause less sedation because it does not readily penetrate the blood- brain barrier. 3. Various receptors with similar structural requirements are found in several organs and tissues. Drug-receptor interaction is an equilibrium process, equation below. A good ability to fit the receptor favors binding and the desired pharmacological response. In contrast, a poor fit favors the reverse reaction and the amount of drug bound to the receptor is too small which leads to a much smaller pharmacological effect. Many variables contribute to a drug’s binding to the receptor. These include: - The structural classes, since most drugs that belong to the same pharmacological class have certain structural features in common. - The 3D shape of the molecule. Very slight changes in structure could cause significant changes in biological activity. These structural variations could increase or decrease activity or change an agonist into an antagonist. - The types of chemical bonding involved in the binding of the drug to the receptor. 12 The initial receptor model was based on a rigid lock-and-key concept, with the drug (key) fitting into a receptor (lock). It has been used to explain why certain structural features produce a predictable pharmacological action. Recent model must realize that both the drug and the receptor can have considerable flexibility. The receptor can undergo an adjustment in 3D structure when the drug makes contact, i.e. the drug docks with the receptor. The fit of drugs onto or into macromolecules is not always an all-or-none process as pictured by the earlier lock-and-key concept of a receptor. Rather, it appears to be a continuous process, as indicated by regular increase and decrease in biological activity of a homologous series of drugs. Drug-receptor association may produce productive changes in the configuration of the macromolecule, leading to agonist responses, an antagonistic or blocking response. Similarly, strong drug-receptor associations may lead to unproductive changes in the configuration of the macromolecule, leading to an antagonistic or blocking response. Acid–Base Properties of Drugs Most drugs used today can be classified as acids or bases. A drug’s acid–base properties can greatly influence its biodistribution and partitioning characteristics. In their definition, an acid is defined as a proton donor and a base is defined as a proton acceptor. Un-ionized acids, like carboxylic acids, donate their protons forming ionized conjugate bases, carboxylate. In contrast, ionized acids, like ammonium compounds, donate proton and yield un-ionized conjugate bases (amine derivatives). Similarly for un-ionized and ionized bases accept protons and yield their ionized and un-ionized conjugated acids respectively. Acid/Conjugated Base and Base/Conjugated Acid Pairs In biological systems, drug molecules face water everywhere and an acid-base reaction can occur. Water is an amphoteric molecule, can be either a weak base accepting a proton from acidic drugs to form the strongly acidic hydrated proton or hydronium ion (H3O+), or a weak acid donating a proton to a basic drug to form the strongly basic hydroxide anion (OH-). 13