Industrial Media and the Nutrition of Industrial Organisms PDF
Document Details
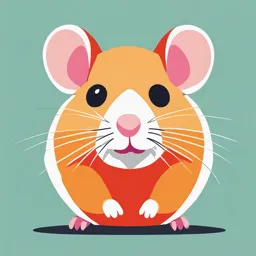
Uploaded by FinerNephrite7027
West Visayas State University
Tags
Related
- Industrial Media and the Nutrition of Industrial Organisms PDF
- Industrial Microbiology Chapter 2 PDF (Nile University)
- Industrial Microorganism Lecture Notes PDF
- Industrial Microorganisms: Preservation and Improvement Techniques PDF
- Micro Chapter 4 PDF
- Tema 6. Identificación de Microorganismos Industriales PDF
Summary
This chapter from a textbook on industrial microbiology and biotechnology discusses the nutrient requirements for industrial microorganisms. It details the importance of suitable media for efficient microbial growth in industrial processes, including the need for carbon, nitrogen, minerals, growth factors, and water. The chapter emphasizes the importance of the right balance of nutrients for optimal results.
Full Transcript
54 Modern Industrial Microbiology and Biotechnology CHAPTER 4 Industrial Media and the Nutrition of Industrial Organisms The use of a good, adequate, and industrially usable medium is as important...
54 Modern Industrial Microbiology and Biotechnology CHAPTER 4 Industrial Media and the Nutrition of Industrial Organisms The use of a good, adequate, and industrially usable medium is as important as the deployment of a suitable microorganism in industrial microbiology. Unless the medium is adequate, no matter how innately productive the organism is, it will not be possible to harness the organism’s full industrial potentials. Indeed not only may the production of the desired product be reduced but toxic materials may be produced. Liquid media are generally employed in industry because they require less space, are more amenable to engineering processes, and eliminate the cost of providing agar and other solid agents. 4.1 THE BASIC NUTRIENT REQUIREMENTS OF INDUSTRIAL MEDIA All microbiological media, whether for industrial or for laboratory purposes must satisfy the needs of the organism in terms of carbon, nitrogen, minerals, growth factors, and water. In addition they must not contain materials which are inhibitory to growth. Ideally it would be essential to perform a complete analysis of the organism to be grown in order to decide how much of the various elements should be added to the medium. However, approximate figures for the three major groups of heterotrophic organisms usually grown on an industrial scale are available and may be used in such calculations (Table 4.1). Carbon or energy requirements are usually met from carbohydrates, notably (in laboratory experiments) from glucose. It must be borne in mind that more complex carbohydrates such as starch or cellulose may be utilized by some organisms. Furthermore, energy sources need not be limited to carbohydrates, but may include hydrocarbons, alcohols, or even organic acids. The use of these latter substrates as energy sources is considered in Chapters 15 and 16 where single cell protein and yeast productions are discussed. In composing an industrial medium the carbon content must be adequate for the production of cells. For most organisms the weight of organism produced from a given weight of carbohydrates (known as the yield constant) under aerobic conditions is about Industrial Media and the Nutrition of Industrial Organisms 55 Table 4.1 Average composition of microorganisms (% dry weight) Component Bacteria Yeast Molds Carbon 48 (46-52) 48 (46-52) 48 (45-55) Nitrogen 12.5 (10-14) 7.5 (6-8.5) 6 (4-7) Protein 55 (50 –60) 40 (35-45) 32 (25-40) Carbohydrates 9 (6-15) 38 (30-45) 49 (40-55) Lipids 7 (5-10) 8 (5-10) 8 (5-10) Nucleic Acids 23 (15-25) 8 (5-10) 5 (2-8) Ash 6 (4-10) 6 (4-10) 4 (4-10) Minerals (same for all three organisms) Phosphorus 1.0 - 2.5 Sulfur, magnesium 0.3 - 1.0 Potassium, sodium 0.1 - 0.5 Iron 0.01 - 0.1 Zinc, copper, manganese 0.001 – 0.01 0.5 gm of dry cells per gram of glucose. This means that carbohydrates are at least twice the expected weight of the cells and must be put as glucose or its equivalent compound. Nitrogen is found in proteins including enzymes as well as in nucleic acids hence it is a key element in the cell. Most cells would use ammonia or other nitrogen salts. The quantity of nitrogen to be added in a fermentation can be calculated from the expected cell mass and the average composition of the micro-organisms used. For bacteria the average N content is 12.5%. Therefore to produce 5 gm of bacterial cells per liter would require about 625 mg N (Table 4.1). Any nitrogen compound which the organism cannot synthesize must be added. Minerals form component portions of some enzymes in the cell and must be present in the medium. The major mineral elements needed include P, S, Mg and Fe. Trace elements required include manganese, boron, zinc, copper and molybdenum. Growth factors include vitamins, amino acids and nucleotides and must be added to the medium if the organism cannot manufacture them. Under laboratory conditions, it is possible to meet the organism’s requirement by the use of purified chemicals since microbial growth is generally usually limited to a few liters. However, on an industrial scale, the volume of the fermentation could be in the order of thousands of liters. Therefore, pure chemicals are not usually used because of their high expense, unless the cost of the finished material justifies their use. Pure chemicals are however used when industrial media are being developed at the laboratory level. The results of such studies are used in composing the final industrial medium, which is usually made with unpurified raw materials. The extraneous materials present in these unpurified raw materials are not always a disadvantage and may indeed be responsible for the final and distinctive property of the product. Thus, although alcohol appears to be the desired material for most beer drinkers, the other materials extraneous 56 Modern Industrial Microbiology and Biotechnology to the maltose (from which yeasts ferment alcohol) help confer on beer its distinctive flavor (Chapter 12). 4.2 CRITERIA FOR THE CHOICE OF RAW MATERIALS USED IN INDUSTRIAL MEDIA In deciding the raw materials to be used in the production of given products using designated microorganism(s) the following factors should be taken into account. (a) Cost of the material The cheaper the raw materials the more competitive the selling price of the final product will be. No matter, therefore, how suitable a nutrient raw materials is, it will not usually be employed in an industrial process if its cost is so high that the selling price of the final product is not economic. Thus, although lactose is more suitable than glucose in some processes (e.g. penicillin production) because of the slow rate of its utilization, it is usually replaced by the cheaper glucose. When used, glucose is added only in small quantities intermittently in order to decelerate acid production. Due to these economic considerations the raw materials used in many industrial media are usually waste products from other processes. Corn steep liquor and molasses are, for example, waste products from the starch and sugar industries, respectively. They will be discussed more fully below. (b) Ready availability of the raw material The raw material must be readily available in order not to halt production. If it is seasonal or imported, then it must be possible to store it for a reasonable period. Many industrial establishments keep large stocks of their raw materials for this purpose. Large stocks help beat the ever rising cost of raw materials; nevertheless large stocks mean that money which could have found use elsewhere is spent in constructing large warehouses or storage depots and in ensuring that the raw materials are not attacked during storage by microorganisms, rodents, insects, etc. There is also the important implication, which is not always easy to realize, that the material being used must be capable of long-term storage without concomitant deterioration in quality. (c) Transportation costs Proximity of the user-industry to the site of production of the raw materials is a factor of great importance, because the cost of the raw materials and of the finished material and hence its competitiveness on the market can all be affected by the transportation costs. The closer the source of the raw material to the point of use the more suitable it is for use, if all other conditions are satisfactory. (d) Ease of disposal of wastes resulting from the raw materials The disposal of industrial waste is rigidly controlled in many countries. Waste materials often find use as raw materials for other industries. Thus, spent grains from breweries can be used as animal feed. But in some cases no further use may be found for the waste from an industry. Its disposal especially where government regulatory intervention is rigid could be expensive. When choosing a raw material therefore the cost, if any, of treating its waste must be considered. Industrial Media and the Nutrition of Industrial Organisms 57 (e) Uniformity in the quality of the raw material and ease of standardization The quality of the raw material in terms of its composition must be reasonably constant in order to ensure uniformity of quality in the final product and the satisfaction of the customer and his/her expectations. In cases where producers are plentiful, they usually compete to ensure the maintenance of the constant quality requirement demanded by the user. Thus, in the beer industry information is available on the quality of the barley malt before it is purchased. This is because a large number of barley malt producers exist, and the producers attempt to meet the special needs of the brewery industry, their main customer. On the other hand molasses, which is a major source of nutrient for industrial microorganisms, is a by product of the sugar industry, where it is regarded as a waste product. The sugar industry is not as concerned with the constancy of the quality of molasses, as it is with that of sugar. Each batch of molasses must therefore be chemically analyzed before being used in a fermentation industry in order to ascertain how much of the various nutrients must be added. A raw material with extremes of variability in quality is clearly undesirable as extra costs are needed, not only for the analysis of the raw material, but for the nutrients which may need to be added to attain the usual and expected quality in the medium. (f) Adequate chemical composition of medium As has been discussed already, the medium must have adequate amounts of carbon, nitrogen, minerals and vitamins in the appropriate quantities and proportions necessary for the optimum production of the commodity in question. The demands of the microorganisms must also be met in terms of the compounds they can utilize. Thus most yeasts utilize hexose sugars, whereas only a few will utilize lactose; cellulose is not easily attacked and is utilized only by a limited number of organisms. Some organisms grow better in one or the other substrate. Fungi will for instance readily grow in corn steep liquor while actinomycetes will grow more readily on soya bean cake. (g) Presence of relevant precursors The raw material must contain the precursors necessary for the synthesis of the finished product. Precursors often stimulate production of secondary metabolites either by increasing the amount of a limiting metabolite, by inducing a biosynthetic enzyme or both. These are usually amino acids but other small molecules also function as inducers. The nature of the finished product in many cases depends to some extent on the components of the medium. Thus dark beers such as stout are produced by caramelized (or over-roasted) barley malt which introduce the dark color into these beers. Similarly for penicillin G to be produced the medium must contain a phenyl compound. Corn steep liquor which is the standard component of the penicillin medium contains phenyl precursors needed for penicillin G. Other precursors are cobalt in media for Vitamin B12 production and chlorine for the chlorine containing antibiotics, chlortetracycline, and griseofulvin (Fig. 4.1). (h) Satisfaction of growth and production requirements of the microorganisms Many industrial organisms have two phases of growth in batch cultivation: the phase of growth, or the trophophase, and the phase of production, or the idiophase. In the first phase cell multiplication takes place rapidly, with little or no production of the desired 58 Modern Industrial Microbiology and Biotechnology Left: Vitamin B12. Please note that cobalt is highlighted. It must be present in the medium in which organisms producing the vitamin are grown Right: Top; The general structure of tetracyclines. Bottom; The structure of 7-Chlortetracycline; a chlorine atom is present in position 7. Chlorine must be present in the medium for producing chlortetracyline; note that chlorine is highlighted in position 7 in chlortetracycline. Fig. 4.1 Vitamin B12 and Chlortetracycline Showing Location of Components Present as Precursors in the Medium material. It is in the second phase that production of the material takes place, usually with no cell multiplication and following the elaboration of new enzymes. Often these two phases require different nutrients or different proportions of the same nutrients. The medium must be complete and be able to cater for these requirements. For example high levels of glucose and phosphate inhibit the onset of the idiophase in the production of a number of secondary metabolites of industrial importance. The levels of the components added must be such that they do not adversely affect production. Trophophase- idiophase relationship and secondary metabolites are discussed in detail in Chapter 5. 4.3 SOME RAW MATERIALS USED IN COMPOUNDING INDUSTRIAL MEDIA The raw materials to be discussed are used because of the properties mentioned above: cheapness, ready availability, constancy of chemical quality, etc. A raw material which is Industrial Media and the Nutrition of Industrial Organisms 59 cheap in one country or even in a different part of the same country may however not be cheap in another, especially if it has already found use in some other production process. In such cases suitable substitutes must be found if the goods must be produced in the new location. The use of local substitutes where possible is advantageous in reducing the transportation costs and even creating some employment in the local population. Prior experimentation may however be necessary if such new local materials differ substantially in composition from those already being used. Some well-known raw materials will now be discussed. In addition, some of potential useability will also be examined. (a) Corn steep liquor This is a by-product of starch manufacture from maize. Sulfur dioxide is added to the water in which maize is steeped. The lowered pH inhibits most other organisms, but encourages the development of naturally occurring lactic acid bacteria especially homofermentative thermophilic Lactobacillus spp. which raise the temperature to 38-55°C. Under these conditions, much of the protein present in maize is converted to peptides which along with sugars leach out of the maize and provide nourishment for the lactic acid bacteria. Lactic fermentation stops when the SO2 concentration reaches about 0.04% and the concentration of lactic acid between 1.0 and 1.5%. At this time the pH is about 4. Acid conditions soften the kernels and the resulting maize grains mill better while the gel-forming property of the starch is not hindered. The supernatant drained from the maize steep is corn steep liquor. Before use, the liquor is usually filtered and concentrated by heat to about 50% solid concentration. The heating process kills the bacteria. As a nutrient for most industrial organisms corn steep liquor is considered adequate, being rich in carbohydrates, nitrogen, vitamins, and minerals. Its composition is highly variable and would depend on the maize variety, conditions of steeping, extent of boiling etc. The composition of a typical sample of corn steep liquor is given in Table 4.2. As corn steep liquor is highly acidic, it must be neutralized (usually with CaCO3) before use. (b) Pharmamedia Also known as proflo, this is a yellow fine powder made from cotton-seed embryo. It is used in the manufacture of tetracycline and some semi-synthetic penicillins. It is rich in Table 4.2 Approximate composition of corn steep liquor (%) Lactose 3.0-4.0 Glucose 0.-0.5 Non-reducing carbohydrates (mainly starch) 1.5 Acetic acid 0.05 Glucose lactic acid 0.5 Phenylethylamine 0.05 Amino aids (peptides, mines) 0.5 Total solids 80-90 Total nitrogen 0.15-0.2% 60 Modern Industrial Microbiology and Biotechnology protein, (56% w/v) and contains 24% carbohydrate, 5% oil, and 4% ash, the last of which is rich in calcium, iron, chloride, phosphorous, and sulfate. (c) Distillers solubles This is a by-product of the distillation of alcohol from fermented grain. It is prepared by filtering away the solids from the material left after distilling fermented cereals (maize or barley) for whiskey or grain alcohol. The filtrate is then concentrated to about one-third solid content to give a syrup which is then drum-dried to give distillers soluble. It is rich in nitrogen, minerals, and growth factors (Table 4.3). Table 4.3 Composition of maize distillers soluble % Moisture 5 Protein 27 Lipid 9 Fibre 5 Carbohydrate 43 Ash (mainly K, Na, Mg, CO3, and P) 11 (d) Soya bean meal Soya beans (soja) (Glycine max), is an annual legume which is widely cultivated throughout the world in tropical, sub-tropical and temperate regions between 50°N and 40°S. The seeds are heated before being extracted for oil that is used for food, as an anti- foam in industrial fermentations, or used for the manufacture of margarine. The resulting dried material, soya bean meal, has about 11% nitrogen, and 30% carbohydrate and may be used as animal feed. Its nitrogen is more complex than that found in corn steep liquor and is not readily available to most microorganisms, except actinomycetes. It is used particularly in tetracycline and streptomycin fermentations. (e) Molasses Molasses is a source of sugar, and is used in many fermentation industries including the production of potable and industrial alcohol, acetone, citric acid, glycerol, and yeasts. It is a by-product of the sugar industry. There are two types of molasses depending on whether the sugar is produced from the tropical crop, sugar cane (Saccharum officinarum) or the temperate crop, beet, (Beta alba). Four stages are involved in the manufacture of cane sugar. After crushing, a clear greenish dilute sugar solution known as ‘mixed juice’ is expressed from the canes. During the second stage known as clarification the mixed juice is heated with lime. Addition of lime changes the pH of the juice to alkaline and thus stops further hydrolysis (or inversion) or the cane sugar (sucrose), while heating coagulates proteins and other undesirable soluble portions of the mixed juice to form ‘mud’. The supernatant juice is then concentrated (in the third stage) by heating under high vacuum and increasing low pressures in a series of evaporators. In the fourth and final stage of crystallization, sugar crystals begin to form with increasing heat and under vacuum, yielding a thick brown Industrial Media and the Nutrition of Industrial Organisms 61 syrup which contains the crystals, and which is known as ‘massecuite’. (In the beet industry it is known as ‘fillmass’.) The massecuite is centrifuged to remove the sugar crystals and the remaining liquid is known as molasses. The first sugar so collected is ‘A’ and the liquid is ‘A’ molasses. ‘A’ molasses is further boiled to extract sugar crystals to yield ‘B’ sugar and ‘B’ molasses. Two or more boilings may be required before it is no longer profitable to attempt further extractions. This final molasses is known as ‘blackstrap molasses’. The sugar yielded with the production of black strap molasses is low-grade and brown in color, and known as raw sugar, cargo sugar, or refining sugar. This raw sugar is further refined, in a separate factory, to remove miscellaneous impurities including the brown color (due to caramel) to yield the white sugar used at the table. The heavy liquid discarded from the refining of sugar is known in the sugar refining industry as ‘syrup’ and corresponds to molasses in the raw sugar industry. The above description has been of cane sugar molasses. In the beet sugar industry the processes used in raw refined sugar manufacture are similar, but the names of the different fractions recovered during purification differ. Cane and beet molasses differ slightly in composition (Table 4.4). Beet molasses is alkaline while cane molasses is acid. Table 4.4 Average composition of beet and cane molasses Beet Molasses Cane Molasses % (W/W) % (W/W) Water 16.5 20.0 Sugars: 53.0 64.0 Sucrose 51.0 32.0 Fructose 1.0 15.0 Glucose - 14.0 Raffinose 1.0 - Non-sugar (nitrogeneous Materials, acids, gums, etc.) 19.0 10.0 Ash 11.5 8.0 Even within same type of molasses – beet or cane – composition varies from year to year and from one locality to another. The user industry selects the batch with a suitable composition and usually buys up a year’s supply. For the production of cells the variability in molasses quality is not critical, but for metabolites such as citric acid, it is very important as minor components of the molasses may affect the production of these metabolites. ‘High test’ molasses (also known as inverted molasses) is a brown thick syrup liquid used in the distilling industry and containing about 75% total sugars (sucrose and reducing sugars) and about 18% moisture. Strictly speaking, it is not molasses at all but invert sugar, (i.e reducing sugars resulting from sucrose hydrolysis). It is produced by the hydrolysis of the concentrated juice with acid. In the so-called Cuban method, invertase is used for the hydrolysis. Sometimes ‘A’ sugar may be inverted and mixed with ‘A’ molasses. 62 Modern Industrial Microbiology and Biotechnology (f) Sulfite liquor Sulfite liquor (also called waste sulfite liquor, sulfite waste liquor or spent sulfite liquor) is the aqueous effluent resulting from the sulfite process for manufacturing cellulose or pulp from wood. Depending on the type, most woods contain about 50% cellulose, about 25% lignins and about 25% of hemicelluloses. During the sulfite process, hemicelluloses hydrolyze and dissolve to yield the hexose sugars, glucose, mannose, galactose, fructose and the pentose sugars, xylose, and arabinsoe. The acid reagent breaks the chemical bonds between lignin and cellulose; subsequently they dissolve the lignin. Depending on the severity of the treatment some of the cellulose will continue to exist as fibres and can be recovered as pulp. The presence of calcium ions provides a buffer and helps neutralize the strong lignin sulfonic acid. The degradation of cellulose yields glucose. Portions of the various sugars are converted to sugar sulfonic acids, which are not fermentable. Variable but sometimes large amounts of acetic, formic and glactronic acids are also produced. Sulfite liquor of various compositions are produced, depending on the severity of the treatment and the type of wood. The more intense the treatment the more likely it is that the sugars produced by the more easily hydrolyzed hemicellulose will be converted to sulfonic acids; at the same time the more intense the treatment the more will glucose be released from the more stable cellulose. Hardwoods not only yield a higher amount of sugar (up to 3% dry weight of liquor) but the sugars are largely pentose, in the form of xylose. Hardwood hydrolyzates also contains a higher amount of acetic acid. Soft woods yield a product with about 75% hexose, mainly mannose. Sulfite liquor is used as a medium for the growth of microorganisms after being suitably neutralized with CaCO3 and enriched with ammonium salts or urea, and other nutrients. It has been used for the manufacture of yeasts and alcohol. Some samples do not contain enough assaimilable carbonaceous materials for some modern fermentations. They are therefore often enriched with malt extract, yeast autolysate, etc. (g) Other Substrates Other substrates used as raw materials in fermentations are alcohol, acetic acid, methanol, methane, and fractions of crude petroleum. These will be discussed under Single Cell Protein (Chapter 15). Barley will be discussed in the section dealing with the brewing of beer (Chapter 12). 4.4 GROWTH FACTORS Growth factors are materials which are not synthesized by the organism and therefore must be added to the medium. They usually function as cofactors of enzymes and may be vitamins, nucleotides etc. The pure forms are usually too expensive for use in industrial media and materials containing the required growth factors are used to compound the medium. Growth factors are required only in small amounts. Table 4.5 gives some sources of growth factors. 4.5 WATER Water is a raw material of vital importance in industrial microbiology, though this importance is often overlooked. It is required as a major component of the fermentation Industrial Media and the Nutrition of Industrial Organisms 63 Table 4.5 Some sources of growth factors Growth factor Source Vitamin B Rice polishing, wheat germ, yeasts Vitamin B2 Cereals, corn steep liquor Vitamin B6 Corn steep liquor, yeasts Nicotinamide Liver, penicillin spent liquor Panthothenic Acid Corn steep liquor Vitamin B12 Liver, silage, meat medium, as well as for cooling, and for washing and cleaning. It is therefore used in rather large quantities, and measured in thousands of liters a day depending on the industry. In some industries such as the beer industry the quality of the product depends to some extent on the water. In order to ensure constancy of product quality the water must be regularly analyzed for minerals, color, pH, etc. and adjusted as may be necessary. Due to the importance of water, in situations where municipal water supplies are likely to be unreliable, industries set up their own supplies. 4.6 SOME POTENTIAL SOURCES OF COMPONENTS OF INDUSTRIAL MEDIA The materials to be discussed are mostly found in the tropical countries, including those in Africa, the Caribbean, and elsewhere in the world. Any microbiological industries to be sited in these countries must, if they are not to run into difficulties discussed above, use the locally available substrates. It is in this context that the following are discussed. 4.6.1 Carbohydrate Sources These are all polysaccharides and have to be hydrolyzed to sugar before being used. (a) Cassava (manioc) The roots of the cassava-plant Manihot esculenta Crantz serve mainly as a source of carbohydrate for human (and sometimes animal) food in many parts of the tropical world. Its great advantage is that it is high yielding, requires little attention when cultivated, and the roots can keep in the ground for many months without deterioration before harvest. The inner fleshy portion is a rich source of starch and has served, after hydrolysis, as a carbon source for single cell protein, ethanol, and even beer. In Brazil it is one of the sources of fermentation alcohol (Chapter 13) which is blended with petrol to form gasohol for driving motor vehicles. (b) Sweet potato Sweet potatoes Ipomca batatas is a warm-climate crop although it can be grown also in sub tropical regions. There are a large number of cultivars, which vary in the colors of the tuber flesh and of the skin; they also differ in the tuber size, time of maturity, yield, and sweetness. They are widely grown in the world and are found in South America, the USA, Africa and Asia. They are regarded as minor sources of carbohydrates in comparison with maize, wheat, or cassava, but they have the advantage that they do not require much 64 Modern Industrial Microbiology and Biotechnology agronomic attention. They have been used as sources of sugar on a semi-commercial basis because the fleshy roots contain saccharolytic enzymes. The syrup made from boiling the tubers has been used as a carbohydrate (sugar) source in compounding industrial media. Butyl alcohol, acetone and ethanol have been produced from such a syrup, and in quantities higher than the amounts produced from maize syrup of the same concentration. Since sweet potatoes are not widely consumed as food, it is possible that it may be profitable to grow them for use, after hydrolysis, in industrial microbiology media as well as for the starch industry. It is reported that a variety has been developed which yields up to 40 tonnes per hectare, a much higher yield than cassava or maize. (c) Yams Yams (Dioscorea spp) are widely consumed in the tropics. Compared to other tropical roots however, their cultivation is tedious; in any case enough of this tuber is not produced even for human food. It is therefore almost inconceivable to suggest that the crop should be grown solely for use in compounding industrial media. Nevertheless yams have been employed in producing various products such as yam flour and yam flakes. If the production of these materials is carried out on a sufficiently large scale it is to be expected that the waste materials resulting from peeling the yams could yield substantial amounts of materials which on hydrolysis will be available as components of industrial microbiological media. (d) Cocoyam Cocoyam is a blanket name for several edible members of the monocotyledonous (single seed-leaf) plant of the family Araceae (the aroids), the best known two genera of which are Colocasia (tano) and Xanthosoma (tannia). They are grown and eaten all over the tropical world. As they are laborious to cultivate, require large quantities of moisture and do not store well they are not the main source of carbohydrates in regions where they are grown. However, this relative unimportance may well be of significance in regions where for reasons of climate they can be suitably cultivated. Cocoyam starch has been found to be of acceptable quality for pharmaceutical purposes. Should it find use in that area, starchy by-products could be hydrolyzed to provide components of industrial microbiological media. (e) Millets This is a collective name for several cereals whose seeds are small in comparison with those of maize, sorghum, rice, etc. The plants are also generally smaller. They are classified as the minor cereals not because of their smaller sizes but because they generally do not form major components of human food. They are however hardy and will tolerate great drought and heat, grow on poor soil and mature quickly. Attention is being turned to them for this reason in some parts of the world. It is for this reason also that millets could become potential sources of cereal for use in industrial microbiology media. Millets are grown all over the world in the tropical and sub-tropical regions and belong to various genera: Pennisetum americanum (pearl or bulrush millet), Setaria italica (foxtail millet), Panicum miliaceum (yard millet), Echinochloa frumentacea (Japanese yard millet) and Eleusine corcana (finger millet). Millet starch has been hydrolyzed by malting for alcohol production on an experimental basis as far back as 50 years ago and the Industrial Media and the Nutrition of Industrial Organisms 65 available information should be helpful in exploiting these grains for use as industrial media components. (f) Rice Rice, Oryza sativa is one of the leading food corps of the world being produced in all five continents, but especially in the tropical areas. Although it is high-cost commodity, it has the advantage of ease of mechanization, storability, and the availability of improved seeds through the efforts of the International Rice Research Institute, Philippines and other such bodies. The result is that this food crop is likely in the near future to displace, as a carbohydrate source, such other starch sources as yams, and to a lesser extent cassava in tropical countries. The increase in rice production is expected to become so efficient in many countries that the crop would yield substrates cheap enough for industrial microbiological use. Rice is used as brewing adjuncts and has been malted experimentally for beer brewing. (g) Sorghum Sorghum, Sorghum bicolor, is the fourth in term of quantity of production of the world’s cereals, after wheat, rice, and corn. It is used for the production of special beers in various parts of the world. It has been mechanized and has one of the greatest potential among cereals for use as a source of carbohydrate in industrial media in regions of the world where it thrives. It has been successfully malted and used in an all-sorghum lager beer which compared favorably with barley lager beer (Chapter 12) (h) Jerusalem artichoke Jerusalem artichoke, Helianthus tuberosus, is a member of the plant family compositae, where the storage carbohydrate is not starch, but inulin (Fig. 4.2) a polymer of fructose into which it can be hydrolyzed. It is a root-crop and grows in temperate, semi-tropical and tropical regions. 4.6.2 Protein Sources (a) Peanut (groundnut) meal Various leguminous seeds may be used as a source for the supply of nitrogen in industrial media. Only peanuts (groundnuts) Arachis hypogea will be discussed. The nuts are rich in liquids and proteins. The groundnut cake left after the nuts have been freed of oil is often used as animal feed. But just as is the case with soya bean, oil from peanuts may be used as anti-foam while the press-cake could be used for a source of protein. The nuts and the cake are rich in protein. (b) Blood meal Blood consists of about 82% water, 0.1% carbohydrate, 0.6% fat, 16.4% nitrogen, and 0.7% ash. It is a waste product in abattoirs although it is sometimes used as animal feed. Drying is achieved by passing live steam through the blood until the temperature reaches about 100°C. This treatment sterilizes it and also causes it to clot. It is then drained, pressed to remove serum, further dried and ground. The resulting blood-meal is chocolate-colored and contains about 80% protein and small amounts of ash and lipids. 66 Modern Industrial Microbiology and Biotechnology Fig. 4.2 Structure of Inulin. This Polymer of Fructose Replaces Starch in some Plants Where sufficient blood is available blood meal could form an important source of proteins for industrial media. (c) Fish Meal Fish meal is used for feeding farm animals. It is rich in protein (about 65%) and, minerals (about 21% calcium 8%, and phosphorous 3.5%) and may therefore be used for industrial microbiological media production. Fish meal is made by drying fish with steam either aided by vacuum or by simple drying. Alternatively hot air may be passed over the fish placed in revolving drums. It is then ground into a fine powder. 4.7 THE USE OF PLANT WASTE MATERIALS IN INDUSTRIAL MICROBIOLOGY MEDIA: SACCHARIFICATION OF POLYSACCHARIDES The great recommendation of plant agricultural wastes as sources of industrial microbiological media is that they are not only plentiful but that in contrast with petroleum, a major source of chemicals, they are also renewable. Serious consideration has therefore been given, in some studies, to the possibility of deriving industrial microbiological raw materials not just from wastes, but from crops grown deliberately for the purpose. However, plant materials in general contain large amounts of polysaccharides which are not immediately utilizable by industrial microorganisms and which will therefore need to be hydrolyzed or saccharified to provide the more available sugars. Thereafter the sugars may be fermented to ethyl alcohol for use as a chemical feed Industrial Media and the Nutrition of Industrial Organisms 67 stock. The plant polysaccharides whose hydrolysis will be discussed in this section are starch, cellulose and hemicelluloses. 4.7.1 Starch Starch is a mixture of two polymers of glucose: amylose and amylopectin. Amylose is a linear (1 ® 4) µ – D glucan usually having a degree of polymerization (D.P., i.e. number of glucose molecules) of about 400 and having a few branched residues linked with (1 ® 6) bondings. Amylopectin is a branched D glucan with predominantly µ – D (1 ® 4) linkages and with about 4% of the µ – D (1 ® 6) type (Fig. 4.3). Amylopectin consists of amylose – like chains of D. P. 12 – 50 linked in a number of possible manners of which 3 in Fig. 4.4 seems most generally accepted. A comparison of the properties of amylose and amylopectin is given in Table 4.6. Table 4.6 Some properties of amylose and amylopectin Property Amylose Amylopectin Structure Linear Branched Behavior in water Precipitates Irreversibly Stable Degree of polymerization 103 104 -105 Average chain length 103 20-25 Hydrolysis to maltose (%) (a) b - amylase 87 54 (b) b - amylase and debranching enzyme 98 79 Iodine Complex max (nm) 650 550 Starches from various sources differ in their proportion of amylopectin and amylose. The more commonly grown type of maize, for example, has about 26% of amylose and 74% of amylopectin (Table 4.7). Others may have 100% amylopectin and still others may have 80 – 85% of amylose. 4.7.1.1 Saccharification of starch Starch occurs in discrete crystalline granules in plants, and in this form is highly resistant to enzyme action. However when heated to about 55°C – 82°C depending on the type, starch gelatinizes and dissolves in water and becomes subject to attack by various enzymes. Before saccharification, the starch or ground cereal is mixed with water and heated to gelatinize the starch and expose it to attack by the saccharifying agents. The gelatinization temperatures of starch from various cereals is given in Table 12.1. The saccharifying agents used are dilute acids and enzymes from malt or microorganisms. 4.7.1.1.1 Saccharification of starch with acid The starch-containing material to be hydrolyzed is ground and mixed with dilute hydrochloric acid, sulfuric acid or even sulfurous acid. When sulfurous acid is used it can be introduced merely by pumping sulfur dioxide into the mash. 68 Modern Industrial Microbiology and Biotechnology Top: a – D (1 ® 4) (left) and a – D (1 ® 6) (right) Bottom: Part structure of amylose (a) and amylopectin (b) 0 = glucose units joined by a – D (I ® 4) linkages ® = a – D (1-6) linkages Fig. 4.3 Linkages of D-glucose Industrial Media and the Nutrition of Industrial Organisms 69 Table 4.7 Amylose contents of some starches Source Amylose Content % Potato 20-22 Corn 20-27 Wheat 18-26 Oat 22-24 Waxymaize 0-5 Cassava 17-20 Sorghum 25-28 Rice 16-18 o = Terminal non-reducing end – groups l = Reducing end group ® = a - D - (1 ® 6) linkage — = Chain of 20 to 25 a - D - (1 ® 4) linked D-glucose residues Fig. 4.4 Diagrams Representing Three Proposed Structures of Amylopectin The concentrations of the mash and the acid, length of time and temperature of the heating have to be worked out for each starch source. During the hydrolysis the starch is broken down from starch (about 2,000 glucose molecules) through compounds of decreasing numbers of glucose moieties to glucose. The actual composition of the hydrolysate will depend on the factors mentioned above. Starch concentration is particularly important: if it is too high, side reactions may occur leading to a reduction in the yield of sugar. At the end of the reaction the acid is neutralized. If it is desired to ferment the hydrolysate for ethanol, yeast or single cell production, ammonium salts may be used as they can be used by many microorganisms. 70 Modern Industrial Microbiology and Biotechnology 4.7.1.1.2 Use of enzymes Enzymes hydrolyzing starch used to be called collectively diastase. With increased knowledge about them, they are now called amylases. Enzymatic hydrolysis has several advantages over the use of acid: (a) since the pH for enzyme hydrolysis is about neutral, there is no need for special vessels which must stand the high temperature, pressure, and corrosion of acid hydrolysis; (b) enzymes are more specific and hence there are fewer side reactions leading therefore to higher yields; (c) acid hydrolysis often yields salts which may have to be removed constantly or periodically thereby increasing cost; (d) it is possible to use higher concentrations of the substrates with enzymes than with acids because of enzyme specificity, and reduced possibility of side reactions. 4.7.1.1.3 Enzymes involved in the hydrolysis of starch Several enzymes are important in the hydrolysis of starch. They are divisible into six groups. (i) Enzymes that hydrolyse a – 1, 4 bonds and by-pass a – I, 6 bonding: The typical example is a - amylase. This enzyme hydrolyses randomly the inner (1 ® 4) - a - D - glucosidic bonds of amylose and amylopectin (Fig. 4.3). The cleavage can occur anywhere as long as there are at least six glucose residues on one side and at least three on the other side of the bond to be broken. The result is a mixture of branched a - limit dextrins (i.e., fragments resistant to hydrolysis and contain the a - D (1 6) linkage (Fig. 4.4) derived from amylopectin) and linear glucose residues especially maltohexoses, maltoheptoses and maltotrioses. a - Amylases are found in virtually every living cell and the property and substrate pattern of a - amylases vary according to their source. Thus, animal a - amylases in saliva and pancreatic juice completely hydrolyze starch to maltose and D-glucose. Among microbial a - amylases some can withstand temperatures near 100°C. (ii) Enzymes that hydrolyse the a – 1, 4 bonding, but cannot by-pass the a – 1,6 bonds: Beta amylase: This was originally found only in plants but has now been isolated from micro-organisms. Beta amylase hydrolyses alternate a – 1,4 bonds sequentially from the non-reducing end (i.e., the end without a hydroxyl group at the C – 1 position) to yield maltose (Figs. 4.3 and 4.5). Beta amylase has different actions on amylose and amylopectin, because it cannot by-pass the a – 1:6 – branch points in amylopectin. Therefore, while amylose is completely hydrolyzed to maltose, amylopectin is only hydrolyzed to within two or three glucose units of the a – 1.6 - branch point to yield maltose and a ‘beta-limit’ dextrin which is the parent amylopectin with the ends trimmed off. Debranching enzymes (see below) are able to open up the a – 1:6 bonds and thus convert beta-limit dextrins to yield a mixture of linear chains of varying lengths; beta amylase then hydrolyzes these linear chains. Those chains with an odd number of glucose molecules are hydrolyzed to maltose, and one glucose unit per chain. The even numbered residues are completely hydrolyzed to maltose. In practice there is a very large population of chains and hence one glucose residue is produced for every two chains present in the original starch. Industrial Media and the Nutrition of Industrial Organisms 71 o = glucose units joined by a-D-(1 ® 4) bonds l = non-reducing ends of chains — = point of attack by enzyme Fig. 4.5 Pattern of Attack of Alpha Amylase and Beta-amylase on Amylose and Amylopectin Respectively 72 Modern Industrial Microbiology and Biotechnology (iii) Enzymes that hydrolyze (a —1, 4 and a — 1:6 bonds: The typical example of these enzymes is amyloglucosidase or glucoamylase. This enzyme hydrolyzes a - D - (1 ® 4) -D – glucosidic bonds from the non-reducing ends to yield D – glucose molecules. When the sequential removal of glucose reaches the point of branching in amylopectin, the hydrolysis continues on the (1 ® 6) bonding but more slowly than on the (1 ® 4) bonding. Maltose is attacked only very slowly. The end product is glucose. (iv) De-branching enzymes: At least two de-branching enzymes are known: pullulanase and iso-amylase. Pullulanase: This is a de-branching enzyme which causes the hydrolysis of a — D – (1 ® 6) linkages in amylopectin or in amylopectin previsouly attacked by alpha- amylase. It does not attack a - D (1 ® 4) bonds. However, there must be at least two glucose units in the group attached to the rest of the molecules through an a -D- (1 ® 6) bonding. Iso-amylase: This is also a de-branching enzyme but differs from pullulanase in that three glucose units in the group must be attached to the rest of the molecules through an a - D – (1 ® 6) bonding for it to function. (v) Enzymes that preferentially attack a - 1, 4 linkages: Examples of this group are glucosidases. The maltodextrins and maltose produced by other enzymes are cleaved to glucose by a - glucosidases. They may however sometime attack unaltered polysaccharides but only very slowly. (vi) Enzymes which hydrolyze starch to non-reducing cyclic D-glucose polymers known as cyclodextrins or Schardinger dextrins: Cyclic sugar residues are produced by Bacillus macerans. They are not acted upon by most amylases although enzymes in Takadiastase produced by Aspergillus oryzae can degrade the residues. 4.7.1.1.4 Industrial saccharification of starch by enzymes In industry the extent of the conversion of starch to sugar is measured in terms of dextrose equivalent (D.E.). This is a measure of the reducing sugar content, expressed in terms of dextrose, determined under defined conditions involving Fehling’s solution. The D.E is calculated as percentage of the total solids. For the saccharification of starch in industry acid is being replaced more and more by enzymes. Sometimes acid is used only initially and enzymes employed at a later stage. Acid saccharification has a practical upper limit of 55 D.E. Beyond this, breakdown products begin to accumulate. Furthermore, with acid hydrolysis reversion reactions occur among the sugar produced. These two deficiencies are avoided when enzymes are utilized. Besides, by selecting enzymes specific sugars can be produced. Starch-splitting enzymes used in industry are produced in germinated seeds and by micro-organisms. Barley malt is widely used for the saccharification of starch. It contains large amounts of various enzymes notably b-amylase and a - glucosidase which further split saccharides to glucose. All the enzymes discussed above are produced by different micro-organisms and many of these enzymes are available commercially. The most commonly encountered organisms producing these enzymes are Bacillus spp, Streptomyeces spp, Aspergillus spp, Penicillium spp, Mucor spp and Rhizopus spp. Industrial Media and the Nutrition of Industrial Organisms 73 4.7.2 Cellulose, Hemi-celluloses and Lignin in Plant Materials 4.7.2.1 Cellulose Cellulose is the most abundant organic matter on earth. Unfortunately it does not exist pure in nature and even the purest natural form (that found in cotton fibres) contains about 6% of other materials. Three major components, cellulose, hemi-cellulose and lignin occur roughly in the ratio of 4:3:3 in wood. Before looking more closely at cellulose, the other two major components of plant materials will be briefly discussed. 4.7.2.2 Hemicelluloses These are an ill-defined group of carbohydrates whose main and common characteristic is that they are soluble in, and hence can be extracted with, dilute alkali. They can then be precipitated with acid and ethanol. They are very easily hydrolyzed by chemical or biological means. The nature of the hemicellulose varies from one plant to another. In cotton the hemicelluloses are pectic substances, which are polymers of galactose. In wood, they consist of short (DP less than 200) branched heteropolymers of glucose, xylose, galactose, mannose and arabinose as well as uronic acids of glucose and galactose linked by 1 – 3, 1 – 6 and 1 – 4 glycosidic bonding. 4.7.2.3 Lignin Lignin is a complex three-dimensional polymer formed from cyclic alcohols. (Fig. 4.6). It is important because it protects cellulose from hydrolysis. Cellulose is found in plant cell-walls which are held together by a porous material known as middle lamella. In wood the middle lamella is heavily impregnated with lignin which is highly resistant and thus protects the cell from attack by enzymes or acid. 4.7.2.4 Pretreatment of cellulose-containing materials before saccharification In order to expose lignocellulosics to attack, a number of physical and chemical methods are in use, or are being studied, for altering the fine structure of cellulose and/or breaking the lignin-carbohydrate complex. Table 4.8 Various pretreatment methods used in lignocellulose substrate preparation Pretreatment type Specific method Mechanical Weathering and milling-ball, fitz, hammer, roller Irradiation Gamma, electron beam, photooxidation Thermal Autohydrolysis, steam explosion, hydrothermolysis, boiling, pyrolysis, moist or dry heat expansion Alkali Sodium hydroxide, ammonium hydroxide Acids Sulfuric, hydrochloric, nitric, phosphoric, maleic Oxidizing agents Peracetic acid, sodium hypochlorite, sodium chlorite, hydrogen peroxide Solvents Ethanol, butanol, phenol, ethylamine, acetone, ethylene glycol Gases Ammonia, chlorine, nitrous oxide, ozone, sulfur dioxide Biological Ligninolytic fungi 74 Modern Industrial Microbiology and Biotechnology Fig. 4.6 Generalized Structure of Lignin Chemical methods include the use of swelling agents such a NaOH, some amines, concentrated H2SO4 or HCI or proprietary cellulose solvents such as ‘cadoxen’ (tris thylene-diamine cadmium hydroxide). These agents introduce water between or within the cellulose crystals making subsequent hydrolysis, easier. Steam has also been used as a swelling agent. The lignin may be removed by treatment with dilute H2SO4 at high temperature. Physical methods of pretreatment include grinding, irradiation and simply heating the wood. 4.7.2.5 Hydrolysis of cellulose Following pretreatment, wood may be hydrolyzed with dilute HCI, H2SO4 or sulfites of calcium, magnesium or sodium under high temperature and pressure as described for sulfite liquor production in paper manufacture see section 4. above). When, however, the aim is to hydrolyze wood to sugars, the treatment is continued for longer than is done for paper manufacture. A lot of experimental work has been done recently on the possible use of cellulolytic enzymes for digesting cellulose. The advantage of the use of enzymes rather than harsh chemicals methods have been discussed already. Fungi have been the main source of cellulolytic enzymes. Trichoderma viride and T. koningii have been the most efficient cellulase producers. Penicillicum funiculosum and Fusarium solani have also been shown to possess equally potent cellulases. Cellulase has been resolved into at least three components: C1, Cx, and b-glucosidases. The C1 component attacks crystalline cellulose and loosens the cellulose chain, after which the other enzymes can attack cellulose. The Industrial Media and the Nutrition of Industrial Organisms 75 Cx enzymes are b - (1 ® 4) glucanases and hydrolyse soluble derivatives of cellulose or swoollen or partially degraded cellulose. Their attack on the cellulose molecule is random and cellobiose (2-sugar) and cellotroise (3-sugar) are the major products of their actions. There is evidence that the enzymes may also act by removing successive glucose units from the end of a cellulose molecule. b-glucosidases hydrolyze cellobiose and short- chain oligo-saccharides derived from cellulose to glucose, but do not attack cellulose. They are able to attack cellobiose and cellotriose rapidly. Many organisms described in the literature as ‘cellulolytic’ produce only Cx and b-glucosidases because they were isolated initially using partially degraded cellulose. The four organisms mentioned above produce all three members of the complex. 5 4.7.2.4.1 Molecular structure of cellulose Cellulose is a linear polymer of D-glucose linked in the Beta-1, 4 glucosidic bondage. The bonding is theoretically as vulnerable to hydrolysis as the one in starch. However, cellulose – containing materials such as wood are difficult to hydrolyze because of (a) the secondary and tertiary arrangement of cellulose molecules which confers a high crystallinity on them and (b) the presence of lignin. The degree of polymerization (D. P.) of cellulose molecule is variable, but ranges from about 500 in wood pulp to about 10,000 in native cellulose. When cellulose is hydrolyzed with acid, a portion known as the amorphous portion which makes up 15% is easily and quickly hydrolyzed leaving a highly crystalline residue (85%) whose DP is constant at 100-200. The crystalline portion occurs as small rod-like particles which can be hydrolyzed only with strong acid. (Fig. 4.7) A = Original cellulose fibril B = Initial attack on amorphous region C = Residue crystalline region D = Attack on crystalline region Fig. 4.7 Diagram Illustrating Breakdown of Crystalline Cellulose 76 Modern Industrial Microbiology and Biotechnology SUGGESTED READINGS Barnes, A.C. 1974. The Sugar cane. Wiley, New York, USA. Dahod, S.K. 1999. Raw Materials Selection and Medium Development for Industrial Fermentation Processes. In: Manual of Industrial Microbiology and Biotechnology. A. L. Demain and J. E. Davies (eds) American Society for Microbiology Press. 2nd Ed, Washington DC. Demain, A.L. 1998. Induction of microbial secondary Metabolism International Microbiology 1, 259–264. Flickinger, M.C., Drew, S.W. (eds) 1999. Encyclopedia of Bioprocess Technology - Fermentation, Biocatalysis, and Bioseparation, Vol 1-5. John Wiley, New York. Ward, W.P., Singh, A. 2004. Bioethanol Technology: Developments and Perspectives Advance in Applied Microbiology, 51, 53 – 80.