Nucleotides Metabolism (Pyrimidine) PDF
Document Details
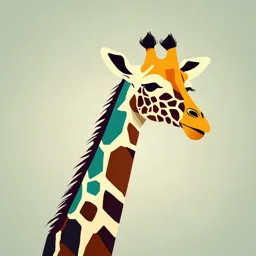
Uploaded by PolishedMistletoe
Tags
Summary
This document provides an overview of pyrimidine nucleotide metabolism. It covers topics such as pyrimidine biosynthesis, the de novo pathway, the salvage pathway, and the formation of various pyrimidine nucleotides. The text also details various pyrimidine analogues that exhibit diverse biological activities, and the implications of pyrimidine metabolism's alterations, such as the hereditary orotic aciduria.
Full Transcript
Pyrimidine Pyrimidine nucleotides, in common with purine nucleotides, are required for the synthesis of DNA and RNA. They also participate in intermediary metabolism (biosynthesis of glycogen and of phospholipids) Biosynthesis of pyrimidine nucleotides can occur by a de novo pathway or...
Pyrimidine Pyrimidine nucleotides, in common with purine nucleotides, are required for the synthesis of DNA and RNA. They also participate in intermediary metabolism (biosynthesis of glycogen and of phospholipids) Biosynthesis of pyrimidine nucleotides can occur by a de novo pathway or by the reutilization of preformed pyrimidine bases or ribonucleosides (salvage pathway). De Novo Synthesis The biosynthesis of pyrimidine nucleotides may be conveniently considered in two stages: the formation of uridine monophosphate (UMP) and the conversion of UMP to other pyrimidine nucleotides. Formation of UMP The synthesis of UMP starts from glutamine, bicarbonate, and ATP, and requires six enzyme activities. 1. The pyrimidine base is formed first and then the nucleotide by the addition of ribose 5-phosphate from PRPP. In contrast, in de novo purine nucleotide biosynthesis, ribose 5-phosphate is an integral part of the earliest precursor molecule. 2. In the biosynthesis of both pyrimidine and urea (or arginine), carbamoyl phosphate is the source of carbon and nitrogen atoms. In pyrimidine synthesis, carbamoyl phosphate serves as donor of the carbamoyl group to aspartate with the formation of carbamoyl aspartate. Formation of UMP 2. Carbamoyl phosphate synthetase I (CPS I) is located in the inner membrane of mitochondria in the liver and, to a lesser extent, in the kidneys and small intestine. It supplies carbamoyl phosphate for the urea cycle. CPS I is specific for ammonia as nitrogen donor and requires N- acetylglutamate as activator. Carbamoyl phosphate synthetase II (CPS II) is present in the cytosol. It supplies carbamoyl phosphate for pyrimidine nucleotide biosynthesis and uses the amido group of glutamine as nitrogen donor. Formation of UMP 3. In mammalian tissue, the six enzymes are encoded by three genes. One gene codes for a multifunctional polypeptide (Pyr 13) that is located in the cytosol and has carbamoyl phosphate synthetase II, aspartate transcarbamoylase, and dihydroorotase activity. The second gene codes for dihydro-orotate dehydrogenase, which is located on the outer side of the inner mitochondrial membrane. Dihydro-orotate, the product of Pyr 13, passes freely through the outer mitochondrial membrane and converts to orotate. Orotate readily diffuses to the cytosol for conversion to UMP. The third gene codes for another multifunctional polypeptide known as UMP synthase (Pyr 5,6). Pyr 5,6 contains orotate phosphoribosyltransferase and orotidylate (orotidine-50- monophosphate) decarboxylase activity. Use of multifunctional polypeptides is very efficient, since the intermediates neither accumulate nor become consumed in side reactions. They are rapidly channeled without dissociation from the polypeptide. Formation of UMP 4. Conversion of dihydroorotate to orotate is catalyzed by dihydroorotate dehydrogenase, a metalloflavoprotein that contains nonheme iron atoms and flavin adenine nucleotides (FMN and FAD). In this reaction, the electrons are probably transported via iron atoms and flavin nucleotides that are reoxidized by NAD+. 5. Biosynthesis of purine and pyrimidine nucleotides requires carbon dioxide and the amide nitrogen of glutamine. Both use an amino acid “nucleus”—glycine in purine biosynthesis and aspartate in pyrimidine biosynthesis. Both use PRPP as the source of ribose 1-phosphate. Formation of UMP 6. UMP is converted to UTP by uridylate kinase and nucleoside diphosphate kinase. UMP + ATP uridylate kinase UDP + ADP UDP + ATP nucleoside diphosphate kinase UTP + ADP Formation of Other Pyrimidine Nucleotides UMP is the parent compound in the synthesis of cytidine and deoxycytidine phosphates and thymidine nucleotides (which are deoxyribonucleotides). Synthesis of Cytidine Nucleotides Synthesis of Thymidine Nucleotides Salvage Pathways Pyrimidines derived from dietary or endogenous sources are salvaged efficiently in mammalian systems. They are converted to nucleosides by nucleoside phosphorylases and then to nucleotides by appropriate kinases. Pyrimidine Analogues This group of compounds includes several drugs that are clinically useful in the treatment of neoplastic disease, psoriasis, and infections caused by fungi and DNA-containing viruses. An antifungal agent, flucytosine (5-fluorocytosine), acts through conversion to 5-fluorouracil by cytosine deaminase in the fungal cells. Idoxuridine (iododeoxyuridine), another halogenated pyrimidine derivative, is used in viral infections. It is a competitive inhibitor (via phosphorylated derivatives) of the incorporation of thymidylic acid into DNA. Cytarabine (cytosine arabinoside) is an analogue of 20- deoxycytidine. Phosphorylated derivatives of cytarabine inhibit nucleic acid synthesis as well as being incorporated into nucleic acids. Pyrimidine Analogues 6-Azauridine and its triacetyl derivative, azaribine, inhibit pyrimidine biosynthesis. Azaribine is better absorbed than 6- azauridine, but it is converted in the blood to 6-azauridine, which undergoes intracellular transformation to 6-azauridylic acid (6-AzUMP). 6- AzUMP, a competitive inhibitor of orotidylate decarboxylase, blocks formation of UMP (Figure 25.20), resulting in high rates of excretion of orotic acid and orotidine in the urine. 5-Azacytidine, after conversion to a phosphorylated derivative, arrests the DNA synthesis phase of the cell cycle. It also affects methylation of certain bases, which leads to hypomethylation. Azathymidine, 30-azido-30-deoxythymidine (AZT), after conversion to the corresponding 50-triphosphate, inhibits viral reverse transcriptase. Hence, AZT is used in the treatment of AIDS. COORDINATION OF PURINE AND PYRIMIDINE NUCLEOTIDE BIOSYNTHESIS A balanced synthesis of pyrimidine and purine nucleotides is essential for the biosynthesis of nucleic acids in growing cells. Several mechanisms exist for coordinating nucleotide synthesis 1. PRPP affects purine and pyrimidine nucleotide biosynthesis. PRPP formation is activated by inorganic phosphate and inhibited by several end products of pathways that use PRPP. In the purine de novo pathway, PRPP activates amidophosphoribosyltransferase and is the rate-limiting substrate for the enzyme. In purine salvage pathways, PRPP is the substrate for HGPRT and APRT. In the pyrimidine pathway, PRPP activates CPS II, may induce Pyr 13, and is a rate-limiting substrate for orotate phosphoribosyltransferase. Thus, changes in the levels of PRPP bring about concordant changes, while enhanced utilization by one pathway might result in reciprocal changes in the other. This interrelationship between the synthesis of purine and pyrimidine nucleotides was seen in a patient who had a deficiency of PRPP synthetase and exhibited orotic aciduria and hypouricemia, consistent with decreased synthesis of purine and pyrimidine nucleotides. COORDINATION OF PURINE AND PYRIMIDINE NUCLEOTIDE BIOSYNTHESIS 2. Coordination of synthesis of purine and pyrimidine nucleotides is affected by activation of CPS II by ATP. 3. Cultured mammalian cells (e.g., human lymphocytes) fail to grow when exposed to adenosine and have increased pools of ADP, ATP, and GTP; decreased pools of UDP, UTP, and CTP; and decreased incorporation of [14C] orotic acid. These effects are reversed by exogenous uridine. These results suggest that adenosine inhibits the conversion of orotic acid to orotidine-5’-monophosphate. Adenosine deaminase reduces the toxic effect of adenosine by converting it to inosine. Increased levels of purine nucleotides may also inhibit PRPP synthesis. This inhibition of formation of pyrimidine nucleotides in the presence of excess purine nucleosides and nucleotides has been termed pyrimidine starvation. CATABOLISM OF PYRIMIDINE NUCLEOTIDES Pyrimidine catabolism occurs mainly in the liver. In contrast to purine catabolism, pyrimidine catabolism yields highly soluble end products. ABNORMALITIES OF PYRIMIDINE METABOLISM Hereditary orotic aciduria is a rare autosomal recessive trait. In this disorder, both orotate phosphoribosyltransferase and orotidine-50-monophosphate decarboxylase activities (reactions 5 and 6 in Figure 25.16) are markedly deficient. Orotic aciduria is characterized by failure of normal growth and by the presence of hypochromic erythrocytes and megaloblastic bone marrow, none of which is improved by the usual hematinic agents (e.g., iron, pyridoxine, vitamin B12, and folate). Leukopenia (decreased number of white blood cell) is also present. Treatment with uridine (24 g/d) results in marked improvement in the hematological abnormalities, in growth and development, and in decreased excretion of orotic acid. ABNORMALITIES OF PYRIMIDINE METABOLISM These patients are pyrimidine auxotrophs and require an exogenous source of pyrimidine just as all humans need vitamins, essential amino acids, and essential fatty acids. Deficiency of folate or vitamin B12 can cause hematological changes similar to hereditary orotic aciduria. Folate is directly involved in thymidylic acid synthesis and indirectly involved in the metabolic functions of vitamin B12. Orotic aciduria without the characteristic hematological abnormalities occurs in disorders of the urea cycle that lead to the accumulation of carbamoyl phosphate in mitochondria. The carbamoyl phosphate exits from the mitochondria and augments cytosolic pyrimidine biosynthesis. ABNORMALITIES OF PYRIMIDINE METABOLISM Treatment with allopurinol or 6-azauridine also produces orotic aciduria as a result of inhibition of orotidine-5’- phosphatedecarboxylase by their metabolic products.