Emmetropisation Notes PDF
Document Details
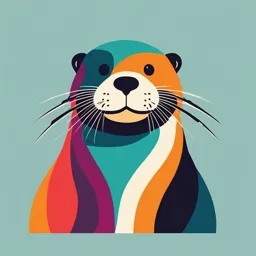
Uploaded by ComplementaryLorentz
Tags
Summary
This document provides an overview of emmetropization, the active process of the visual system during the first years of life that aims to correct refractive error towards zero. It notes that most people aren't truly emmetropic and often slightly hyperopic. The processes and mechanism behind this are discussed, along with the genetic input and development of refractive error.
Full Transcript
Emmetropisation WHAT IS EMMETROPISATION? Emmetropisation is the active process of the visual system during the first years of life that aims to move the refractive error towards zero (emmetropia). It involves the coordination of the growth of the refractive components of the eye such as the lens, c...
Emmetropisation WHAT IS EMMETROPISATION? Emmetropisation is the active process of the visual system during the first years of life that aims to move the refractive error towards zero (emmetropia). It involves the coordination of the growth of the refractive components of the eye such as the lens, cornea and axial length, with defocus detection signals from the retina and visual cortex. This leads to active structural changes allowing the power and focal length of the lens and cornea to precisely match the axial length of the growing eye. In the first three years of life, the increase in axial length from birth would lead to an approximate 20-dioptre shift if it weren't naturally counteracted by changes in the lens and cornea. Although during emmetropisation the refractive error changes towards emmetropia, very few eyes in childhood or adulthood are actually emmetropic, with most of the population being slightly hyperopic (~+0.50). Note: this becomes latent hyperopia with the in-built accommodation reflex. Emmetropisation primarily occurs in the 1st year of life from 3-9 months, with little small changes occurring from 9-18 months. The range of refractive error then remains quite stable until the distribution widens again at around 8 years of age (due to myopia progression in some children). Astigmatism undergoes emmetropisation too leading to an overall reduction in the mean astigmatic error in the first 12 months of life. The emmetropisation mechanism The precise mechanism is as yet unknown. The involved signalling loop is very complex and requires precision to ~0.1mm. The process is thought to be mediated by retinal blur: 1. The detection of blur 2. Release of factors triggering signalling cascades and protein transcription. 3. Changes in the collagen content and ECM of the sclera 4. Axial elongation 5. Reduced retinal blur Genetic input into the emmetropisation process Genes produce the enzymes, growth factors and structural proteins required to generate a particular focal plane at birth, and manage the movement of this plane with eye growth. These genes include those controlling: Scleral fibroblast proliferation Fibroblast collagen production Quantity and width of the scleral lamellae Scleral composition (ie. amount of glycosaminoglycans, and presence of integrin-mediated cell adhesions). DEVELOPMENT OF REFRACTIVE ERROR The development of refractive error is due to a mismatch between axial length and eye refractive power, which may be caused by: Inappropriate input (environmental factors) Dysfunction of the signalling loop (genetic factors) Children with connective tissues disorders such as Marfan’s or Stickler syndrome or may have a dysfunction in emmetropisation loop at the level of scleral collagen remodelling, causing large refractive errors. Children with disabilities such as Down’s syndrome or cerebral palsy may also have impaired emmetropisation and commonly have low hypermetropia. After emmetropisation, the mechanism remains active and alters the gene-mediated growth of the eye as required to maintain emmetropia as the eye elongates slightly with growth. Axial length increases 2 to 4 mm between ages 3 and 13. This requires a refractive adjustment of ~3 diopters in order to preserve emmetropia (this has been found to be a mostly lenticular change while the cornea remains stable). Patients may have ongoing elongation until their early 20s. A dysfunction of the emmetropisation loop may occur in the first 3 years of life when the process is most active, or may be influenced by environmental factors later in life. For example decreased time outdoors and increased near work during schooling may lead to hyperopic defocus and the development of school myopia. Changes in refractive error At birth, there is a wide distribution of refractive error, centering around 2.00D of hyperopia. From birth, the mean value shifts towards lower hyperopia during a dramatic loss of refractive error (emmetropisation). This means that very few children commencing school have clinically significant refractive errors, with only ~6% being expected to wear spectacles. At this age, the refractive error distribution curve is not a normal curve due to a very sharp peak at low hyperopia (leptokurtic growth curve). Refractive error at 3 months (dotted line), 9 months (bold line), and adulthood (thin line) Refractive Error Refractive error refers to an abnormality in the way light is focused by the eye, resulting in blurred or distorted vision. Normally, light enters the eye and is refracted by the cornea and the lens to form a clear image on the retina. However, in individuals with refractive error, the shape or length of the eye causes the light to focus improperly, leading to visual disturbances. There are several types of refractive error, each with its distinct characteristics, which will be explained in detail throughout the module. The impact of refractive error on visual function can vary depending on the severity of the condition and the individual's visual demands. Uncorrected refractive error can lead to a range of symptoms, including blurry vision, eyestrain, headaches, and difficulty in reading or performing daily tasks. LEARNING OBJECTIVES The key learning outcomes for this week are: Explain the concept of hyperopia, myopia, astigmatism and presbyopia Describe the process and mechanism of emmetropisation Describe the mechanism of accommodation and its role in refractive error, including the pathophysiology of the decline in accommodation with age Describe the clinical presentation of myopia, hyperopia and presbyopia Describe the pathophysiology of myopia progression and the evidence-based management options Discuss the spectacle, contact lens and surgical management of refractive error, including when these options are appropriate Identify the consequences of uncorrected hyperopia THE REFRACTIVE ERRORS Emmetropia When light from infinity is focused correctly on the retina, leading to clear distance vision (A). During near tasks, the target is moved forward and the focal point falls behind the retina (B). This retinal defocus stimulates the reflex accommodation mechanism, which causes an increased curvature of the lens, increasing its power and focussing the light on to the retina (C), allowing near tasks to appear clear. Myopia (short-sightedness) In myopia the eye is too long or the power is too high. The light is focussed in front of the retina, leading to retinal defocus and blurry distance vision (E). As targets move closer, the light rays eventually fall on the retina allowing myopes to see clearly up close (F). Concave/diverging lenses (minus power) diverge the incoming parallel rays from infinity so that they fall on the retinal plane allowing for clear vision in the distance (G). Myopia is known as short-sightedness is because a myope can usually see clearly for near tasks. The level of myopia determines how far away you can see. For example, a -2.50D myope will have clear vision to ~40cm away from their face (1m/2.5), 40cm is their ‘infinity’. Alternatively, a -10D myope will only see ~10cm away from their face (1m/10). Therefore, high myopes will still have difficulty with near tasks at normal working distance (~40cm). Hyperopia (long-sightedness) In hyperopia the eye is too short or the power is too weak. The light is focussed behind the retina, leading to retinal defocus and blurry distance vision (D). Hyperopia is corrected with convex/converging lenses (plus power), which converge the incoming parallel rays from infinity so that they fall on the retinal plane. However, the retinal defocus also stimulates the accommodation mechanism that can move the image to the retinal plane – this means that many patients with low hyperopia commonly have clear distance vision, but may struggle when the accommodative demand is increased during near work. Even still, many are completely asymptomatic, particularly early in life when they have large accommodative reserves. Astigmatism Astigmatism is when the eye has two focal points due to a different curvature of the cornea (or uncommonly the retina) in different meridians. This means that light rays in one meridian has a different focal point to light rays in a perpendicular meridian. Astigmatism can be classified as: With-the-rule (flat meridian at 180°) (A) Against-the-rule (flat meridian at 90°) (B) Oblique (flat meridian at 45° or 135°) Irregular (two meridians are not perpendicular to each other) A: B: Example: With-the-rule astigmatism. The flatter curvature along the 180° meridian leads to horizontal rays being focused more posteriorly into a vertical line. The steeper curvature along the 90° meridian leads to vertical rays being focused more anteriorly into a horizontal line. This leads to a blur zone in between the two focal points (Interval of Sturm). The mid-point between the two meridional focal points is called the circle of least confusion. Astigmatism can be hyperopic or myopic. When writing prescriptions, the most positive power is written first, and the difference to the more negative power is written afterwards, followed by the axis. For example: Hyperopic with-the-rule astigmatism: +2.00/-1.00 x 180 Hyperopic against-the-rule astigmatism: +2.00/-1.00 x 90 Hyperopic oblique astigmatism: +2.00/-1.00 x 45 Myopic with-the-rule astigmatism: -2.00/-1.00 x 180 Presbyopia Presbyopia is an irreversible, age-related impairment of near vision that relates to the loss of accommodative function with age. From birth the accommodative power of the lens gradually decreases – from approximately 15D at birth to 1D before 60 years of age. Presbyopia is when this loss of accommodation becomes symptomatic. This is usually at about 40 years of age, but may be earlier or later depending of the refractive state of the patient and their visual tasks. Symptoms of presbyopia include blurry near vision or an increasing working distance as the accommodative reserve of the patient fails to place the image on the retina when objects are moved too close. LET'S GO! But how do all of these refractive errors develop? As Shakespeare once asked, are people born with refractive error, or do they have refractive error thrust upon them? Well, let's start at the very beginning. After all, it's a very good place to start... (click the NEXT button in the bottom right of the page) Accommodation When focussing on an object at near, the visual cortex detects and processes the retinal disparity and blur of the object seen by both retinas. This stimulates the near triad: Accommodation – to focus the object on the retina Convergence – to converge the eyes so that the object of interest falls on the fovea of both eyes. Pupil constriction – to increase the depth of focus and slightly reduce the accommodative demand Helmholtz theory of accommodation When the ciliary muscle is relaxed the zonules are pulled taut and the lens is less convex, allowing distance targets to be focussed on the retina. When focussing at near, hyperopic defocus is detected by the retina, triggering the accommodation reflex: The ciliary muscle is stimulated by the parasympathetic fibres travelling from the Edinger-Westphal nucleus with CNIII (to ciliary ganglion) and CNV1 (from ciliary ganglion). This leads to: 1. Muscle contraction 2. Forward movement of the ciliary muscle and body. 3. Zonules slackens 4. Lens increases anterior surface curvature and forms a more convex shape 5. Increases lens power allowing the near object to be focussed on the retina Image from: Adler's Physiology of the Eye, 2011 THE NEAR TRIAD The near triad consists of accommodation, eye convergence and pupil constriction. The convergence reflex involves with following: 1. Detection of blur and retinal disparity in the visual cortex 2. Activation of bilateral supramotor nuclei 3. Activation of oculomotor nuclei in the brain stem 4. Activation of the medial longitudinal fasciculus 5. Innervation of medial rectus of both eyes by CNIII 6. Convergence of both eyes Pupil constriction involves the following: 1. Detection of blur in the visual cortex 2. Activation of bilateral pretectal (olivary) nuclei 3. Activation of bilateral Edinger-Westphal nuclei 4. Preganglionic parasympathetic nerves travel with CNIII to the ciliary ganglion in the orbit 5. Postganglionic parasympathetic neurons travelling with CNV1 neurons to the iris sphincter 6. Activation and constriction of the iris sphincter muscle Image from: http://neuroscience.uth.tmc.edu/s3/chapter07.html Myopia Myopia, or short-sightedness is when the eye is too long or in some unusual circumstances, the combined parameters of the lens and cornea make the overall refraction myopic. This causes the light to be focused in front of the retina, leading to defocused image falling on the retina, and blurry distance vision. Concave lenses ('minus powered' lenses) that diverge the light entering the eye push the focal point backwards to fall on the retina and correct the blurred vision. TYPES OF MYOPIA There are a few different types of myopia... Simple myopia Simple myopia may be classified low (more than -0.50D and less than -6.00D) and high (>-6.00D). It is the most common form of myopia and its aetiology is not fully understood. It commonly presents in school-aged children/teenagers and it almost always increases in severity – most progression rates variable and are dependant on many different genetic and environmental factors, but can be ~0.3-0.5 D per year. Progression often continues the until late 20s. Due to the stretching of the retina that occurs with increasing myopia, patients with higher degrees of myopia have an increased likelihood for developing retinal tears (middle image below), holes and/or detachments, as well as the following peripheral retinal/chorioretinal degenerations: Peripapillary atrophy (choroidal or scleral crescents/rings around the optic nerve, often temporally) Lattice degeneration – spindle-shaped areas of retinal thinning with hyperpigmented borders (left image below) Tilted insertion or malinsertion of the optic disc (right image below) Tigroid fundus – atrophic tessellated fundus with increased visibility of the choroidal vessels due to thinning of the retinal pigment epithelium (right image below) Lacquer cracks – Breaks in the choriocapillaris and Bruch's membrane Fuchs' spot at the macula Pavingstone degeneration – areas of chorioretinal atrophy showing the underlying sclera Posterior staphyloma – extreme stretching and thinning of the sclera leads to ectasia posteriorly Loss of choroidal circulation due to the stretching and thinning of the globe Choroidal neovascularisation can develop secondary to this if the retina is deprived for oxygen Pathologic myopia Pathologic myopia is defined as excessive myopic axial elongation that leads to structural changes in the posterior eye. This may include conditions that lead to the loss of visual acuity, such as myopic maculopathy, posterior staphyloma, and optic neuropathy associated with high myopia. Psuedomyopia Psuedomyopia is the product of over-reactive accommodation, leading to the appearance of myopia. This may be due to a spasm of the ciliary body. Nocturnal myopia In the dark there are less visual cues that can signal the accommodative system. In these situations, the accommodative system often adopts a default (tonic) accommodative position. This results in a myopic shift causing retinal defocus in the distance and can lead to symptoms of blurry vision at night, particularly noticed as difficulty driving. MYOPIA EITOLOGY The etiology of myopia is still relatively poorly understood. The genetic heritability of myopia is well-documented with current rates of inheritance at approximately: 7% if no parents have it, 23- 40% is one parent has it, and 33-60% if both parents have it (Note: the risk is higher if parents have high myopia). This is due to the inheritance of genes coding for the shape of the eye, and how it goes throughout life (such as axial length, anterior chamber depth, lens thickness and corneal curvature. Specific gene loci mutations have been identified for high (pathologic) myopia. These are primarily autosomal dominant or X-linked inherited and are named MYP1-13. These mutations may code for proteins, growth factors or enzymes in outer retina (eg. causing cone or ON-bipolar cell dysfunction, affecting blur detection) and/or the sclera (affecting collagen or ECM composition and metabolism). While pathological myopia has been mostly contributed to genetic mutations, the etiology of simple/school-aged myopia is more complex and thought to be multifactorial. There is likely to be a large numbers of genes having a small effect and a major influence by environmental factors. The rise in myopia in the last few decades, particularly in east Asian countries (more than 80% of school-leavers are myopic, and ~15% are highly myopic), provides a strong argument for the impact of environmental factors on myopia development and progression. Associations with myopia include urbanization, decreased time spent outdoors and increasing levels of education. It has also been proposed that some patients may have a genetic susceptibility to the potential myopia-triggering environmental factors and may increase the incidence of myopia progression. Two theories exist about how environmental factors lead to myopia development/progression: Dopamine theory: The increasing time spent indoors decreases reduces the exposure to sunlight and the activation of dopamine receptors in the eye, causing eye growth and the development/progression of myopia Hyperopic defocus theory: myopia development and progression may be due to hyperopic defocus in the mid-peripheral retina. This defocus can be caused by either accommodative lag from near tasks and/or the image of the walls and ceiling of an indoor environment falling behind the elongated retina of a myopic eyeball. This 'mid-peripheral hyperopic defocus' triggers a signalling cascade leading to axial elongation which attempts to place the mid- peripheral back on the retina and resolve blur. However, this elongation leads to myopic defocus at the fovea, requiring a higher myopic prescription, and the cycle continues. MYOPIA MANAGEMENT/CONTROL The management of myopia is no longer as simple as prescribing minus lenses to improve the clarity of vision. An increasing body of literature is helping us understand the aetiology and progression of myopia, so that we can figure out how to stop/slow it. Reducing progression will decrease the burden of disease and the risk of complications of high myopia such as retinal tears and detachment. The current best evidence-based management of myopia involves a discussion with the patient (and their parents) about 'myopia control' options to help slow the growth rate of the eyes. At the moment, there are current four different options that are considered relatively equivalent in effectiveness for slowing progression of myopia, which is on average ~40%. Therefore, a patient is highly encouraged one of the following options: Atropine As a muscarinic antagonist, the mechanism of atropine as an inhibitor of myopia progression is thought to be unrelated to the relaxation of accommodation but instead due to its affects on M4 receptors in the sclera The ATOM-1 study was one of the landmark trials in demonstrating the effect of atropine in slowing myopia progression. Over two years, the study found that when compared to placebo, 1% atropine daily significantly reduced the progression of myopia (−0.28 ± 0.92D vs. −1.20 ± 0.69D) and axial elongation (−0.02 ± 0.35 mm vs. 0.38 ± 0.38 mm) This equivocated to a 77% decrease in myopia progression over 2 years HOWEVER, there were significant size effects of the 1% atropine: photophobia and loss of accomodation, AND in the year following the study there was large rebound axial elongation in the treatment group (−1.14 ± 0.8D compared to −0.38 ± 0.39D in the control group This was followed by the ATOM-2 study, which compared the myopia progression, side effects and rebound myopia of patients treated with 0.5%, 0.1% and 0.01% atropine. The side effects (pupil size and loss of accomodation) and rebound myopia progression (after cessation) were significantly less in the 0.01% group, but so was the effect on slowing myopia progression. After two years of treatment and 1 year of wash out (no treatment), the study concluded that the most effective treatment was the 0.01%. However, it should be noted that there was no placebo group in the ATOM-2 study, AND that the myopia progression in the 0.001% group in this study was not significantly different from the placebo group in the ATOM-1 study The LAMP study was then conducted. It compared atropine 0.05%, 0.025%, 0.01%, and placebo eye drops. Over two years, the progression of myopia was 0.55 ± 0.86D, 0.85 ± 0.73D, and 1.12 ± 0.85D in each treatment group, respectively After the two years, the authors concluded that the 0.05% atropine was double the efficacy of 0.01%, and should be used for myopia control. However, due to the increased side effects and potential rebound myopia from 0.05%, many practitioners use the LAMP study as evidence for 0.025% as a good balance of positive and negative factors So, after years of research we have so far uncovered a seemingly dose-dependent response between atropine % and myopia progression, ocular side effects and rebound progression. As such, it is now recommended that the % chosen is a balance between these elements, mainly 0.05% or 0.025%. There are still many unknowns with atropine treatment - mostly revolving around the optional dosing, and in particular, how long patients should be on the drops for? ie. is it until they are in their mid-20s? Because that is a loooooong time. Ortho K Orthokeratology is a specialised form of hard contact lens correction for myopia (and less commonly hyperopia) These lenses are designed the multiple curves that create a negative pressure gradient in areas under the lens when it is worn overnight. This pressure gradient moulds the corneal surface by thickening the epithelium in the mid-peripheral and thinning centrally. This leads to the correction of myopic refractive error at the macula and correction of the hyperopic defocus at the mid-peripheral retina. The ROMIO and HM-PRO studies evaluated the myopia progression in Ortho-K lenses vs. control and found a reduction in axial length of 43% to 63%, although both studies had high drop out rates, likely due to the nature of the treatment ie. hard contact lenses are tricky and uncomfortable The long-term efficacy of Ortho-K is not yet known and there is the potential for significant rebound myopia progression following the cessation of Ortho-K treatment Multifocal (MF) Soft Contact Lenses (CLs) Multifocal soft contact lenses use the same mechanism as Ortho-K in that they aim to correct peripheral hyperopic defocus, which is a stimulus for axial elongation These lenses are 'distance-centred' MF CLs, so distance vision is corrected in the middle of the lens for clarity at the fovea, and plus power is added to the periphery of the lens to correct for mid-peripheral hyperopic defocus on the retina Over three year, MiSight lenses by Coopervision have demonstrated a 59% and 52% reduction in myopia progression in diopters and axial length, respectively, compared to placebo The BLINK study found that the greater the peripheral addition power, the greater the effect on myopia control. However, a higher addition power may impact visual function during wear. DIMS/Stellest Lenses DIMS spectacles lenses contain small lenslets in the mid-periphery of the lens (surrounding a central 9mm optical zone), which bends incoming light in front of the retina to help reduce the amount of hyperopic defocus. Over two years, myopia progression with DIMS lenses was −0.41 ± 0.06 D compared to −0.85 ± 0.08 D in single-vision spectacles Stellest lenses are use a similar technology called 'Highly Aspheric Lenslet Technology', This technology was found to reduce myopia progression in diopters by 55% and axial length by 50% over two years Either of these lenses are options for full time wear to control myopia, and may be combined with atropine therapy for further control if progression is still too significant. Things that are not acceptable myopia control options: Given the amount of more recent evidence about the efficacy of the above four options for myopia control, the following options are officially not an acceptable management strategy for myopia progression PALs Progressive addition lenses aim to reduce peripheral hyperopic defocus during near work by providing a reading add in the bottom of myopic lenses. Results from the COMET study determined that although PALs significantly slowed the myopia progression by 0.20±08 D (p = 0.004) over three years, this was not clinically significant enough to change current prescribing practices. Undercorrection There has only been one masked, randomised clinical trial studying the effects of undercorrection of myopia on its progression. Results of the two-year study revealed 0.77D of progression in the fully corrected group. This was significantly less than the 1.00 D of progression seen in the 0.75D undercorrected group (p < 0.01). These results differed from animal studies in that myopic defocus increases myopia progression rather than slowing it. This may be due to an abnormal detection of the direction of retinal image defocus. Part-time wear Data from a three-year preliminary study found that refractive shifts were not significantly different between full-time wearers, patients who changed from distance to full-time wear, distance wearers, and nonwearers. In summary, myopia development and progression is complex, multifactorial and increasingly more common. Greater evidence is required to determine the optimal management strategy for these patients to prevent progression to high myopia and increasing the risk of the associated adverse events. MYOPIA CORRECTION For patients with stable myopia, more traditional correction options are available, such as single vision glasses and contact lenses. Surgical refractive correction may also be considered by some patients. The surgical correction options for refractive error (most commonly myopia) are: PRK LASIK LASEK SMILE Clear lens extraction and replacement For myopia correction, all of these techniques aim to flatten the central cornea to decrease the refractive power and allow the image to fall back on the fovea. In clear lens extraction, the natural lens is removed and a new plastic lens relates it and can be made to any power to perfectly match the axial length of the eye - this procedure is the same as cataract surgery, but without the cataract (see next week). Note: with all refractive surgeries, patients need to be over 18 years of age and have had a stable refraction for at least two years before going ahead. Laser surgery PRK, LASIK and LASEK are all similar procedures involving ablation of the corneal stroma using an excimer laser. PRK is used in thin corneas and the epithelium is abated away along with the stroma - this leads to a more painful recovery due to the large central area of absent epithelium and exposed corneal nerves left after surgery. LASIK and LASEK both utilise a flap of corneal tissue that is temporarily flipped to one side to allow the excimer laser to ablate the storm before the flap is replaced. The thickness of the cornea determines which procedure will be used, with LASIK requiring a thicker cornea. SMILE is a less invasive form of LASIK where no flap is required. A lenticule is formed within the stroma using a femtosecond laser , this is then removed through a small incision in the stroma, flattening the cornea to the desired curvature. The advantages of laser surgery is excellent unaided distance vision without glasses or contact lenses. Due to the severing of corneal nerves during the procedure, dry eye is an almost guaranteed side effect, and patients with already dry eyes should proceed with caution. Due to the change in corneal curvature that occurs at the edge of the treatment zone in the mid-peripheral cornea, patients may have increased glare in dim lighting. Clear lens extraction Clear lens extraction and replacement is the same as cataract surgery, before a cataract develops. It is a more invasive surgery and for high myopes has an increased risk of retinal tears, holes and detachments. However, it may be a good option for high myopes or those with thin corneas when laser surgery is not an option. The advantages of the surgery is that that patient will never need cataract surgery, so it simply brings the inevitable surgery forwards by many years. The main disadvantage of clear lens extraction in a younger patient is the complete loss of accomodation due to the replacement of the natural accomodating lens with an artificial one. Therefore, these patients will require reading glasses after surgery, so it is really just replacing one pair of glasses (ie. distance glasses) for another (reading glasses). Hyperopia In hyperopia the eye is too short or the power is too weak. The light is focussed behind the retina, leading to retinal defocus and blurry distance vision (D). Hyperopia is corrected with convex/converging lenses (plus power), which converge the incoming parallel rays from infinity so that they fall on the retinal plane. However, the retinal defocus also stimulates the accommodation mechanism that can move the image to the retinal plane – this means that many patients with low hyperopia commonly have clear distance vision, but may struggle when the accommodative demand is increased during near work. Even still, many are completely asymptomatic, particularly early in life when they have large accommodative reserves. ACCOMMODATION IN HYPEROPIA The role of accommodation in hyperopia is extremely important and relates to both the diagnosis and management of the condition. The process of accommodation occurs as follows: 1. The retinal blur in hyperopia is detected by the visual cortex leading to the activation of bilateral Edinger-Westphal nuclei in the CNIII oculomotor nuclei in the midbrain. 2. Preganglionic parasympathetic fibres travel with CNIII to the ciliary ganglion and synapse with postganglionic neurons. 3. Postganglionic neurons travel with CNV1 ciliary nerves to the ciliary muscle (note: and pupillary sphincter muscle) 4. Activation of muscarinic receptors by ACh leads to contraction of the ciliary muscle (and pupil sphincter muscle) 5. Helmhotz theory of accommodation: Ciliary muscle contraction causes a forward movement of the muscle, slackening the zonules attached to the lens and allowing the lens to bulge, increasing the anterior surface curvature, thickness and refractive power. 6. This increased power to the lens move the image from behind the eye and onto the retinal plane allowing clear distance vision, and, when required, near vision. However, it is important to note that retinal blur and disparity also activates supraocular motor nuclei that innervate the oculomotor nuclei. These nuclei then send axons to the Medial Longitudinal Fasiculus, leading to the contraction of the medial rectus muscles by CNIII and convergence of the eyes when accommodating. This is helpful when normally accommodating for near tasks, but not so great when trying to overcome hyperopia to make the distance vision clear, because it leads to unwanted convergence for distance objects. TYPES OF HYPEROPIA Hyperopia is like an iceberg. It is made of two components – one you can see, and one you can’t: Manifest hyperopia (visible) Latent hyperopia (invisible) Manifest hyperopia This is the amount of hyperopia ‘visible’ AKA the amount accepted in refraction. It causes blurry distance and near vision due to an inability to accommodate to maintain clarity – this may be due to the level of hyperopia or a dysfunctional accommodative system, or both. Almost all patients with manifest hyperopia will have a latent component as well. Latent hyperopia This is the amount of hyperopia ‘hidden’ by the accommodation system. A person may be a +2.00D hyperope, but will present with perfect 6/6 vision and appear to have no refractive error on subjective refraction because they are accommodating for this hyperopia. Accommodating for latent hyperopia can lead to ocular fatigue (asthenopia), poor concentration, headaches and blurry near vision (and maybe distance). These symptoms may occur when there is high hyperopia or insufficient accommodative reserves, which may be due to age, fatigue or accommodative dysfunction. Due to the relationship between accommodation and convergence, latent hyperopia often produces an esophoria (or esotropia), an inwards turning of the eyes, at distance and near. This can lead to similar symptoms to accommodative fatigue (asthenopia, poor concentration, and headaches) as well as double or blurry vision at distance or near. HYPEROPIA AND AGING Hyperopia often becomes manifest with age due to the natural decline in lens accommodation (presbyopia) which prevents the patient from being able to accommodate for all of their previously latent hyperopia. The effect hyperopia has on the patient depends on the magnitude of the refractive error, the age of the patient, the status of accommodative and convergence system and the demands placed on this system. PATHOPHYSIOLOGY OF HYPEROPIA Unlike myopia that most commonly develops and progresses after emmetropisation in childhood or teenage years, hyperopia is the product of emmetropisation and may stay constant or gradually decrease with age. As previously discussed, the process of emmetropisation occurs primarily in the first year life. This process involves matching the refractive error of the eye with the elongation of axial length caused by eye growth, with the aim of achieving emmetropia (no refractive error). Most people never fully achieve emmetropia and have ~+0.50 of latent hyperopia which is easily overcome by the accommodative system to allow clear vision at distance and near. A dysfunction of the emmetropisation mechanism can lead to a failure of this process, resulting in the patient remaining hyperopic. This is most likely caused by genetic factors that lead to a malfunction of the signalling within the emmetropisation loop, but may also be due to interruptive environmental factors resulting in inappropriate input to the loop. Image from: Adler's Physiology of the Eye, 2011 CONSEQUENCES OF UNCORRECTED HYPEROPIA Many hyperopes may go through life completely asymptomatic – this is most often beneficial, but can sometimes lead to negative consequences. Hyperopia is present from birth, and children with high hyperopia may not accommodate correctly or only accommodate enough for one eye (in eyes with different refractive error – anisometropia). This leads to blurred vision in one or both eyes. As the visual system is developing during this time and forming the connections between the retina and the visual cortex, it is crucial that both eyes have clear distance vision to allow equal development of the visual pathway for each eye as well as binocularly driven neurons to facilitate effective single binocular vision. Failure of these connections to develop in one or both eyes leads to amblyopia – a loss of vision in a healthy eye due to an interruption in the development of the visual pathway. The excessive accommodation required to overcome high hyperopia may lead to excessive convergence. This results in an esophoria that is greater at near due to the higher demand on accommodation. Esophoria places strain on the visual system as it attempts to diverge the eyes to maintain single binocular vision. An esophoria can make focusing very difficult, and may lead to an aversion to reading or difficulty concentrating on near tasks and can result in learning difficulties in young children. A high esophoria can lead to a breakdown of binocular fusion and cause an esotropia. This is when one eye turns inwards, causing the image to fall on different locations in the retina of the two eyes, leading to diplopia (double vision). Children can easily adapt to diplopia by suppressing the image from the deviated eye. If the esotropic is consistently the same (esotropias can be monocular or alternating), the visual input from the deviated eye to the brain will be continually suppressed. This can disrupt the development of the visual pathway in the esotropic eye, leading to amyblopia. MANAGEMENT OF HYPEROPIA The management of hyperopia is complex due to the involvement of the accommodative system and the concept of latent hyperopia. Cycloplegic drugs can be used block the muscarinic receptors on the ciliary muscle and prevent accommodation – this reveals the full level of hyperopia (the whole iceberg) and is particularly important for children as they can mask a sizeable amount of refractive error with their large accommodative reserves. The refractive correction of hyperopia is often driven by symptoms. For example: an asymptomatic patient may not require any glasses, but simply an education about latent hyperopia and the possible symptoms that may occur and would indicate some correction is required. However, glasses should be considered for high hyperopia in asymptomatic children. Glasses should be prescribed for patients reporting blur or signs or symptoms of binocular dysfunction, strabismus or amblyopia – especially in children where development of clear single binocular vision is of utmost importance. Prescribing glasses for hyperopia The accommodative mechanism complicates prescribing glasses and patients do not often readily accept their latent hyperopia prescription, and very rarely their full cycloplegic prescription. When prescribing for hyperopic patients we need to take a thorough history of when signs/symptoms occur (ie. distance and/or near) and then consider the subjective refraction, retinoscopy results and cycloplegic refraction as well as age of the patient and the state of the accommodative/convergence system. Depending on the patient, their visual symptoms and vision needs they may require: A distance prescription for use mainly for near work but that allows clear distance vision Near glasses (distance prescription with extra plus for near focusing) that will blur any distance viewing A multifocal lens that allows for clear vision at distance and near by including increased plus for near in the inferior portion of the lens (distance prescription may be the latent hyperopia, manifest hyperopia or a mixture between the two – maximum plus) Presbyopia Presbyopia is an irreversible, age-related impairment of near vision that relates to the loss of accommodative function with age. From birth the accommodative power of the lens gradually decreases – from approximately 15D at birth to 1D before 60 years of age. Presbyopia is when this loss of accommodation becomes symptomatic. This is usually at about 40 years of age, but may be earlier or later depending of the refractive state of the patient and their visual tasks. Symptoms of presbyopia include blurry near vision or an increasing working distance as the accommodative reserve of the patient fails to place the image on the retina when objects are moved too close. PATHOPHYSIOLOGY OF PRESBYOPIA The cause of this decrease of accommodation with age is due to two main factors: 1. Increased thickness and decreased pliability of the anterior lenses capsule. This means the capsule fails to move and mould the lens efficiently when the zonules slacken following cillary muscle contraction 2. Increased thickness and decreased elasticity of the lens. As the lens epithelium is constantly undergoing mitosis and differentiation into lens fibres, the lens grows in size and becomes less elastic with age. This means that although the ciliary muscle contracts with the same amount of force, the aging lens fails to achieve an adequate accommodative state, leading to insufficient convergence of the incoming light rays from near objects and the image falling behind the retina, resulting in blurry near vision. Although this process begins at birth it is most noticeable at approximately 40 years of age and continues until about 60 years when the lens loses all accommodative ability and patient rely fully on refractive correction to see clearly at near. Note: this largely depends on the refractive state of the patient and their visual tasks. MANAGEMENT OF PRESBYOPIA The management of presbyopia requires determining the distance prescription and then prescribing a near addition. This depends on the accommodative demand of near tasks (ie. focusing at 40cm = 1/0.4 = 2.5D), the accommodation reserves of the patient and their distance refractive error. There are a number of ways to determine a near add including: Fused Cross-Cyl Age expected value Push-up and Push-down method Accommodative demand – (Accommodation/2) Prescribing for presbyopia The near add can be prescribed in a number of forms including: Reading/computer glasses (blurry for distance) Bifocals lenses where the near add is placed in the inferior part of the lens separated by a segment line Multifocal lenses (distance prescription that progressively increases to the near addition in the inferior section of the lens) Occupational lenses (intermediate addition that progress to near addition, distance is blurry) Monovision and multifocal contact lenses