Level 1 Motors I RACM-155 PDF
Document Details
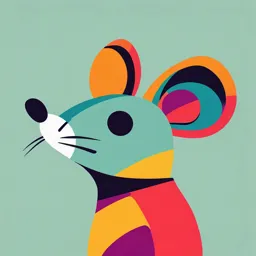
Uploaded by SuperiorSugilite6974
Tags
Summary
This document provides an overview of single-phase motor types, including capacitor-start, split-phase, capacitor-start capacitor-run, and shaded-pole motors.
Full Transcript
 Level 1 ---------- Motors I RACM-155 -- -- **Terminology Associated with Single Phase Motors**: **1. Single-Phase Power** - **Definition**: A type of electrical power in which the current alternates in a single waveform. Single-phase motors are powered b...
 Level 1 ---------- Motors I RACM-155 -- -- **Terminology Associated with Single Phase Motors**: **1. Single-Phase Power** - **Definition**: A type of electrical power in which the current alternates in a single waveform. Single-phase motors are powered by this type of electricity, typically found in residential settings. - **Example**: Standard electricity (120V or 240V) is single-phase. **2. Starting Mechanism** - **Definition**: The method used to start a single-phase motor, which typically requires an auxiliary mechanism to create a rotating magnetic field. - **Types**: - **Capacitor-Start**: Uses a capacitor to provide an extra phase for starting the motor. This increases starting torque. - **Split-Phase**: Uses different windings with different resistance to create the phase shift needed to start the motor. - **Capacitor-Start Capacitor-Run**: Has two capacitors---one for starting and one for running---to improve both starting torque and running efficiency. - **Shaded-Pole**: A simple design with a shaded coil in the pole to create a weak starting torque. **3. Running Capacitor** - **Definition**: A capacitor that remains in the circuit while the motor is running. It helps improve efficiency and performance by creating a phase shift in the motor\'s windings. - **Usage**: Common in **capacitor-run** and **capacitor-start capacitor-run motors**. **4. Starting Capacitor** - **Definition**: A temporary capacitor that provides a phase shift to create a high starting torque, which is disconnected from the circuit once the motor reaches a certain speed. - **Usage**: Typically found in **capacitor-start** and **capacitor-start capacitor-run motors**. **5. Induction Motor** - **Definition**: A type of electric motor where the electric current needed to produce torque is induced by electromagnetic induction. This is the most common type of single-phase motor. **6. Stator** - **Definition**: The stationary part of the motor that creates the magnetic field. In single-phase motors, the stator is energized by the alternating current (AC) to generate the magnetic field. - **Purpose**: The stator\'s magnetic field interacts with the rotor to produce motion. **7. Rotor** - **Definition**: The rotating part of the motor that is subjected to the magnetic field created by the stator. - **Types**: - **Squirrel-Cage Rotor**: Most commonly used rotor type in single-phase induction motors, characterized by its durable, simple construction. - **Wound Rotor**: Used in some single-phase motors, consisting of windings connected to external resistance or other components for speed control. **8. Run Load Amps (RLA)** - **Definition**: refers to the current a motor draws when it is operating under normal, steady-state conditions, typically when the motor is running at a load that is lower than its full-load condition. - **Significance**: Sizing overloads, motor maintenance. **8. Locked Rotor Amps (LRA)** - **Definition**: The current drawn by the motor when it is not rotating (i.e., during startup). - **Significance**: The locked rotor current is typically much higher than the normal running current and should be considered when sizing circuit breakers and fuses. This is due to the lack of back emf being generated. **9. Full Load amps (FLA)** - **Definition**: The current that the motor draws when operating at its rated power (under normal conditions and full load). - **Significance**: This is important for sizing electrical components such as circuit breakers and wiring to handle the motor\'s running current **10. Service Factor** - **Definition**: The service factor of an electric motor is a multiplier that indicates how much over its rated capacity a motor can be safely operated without causing damage or reducing its lifespan. It can be thought of as reserved horsepower. - **Significance:** This is needed when sizing overloads for motors. Typically, the service factors range from 1 to 1.35. This means that the motor can operate at 100-135 % of its rated amp draw. **Single Phase Motor Operation** - **Concept**: A single-phase motor operates on the principle of electromagnetic induction and relies on the interaction between the magnetic field generated by the stator and the current flowing through the rotor to produce mechanical motion. - **Stator Field:** The stator (the stationary part of the motor) is energized by a single-phase AC supply, creating a magnetic field that alternates as the current changes direction. This alternating magnetic field causes the rotor (the rotating part of the motor) to begin to turn. This creates back emf, adding the needed resistance to complete the circuit. Without this, the motor would draw it's LRA. - **Considerations:** there\'s a challenge with single-phase motors: the magnetic field produced by the stator is not rotating, unlike in three-phase motors where the current creates a rotating magnetic field. This results in a starting issue---without an initial rotating field, a single-phase motor won\'t start by itself. **Voltage Changes and Motor Operation** - **Voltage Range:** The range of voltages on most motors is +/- 10 %. This means that on a 208V motor, the lower limit would be 187V, with the upper limit being 229V. On a 208/230V motor, the lower limit is still 187V, but the upper limit is now increased to 253V. - **Increased Voltage:** Increasing the voltage to a motor can actually increase its rated HP, however the windings will develop hot spots. The motor will eventually overheat, and can actually burn out if it continually runs like this. This is considering operating overloaded, although we usually do not see an increase in current. - **Decreased Voltage:** Decreasing the voltage to a motor will decrease the HP, not giving the motor enough fuel to do the work. This leads to an increase in current, and can cause the motor to trip its overload. If the motor operates in this overloaded condition continuously, it will overheat and eventually burn out. - **Increased Load:** Increasing the load on the majority of motors increases the current draw. This comes from an increase in torque needed to achieve its rated RPM. This causes the motor to operate at a higher temperature, decreasing its efficiency. - **Decreased Load:** Decreasing the load on majority of motors will lower the current draw. This comes from the decrease in torque needed to achieve its rated RPM. This also allows the motor to run slightly cooler. **Types of Motors** - **Description:** Induction motors are the most commonly used motors in HVAC systems. They are robust, simple, and cost-effective. The rotor is not powered directly; instead, it induces a current through electromagnetic induction from the stator. - **Application:** Used in a wide variety of HVAC components, including fans, blowers, compressors, and pumps. - **Advantages:** - Reliable and low maintenance. - Cost-effective. - Commonly available in both single-phase and three-phase configurations. - **Disadvantages:** - Less efficient compared to other motor types (like permanent split capacitor or ECM motors). - Not ideal for applications that require variable speed. **Capacitor Start Induction Run (CSIR)** **Capacitor-Start Motor Overview:** - A capacitor-start motor is essentially a split-phase motor with an added start capacitor. This capacitor is connected in series with the start winding, and it significantly improves the motor\'s starting torque compared to a typical split-phase motor. - The capacitor's primary function is to create a phase shift between the current and voltage, improving the torque when the motor starts. As you correctly mentioned, in an inductive circuit (like the main winding of the motor), the current lags the voltage, while in a capacitive circuit (like the start winding), the current leads the voltage. The phase angle that results from this difference is crucial for maximizing the torque during startup. **Starting Process:** 1. **Start Winding and Capacitor:** When the motor is energized, the start winding, along with the capacitor, provides the initial boost needed to get the motor spinning. The capacitor creates a phase shift between the current and voltage, which helps generate the necessary starting torque. This is particularly important in applications requiring high starting torque, such as compressors or fans. 2. **Motor Starts:** Once the motor reaches a certain speed, typically around 70-80% of full speed, the capacitor and the start winding are disconnected from the circuit. This is done by a centrifugal switch or an electronic relay. Once the capacitor is removed, the motor operates just like a standard induction motor, with only the run winding engaged. 3. **Continued Operation**: After the start winding and capacitor are out of the circuit, the motor continues to run with just the run winding energized. The rotor's motion helps maintain the imbalance in the magnetic field, which keeps the motor turning. This is why the motor is often referred to as a CSIR motor --- Capacitor-Start, Induction-Run. **Key Features of Capacitor-Start Motors:** - **Starting Torque:** The capacitor provides more starting torque than a split-phase motor without a capacitor. This makes the capacitor-start motor suitable for applications that require higher initial torque. - **Efficient Starting:** The phase shift created by the capacitor ensures that the motor starts more efficiently and can overcome the initial resistance of the load (like fans or compressors). - **No Use of Capacitor During Running**: The capacitor is removed from the circuit once the motor reaches the appropriate speed. This is because it's not needed for continuous operation, and running the motor with the capacitor in the circuit could result in inefficient operation or potential damage to the capacitor. **Advantages:** - **Higher Starting Torque:** The addition of the capacitor helps produce significantly higher starting torque compared to a regular split-phase motor. - **Simple Design:** Like the split-phase motor, the capacitor-start motor has a relatively simple design, with fewer components than more complex motors like capacitor-run motors or electronically commutated motors (ECM). **Applications:** Capacitor-start motors are commonly used in applications requiring high starting torque, such as: - **Compressors (especially in HVAC systems).** - **Pumps.** - **Fans and blowers.** - **Air conditioning units.**  **Capacitor-Start, Capacitor-Run Motor (CSCR):** - **Motor Design:** - Similar to a split-phase motor with both start and run capacitors. - Run capacitor stays in the circuit during running; start capacitor is only used for startup. - **Capacitors and Windings:** - Capacitors wired in parallel, both in series with the start winding. - Start capacitor provides a higher microfarad rating than the run capacitor. - Capacitance adds up (start + run capacitors) to give higher starting torque. - **Starting Process:** - Both capacitors are in the circuit during start-up, creating greater phase shift and more starting torque. - After reaching speed, the start capacitor is disconnected from the circuit. - The run capacitor stays in the circuit, aiding continued running and limiting current through the start winding**.** - **Running Process:** - Acts like a Permanent Split Capacitor (PSC) motor after startup. - If the run capacitor fails, the motor still starts, but running amperage increases (about 10%) and causes overheating at full load. - **Efficiency:** - Highly efficient for both start-up and continuous running. - Common in refrigeration and air conditioning systems. - **Applications:** - Used in belt-driven fans, compressors, and HVAC systems where high starting torque is needed. - **Advantages:** - High starting torque. - Efficient operation. - Reduced current in start winding during running, preventing overheating. - **Issues:** - If the run capacitor fails, the motor will overheat when running at full load. **PSC Motor (Permanent Split Capacitor Motor):** - **Motor Design**: - Similar to the **split-phase motor**, but does **not** have a start capacitor. - Uses **one run capacitor** wired in the circuit, connected to the **L2 sides of the start and run windings**. - No **start switch** is required since both the start and run windings, along with the run capacitor, are always energized when the motor is on. - This makes it the **simplest** type of split-phase motor. - **Efficiency and Operation**: - **Efficient** operation with no moving parts needed for startup. - **Low starting torque**, making it suitable only for **low-torque applications**. - **Multispeed PSC Motor**: - Identified by multiple wires at the motor electrical connections. - Speed is controlled by adding or removing **resistance** from the circuit. - Used in **HVAC** systems, especially in **fan sections**. - **Speed adjustment** can be done by **switching the wiring** or varying resistance. - **Speed and Pole Configurations**: - Available in **2-, 4-, 6-, and 8-pole designs**. - Motor speed is determined by the number of poles and the frequency of the AC source. - **For 60 Hz operation**: - 2-pole: 3600 rpm (synchronous), actual 3450 rpm - 4-pole: 1800 rpm (synchronous), actual 1725 rpm - 6-pole: 1200 rpm (synchronous), actual 1075 rpm - 8-pole: 900 rpm (synchronous), actual 825 rpm - **Application in HVAC**: - Often used for **slow fan speeds** during **winter heating** (providing higher leaving-air temperatures for furnaces). - Speed can be **increased in summer** to provide more airflow for cooling. - **Advantages**: - **Soft start-up** with gradual acceleration, preventing **start-up noise** (ideal for fan applications near return air inlet). - **More energy-efficient** and quieter than **split-phase motors** in fan applications. - **Disadvantages**: - **Low starting torque**, making it unsuitable for high-torque applications. **Shaded-Pole Motor:** - **Design and Operation**: - Uses **shading coils** on each pole to help the motor start by creating an **induced current** and a **rotating magnetic field**. - The **location of the shading coil** determines the direction of rotation. - **Rotation Reversal**: - **Rotation can be reversed** by **disassembling the motor** and turning the **stator** over. This moves the shading coil to the opposite side of the pole face, reversing the direction. - **Applications**: - Commonly used in **air-cooled condensers** and **evaporators** to turn the **fans**. - Suitable for **light-duty applications** due to low starting torque. - **Efficiency and Performance**: - Has **very low starting torque** and is **less efficient** compared to other motors like the **PSC motor**. - Best for **small, low-power applications** (typically in the **fractional horsepower range**). **Three-Phase Motor Summary:** - **Power and Efficiency**: - Ranges from about **1 hp** to **thousands of horsepower**. - **Very efficient** and suitable for high-torque applications. - **Starting**: - **No start assist** required (no starting windings or capacitors). - High **starting torque** due to the three-phase current, making it easy to start large loads like **fans** and **compressors**. - **Construction**: - Comprised of **three single-phase power supplies**, each with either **two or four poles**. - For example: - **3600 rpm motor**: 3 sets of 2 poles (total 6). - **1800 rpm motor**: 3 sets of 4 poles (total 12). - **Rotation and Speed**: - The motor operates with **three phases** that are **120 degrees out of phase** with each other. - At any point in the rotation, one winding is positioned for high torque. - **Slip** causes the motor\'s actual rpm to be slightly lower than the synchronous speed: - **3600 rpm motor**: Slips to about **3450 rpm** under load. - **1800 rpm motor**: Slips to about **1750 rpm** under load. - **Power Supply**: - Three-phase power consists of three single-phase supplies, each **120 degrees apart** in phase, creating a continuous, balanced current flow. - **Motor Rotation**: - Rotation can be changed by **switching any two motor leads**. - This is crucial for fan applications, as **incorrect rotation** will cause the fan to move less air. Reversing the leads will correct the direction. - **Availability**: - Typically **either 1800 rpm or 3600 rpm**---not available with dual speed.  **Single Phase Open Motors:** **Power Supply for Single-Phase Motors:** - Common supply voltages: 115 V, 208 V, 230 V. - Home furnace: Uses 115 V. - Outdoor air conditioner: Uses 230 V. - Commercial buildings: May use 208 V, 230 V, or 460 V, depending on the power company. **Dual-Voltage Single-Phase Motors:** - **Equipped with:** - Two run windings: Same resistance. - One start winding: High resistance. - **Operates in two modes:** - Low-voltage mode: Run windings connected in parallel. - High-voltage mode: Run windings connected in series (effective voltage = 115 V per winding). - Windings always operate on 115 V, regardless of the mode. - Wiring changes for voltage mode adjustments are made at the motor terminal box. **Commercial/Industrial Installations:** - Larger motors: May use a 460-V power supply. - Smaller motors: Operate on reduced voltage (single-phase) from the same power source. **Motor Direction of Rotation:** - Can rotate **clockwise** or **counterclockwise.** - Direction is reversible by: - Reversing the **start** winding leads. - Changing the current flow direction, which alters the pole charges. - Adjustments made at the motor terminal box.  **ECM (Electronically Commutated Motors):** - Used for open-drive fans (1 hp or smaller). - Reliable, energy-efficient, and cost-effective over time. - Combines DC motor efficiency with electronic commutation, eliminating brushes. - Armature magnetized with permanent magnets and adhesives. **Factory Calibration:** - **Pre-programmed for:** - Speed, torque, airflow, and static pressure. - Adapts airflow to field conditions (e.g., restricted filters or closed registers). **Applications:** - **Used in:** - Gas furnaces, air conditioners, oil burners, heat pumps. - Refrigeration systems for variable loads. **Motor Components and Troubleshooting:** - **Two-piece design:** - Control section and motor section. - Controls can be replaced independently. - **Troubleshooting:** - Use an ohmmeter or manufacturer's test module. - Control packages are non-interchangeable and must match the equipment. **Variable-Speed Motors:** - **Benefits of variable-speed motors:** - Soft start reduces inrush current. - Minimizes voltage drops in large systems. - Reduces power fluctuations (spikes/valleys) using filters. - **Controlled speeds:** - Adjust fan speeds to match compressor load. - Ensures proper humidity control and pressure stabilization. **Advantages of Variable-Speed Technology:** - Energy savings and load reduction. - Soft motor startup (avoids Locked Rotor Amperage). - Enhanced temperature and humidity control. - Solid-state motor starters (no open contacts). - Supports future expansion with part-load operation. - Matches load and capacity efficiently**.** **Additional Notes:** - Power fluctuations are filtered to prevent electronic interference. - Always check the incoming power supply before troubleshooting. - Follow the manufacturer's procedures for maintenance and diagnostics. **\ ** **Detailed Summary of Inverter-Controlled and Variable Frequency Drives (VFDs)** **1. Role of Inverters in Motor Speed Control** - Inverters adjust motor speed by controlling the frequency of the power supply. - Traditional motors operate at a constant 60 Hz, with speed determined by the number of poles. - Variable-speed motors use inverters to: - Decrease speed to about 10% of the rated speed. - Increase speed up to 120% of the rated speed by varying frequency beyond the 60 Hz standard. **2. Types of Inverters** - **Six-Step Inverter:** - Uses six switching components (two per phase for three-phase motors). - Two variations: 1. Voltage-Controlled: Stabilizes voltage using a large capacitor on the DC bus output. 2. Current-Controlled: Stabilizes current flow using a choke (large coil) on the DC bus output**.** - **Pulse-Width Modulator (PWM) Inverter:** - Provides a fixed DC voltage input and modulates it into pulses for the motor. - Pulse length varies with speed: 1. Short pulses for low speeds. 2. Long pulses for high speeds. - Produces sine-coded pulses that resemble a sine wave, enabling precise motor speed control. - **Efficiency Gains:** - Motors with VFDs are 65%-75% more efficient than constant-speed motors at line voltage. - Payback period for retrofitting HVAC systems with VFDs: 1--2 years. - Life cycles of HVAC systems: 15--20 years, making VFDs a cost-effective solution. **3. Benefits of VFDs in HVAC Systems** - **Energy Savings:** - Matches airflows to system demands with Variable Air Volume (VAV) systems. - Operates motors at reduced capacities most of the time, saving energy and money. - **Comfort and Noise Control:** - Regulates air and water flows for closer temperature and humidity control. - Reduces noise levels compared to constant-speed systems. - **Soft Start Feature:** - Gradually ramps motor speed to match demand. - Eliminates inrush current (LRA), reducing voltage dips and prolonging motor life. - **Reduced Maintenance:** - Eliminates motor short cycling and minimizes starting shock. - Extends the life of motors and connected equipment. **4. Applications of VFDs** - **Commonly used for:** - Evaporator and condenser fans. - Compressor motors. - Centrifugal pumps for chilled and hot water systems. - **Additional motor applications include:** - Cooling tower fans and water pumps. - Make-up air fans. - Exhaust fans. - Booster fans. - Air handler fans. **5. Technical Insights** - **Synchronous Speed:** - Motor speed (rpm) is determined by: - Frequency (Hz). - Number of poles in the motor. - Higher frequency or fewer poles increase motor speed. - Lower frequency or more poles decrease motor speed. - **Significance of VFDs:** - Frequency (Hz) adjustment via VFDs is simpler than altering the number of poles. - Essential for achieving precise speed control. **6. Environmental and Operational Benefits** - Integration of VFDs supports newer high-efficiency refrigerants like R-410A. - Enhances system efficiency while reducing energy consumption and operational costs. - Provides longer equipment lifespan and ensures optimized performance tailored to varying loads. **Starting Components for Single Phase Motors:** **Potential Relay:** **1. Purpose and Application** - Function: Potential relays assist in starting single-phase, capacitor-start, capacitor-run motors that require high starting torque. - Common Names: Also referred to as voltage relays or potential magnetic relays (PMRs). - Applications: Used in motors that power systems like compressors and require precise starting conditions. **2. Components and Configuration** - Key Components: - High-Resistance Coil: Wired between terminals 2 and 5. - Normally Closed Contacts: Wired between terminals 1 and 2. - Dummy Terminals: Used for additional connections, such as for condenser fans or capacitors. - **Capacitor and Winding Configuration:** - The start winding is connected in series with both the start and run capacitors. - The capacitors are in parallel with each other but collectively in series with the start winding. - Capacitance Effect: Parallel capacitors combine capacitances, providing high starting torque. **3. Operating Mechanism** - **Starting Phase:** - When power is applied via the compressor\'s contactor, the run and start windings are energized. - The motor rotor begins turning, generating back electromotive force (BEMF). - **Relay Action:** - As the motor accelerates, the induced BEMF increases. - When BEMF reaches the pick-up voltage, the relay coil generates enough magnetism to open the normally closed contacts (1 and 2), removing the start capacitor from the circuit. - **Coasting or Slowing Down:** - If the motor slows down (e.g., due to a load increase), BEMF drops below the drop-out voltage. - The contacts close again, reconnecting the start capacitor to provide additional torque and bring the motor back up to speed. **4. Key Terms** - Pick-Up Voltage: The voltage at which the relay's contacts open to disconnect the start capacitor. - Drop-Out Voltage: The voltage at which the contacts close to reconnect the start capacitor when the motor slows down. **5. Sizing and Selection** - **Why Proper Sizing is Critical:** - Each motor and compressor generate different BEMF levels, so the relay must match the motor's specific requirements. - **Factors for Sizing a Relay:** - Pick-Up Voltage: Voltage required to open the contacts**.** - Current-Carrying Capacity: The relay contacts' ability to handle current during operation. - Drop-Out Voltage: Voltage level at which contacts close to reconnect the start capacitor. - Continuous Coil Voltage: Voltage the relay coil can handle over extended operation. - **How to Select the Correct Relay:** - Consult service manuals, supply houses, or the compressor manufacturer for proper specifications**.** **6. Advantages of Potential Relays** - **Enhanced Torque Control:** - Provides additional starting torque during motor slowdowns by re-engaging the start winding when needed. - **Motor Protection and Efficiency:** - Ensures proper starting and running conditions by disconnecting the start capacitor at the correct speed. **Current Relays** **1. Purpose and Application** - **Function**: Current relays assist in starting single-phase fractional horsepower motors that require low starting torque. - **Common Names**: Also known as magnetic current relays or simply current relays. - **Usage:** Primarily found in motors with split-phase designs. **2. Components and Configuration** - **Relay Components:** - **Coil:** Surrounds an iron core and generates a magnetic field when current flows. - **Contacts:** Normally open (between terminals L and S) until closed by magnetic force. - **Spring/Gravity Mechanism:** Returns the contacts to the open position when magnetic force decreases. - **Wiring Configuration:** - The relay coil is in **series** with the run winding of the motor. - The start winding is energized when the contacts between L (line) and S (start) close. **3. Operating Mechanism** - **Starting Phase:** - When the motor is powered, the locked-rotor amperage (LRA) flows through the relay coil. - **High current (LRA) generates a strong magnetic field around the relay coil, which magnetizes the iron core.** - This magnetic field closes the contacts between L and S, energizing the start winding and initiating rotor movement. - **Running Phase:** - As the motor accelerates, **back electromotive force** (BEMF or back voltage) is generated in the run winding. - **BEMF** reduces the current draw through the run winding and relay coil to full load amps (FLA) or rated load amps (RLA). - The reduced current weakens the magnetic field, allowing spring pressure or gravity to open the contacts between L and S. - This disconnects the start winding, leaving the motor to operate on the run winding alone. - **Stopping Phase:** - When the motor is powered down, it coasts to a stop. - The relay contacts remain in their normally open position, ready for the next cycle. **4. Key Terms** - **Locked-Rotor Amperage (LRA):** The high initial current draw when the motor starts, causing the relay to activate. - **Full Load Amps (FLA)/Rated Load Amps (RLA):** The steady-state current drawn by the motor during normal operation. - **Back Electromotive Force (BEMF):** Voltage induced in the motor windings as the rotor turns, reducing current draw. **5. Applications and Benefits** - **Applications:** - Used in motors with split-phase designs requiring low starting torque. - Common in systems where the pressure equalizes during the off cycle, such as those with: - Capillary tube metering devices - Fixed-bore orifice metering devices - **Advantages:** - Simple and reliable operation. - Automatically adjusts to motor conditions by using the current draw as the control signal. - Reduces wear on the start winding by disconnecting it once the motor is running at normal speed. **6. Limitations** - **Low Starting Torque:** Current relays are unsuitable for motors requiring high starting torque or where starting under high-load conditions is common. - **Application-Specific:** Best suited for compressors or motors where pressure equalization occurs during the off cycle, reducing the need for high torque during startup.  **\ ** **Positive Temperature Coefficient Resistors (PTCRs):** **1. Definition and Function** - **PTCR Overview:** - Variable resistors whose resistance increases as temperature rises and decreases as temperature falls. - Operates as a compressor starting device. - Made from highly pure semiconducting ceramic material. - **Temperature Behavior:** - At room temperature, resistance is low, allowing significant current flow. - At a certain high temperature, resistance spikes, acting like an open circuit and stopping current flow. - When cooled, resistance returns to initial levels. **2. Applications** - **Replaces Relays**: Commonly used instead of **current** or **potential** relays. - **Industries and Systems:** - Ice machines. - Air-conditioning and heat pump systems with orifice metering devices or TXVs (with bleed ports for pressure equalization). - **Advantages Over Relays:** - Simplifies wiring. - Eliminates moving parts like contacts, coils, and springs, reducing maintenance. **3. Wiring and Operation** - **Wiring Configuration:** - PTCR is wired in **parallel** with the **run** capacitor**.** - PTCR is also in **series** with the **start** winding in permanent split capacitance **(PSC) motors.** - **Startup Process:** - **Initial Inrush Current:** - Both the run and start windings experience high current when power is applied. - The PTCR, at room temperature, has low resistance, allowing increased current in the start winding. - This creates high starting torque. - **PTCR Response:** - High current flow heats the PTCR, quickly raising its resistance. - Within **1 second**, the PTCR acts as an open circuit, effectively removing itself from the circuit. - **Motor Operation:** - The motor continues to operate as a PSC motor using the run capacitor for the remainder of the cycle. **4. Key Considerations** - **Cool-Down Requirement:** - The PTCR must cool down to near ambient temperature before the next startup. - If the PTCR remains hot, its high resistance can prevent effective motor startup. - **Challenges:** - **Short Cycling or Overload:** Can leave the PTCR too hot for proper operation. - **Low Voltage:** During startup, insufficient voltage can reduce starting torque, causing the motor to stall. **5. Benefits** - **Simplifies Design:** - Reduces reliance on complex relay systems**.** - **Improves Durability:** - No moving parts mean less wear and tear. - **Enhanced Reliability:** - Especially suitable for systems with equalized pressures during off cycles, ensuring easier starts. **Checking Motor Windings:** - **Single Phase Motors:\ ** - **Start to Common --** the start winding will have a higher resistance to **common** than the run winding, it is needed to generate enough current to start the motor. - **Run to Common --** the run winding will have a lower resistance to **common** than the start winding. - **Start to Run --** measuring start to run will give you the highest resistance reading compared to the other checks. - **If you know two, you can get the third** - If you take the resistance reading of start to common, and run to common, add them together, it will equal the value of start to run. - Ex. S to C = **2.5 ohms**. R to C = **2 ohms.** What would you expect S to R to be? S to R = **4.5 ohms**. - Ex. S to R is **1.8 ohms**. R to C is **0.8 ohms**. What would you expect S to C to be? S to C = **1 ohm.** - **Three Phase Motors:** - **Three Phase Motors --** Three phase motor windings are **all equal.** Typically, the windings are labelled U, V, W. - **Ex.** U to V **= 1.8 ohms.** U to W **= 1.8 ohms.** V to W **= 1.8 ohms.** **\ ** **Effects of Motor Pulley Selection, Adjustment, and Alignment on Electric Motors** **1. Pulley Selection** **The type, size, and material of a motor pulley significantly affect the motor\'s performance and system efficiency.** - **Pulley Size:** - **Larger Pulley on Motor:** - Increases the driven equipment speed. - May result in higher current draw, increased motor load, and reduced motor lifespan if the motor is undersized for the load. - **Smaller Pulley on Motor:** - Decreases the driven equipment speed. - Reduces motor load and current draw but may result in insufficient equipment performance if speed is too low. - **Pulley diameter affects belt tension and must match the desired speed ratio between motor and driven equipment.** - **Pulley Type:** - **Fixed Pulley:** - Provides a constant speed ratio. - Simpler setup but limited adjustability. - **Adjustable Pulley:** - Allows speed adjustments for specific applications. - Offers flexibility but requires careful calibration to avoid misalignment or belt slipping. - **Material:** - Lighter materials (e.g., aluminum) reduce rotational inertia, improving motor efficiency. - Heavier materials (e.g., steel) are more durable for high-torque applications but may place more strain on the motor. **2. Pulley Adjustment** **Proper adjustment of the pulley impacts the motor's operation, efficiency, and reliability.** - **Belt Tension:** - **Too Tight:** - Increases bearing and shaft wear. - Causes excessive strain on the motor, leading to overheating or premature failure. - **Too Loose:** - Causes belt slippage, reducing torque transfer and system efficiency. - Increases wear on both the belt and pulley, resulting in frequent maintenance. - **Pulley Position:** - Adjustments that shift the pulley along the motor shaft can help achieve the correct belt alignment, minimizing wear and ensuring smooth power transmission. - **Speed Adjustment (with Variable Pulley):** - Allows for fine-tuning motor-driven equipment speed without replacing components. - Incorrect adjustments can lead to speed mismatches, inefficiency, or overloading the motor. **3. Pulley Alignment** **Alignment is critical for minimizing wear, vibration, and noise in motor-driven systems.** - **Proper Alignment:** - Ensures that the motor pulley and driven pulley are parallel and their grooves are aligned. - Prevents belt wear, excessive vibration, and stress on motor bearings. - **Misalignment Types:** - **Angular Misalignment:** - Occurs when pulleys are not parallel. - Leads to uneven belt wear, vibration, and inefficient power transfer. - **Offset Misalignment:** - Happens when pulley grooves are not aligned. - Causes belt tracking issues, slippage, and increased noise**.** - **Combination Misalignment:** - A mix of angular and offset misalignment, exacerbating mechanical problems. - **Effects of Misalignment:** - Reduced energy efficiency due to friction and slippage. - Increased motor wear, including bearing and shaft damage. - Higher operating noise and vibration, potentially affecting the entire system**.** **4. Practical Implications** - **Efficiency:** - Proper selection, adjustment, and alignment ensure optimal power transfer and reduced energy loss. - **Motor Longevity:** - Reduces mechanical stress on the motor and associated components. - **Maintenance Costs:** - Poor practices increase wear, leading to frequent repairs or replacements. - **Performance:** - Proper setup ensures the driven equipment performs at the desired speed and torque levels. **5. Recommendations** - Select pulleys with appropriate size, type, and material for the specific motor and application. - Regularly check and adjust belt tension to prevent slippage or excessive wear. - Use alignment tools (e.g., laser alignment or straight edges) to ensure accurate pulley alignment. - Inspect pulleys and belts periodically for wear or damage and replace them as needed to maintain system efficiency and reliability. **Run vs Start Capacitors:** Run capacitors can usually be identified by being a in metal shell vs plastic for start caps.  **Steps for Testing a Capacitor Under Load** **1. Check the Capacitor Data Tag** - Look at the capacitor\'s label to determine its rated capacitance (in microfarads, MFD) and the tolerance (e.g., ±5%). - Note these values for comparison after performing the test. **2. Measure Start Winding Amps** - Using an **amp clamp**, measure the current flowing through the start winding. - Place the clamp around the wire connecting the capacitor to the start terminal of the motor. **3. Measure Voltage Across the Capacitor** - Use a multimeter to measure the voltage applied across the capacitor's terminals. - Ensure the voltage is steady and accurately recorded. **4. Perform the Capacitance Calculation** - Use the formula: [\$\\text{Capacitance}\\left( \\text{MFD} \\right) = Start\\ Winding\\ Amps\\ x\\frac{2652}{\\text{Voltage}}\$]{.math.inline} - Multiply the measured **start winding amps** by **2652**, then divide by the measured voltage. **5. Compare the Results to the Capacitor's Rated Capacitance** - Check if the calculated capacitance falls within the tolerance range specified on the capacitor\'s label. **Example Calculation** - Rated capacitance: **50 µF ± 5%** - Measured start winding amps: **6 A** - Measured voltage across the capacitor: **240 V** **Check Tolerance** - Tolerance range for 50 µF ± 5%: - 50uf x 0.05 = 2.5uF. - Range = 47.5uF to 52.5 uF. - In this case, **66.3 µF** exceeds the tolerance, indicating the capacitor is not functioning within its rated specification and may need replacement. **Questions to Complete:** 1. **You measure 7.2A on the start winding of a compressor, the voltage across it is 212v, the rated MFD on the capacitor is 95uF +/- 10%. Is the capacitor faulty?** 2. **Describe the common failure of electric motors, mechanically and electrically.** 3. **U to V = 2.5 ohms. U to W = O.L. V to W = O.L. What is the probable cause for** **these readings?** 4. **C to S = 4.8 ohms. C to R = 2.8 ohms. What would you expect S to R to be?** 5. **Why is it important for the start winding to drop out during motor operation on a single-phase motor?** 6. **S to C = O.L. R to C = O.L. S to R = 5.5 ohms. What is a probable cause for these readings?** 7. **True or False: It is good practice to replace the starting components on a single-phase compressor that has failed.** 8. **What is the difference between inherent motor protection and external motor protection?**