Immunology Past Paper PDF
Document Details
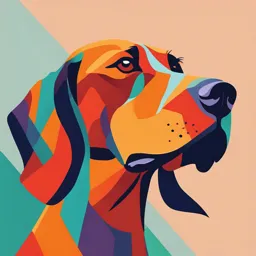
Uploaded by SeamlessBiedermeier7041
Tags
Summary
This document provides an introduction to immunology, outlining the overview of the physiological immune system and key questions. The document explores the different parts of the immune system, including barriers, cells, and molecules, and details how it works in defending against harmful invaders.
Full Transcript
Introduction to Immunology – Jan 6 Overview of Physiological Systems and the Immune System Physiological systems, like the immune system, are circular. There's no clear start or end—they continuously work to maintain balance (called homeostasis). The immune system is hard to teach because everyth...
Introduction to Immunology – Jan 6 Overview of Physiological Systems and the Immune System Physiological systems, like the immune system, are circular. There's no clear start or end—they continuously work to maintain balance (called homeostasis). The immune system is hard to teach because everything is interconnected. Understanding the immune system involves breaking it into parts and seeing how they fit together. Key Questions About the Immune System How do we know we have an immune system? When you get a cut or scrape, the area swells, turns red, and heals itself. This is the immune system in action. Allergies, like poison ivy reactions, show the immune system’s ability to react to substances. After being infected once (like catching the flu), the immune system protects us from getting the same illness again quickly. How do we know we need an immune system? Some children are born with no immune system (severe combined immune deficiency). Without treatment, they cannot survive infections. o Experiments with animals show that without immune system components (like certain genes), organisms can’t fight off infections. What is the purpose of the immune system? 1. To defend against invaders like bacteria and viruses (like soldiers protecting a city). 2. To distinguish between self and non-self, ensuring the body doesn't attack itself. 3. To detect danger, focusing on threats that harm us. 4. To maintain peace and harmony with harmless bacteria and other microorganisms that live in our bodies. How Immunity Works When we’re exposed to something harmful (an infection), the immune system responds by fighting it off. This response is stronger and faster the second time because of something called immunological memory. This memory explains why vaccines protect us—they train the immune system to respond quickly to specific infections. Evidence That Vaccines Work Diseases like measles and polio were very common before vaccines but became rare after vaccines were widely used. Recent decreases in vaccination rates (e.g., during COVID) have led to the return of some diseases. Components of the Immune System The immune system has three main parts: 1. Barriers (today’s focus) 2. Cells (e.g., white blood cells) 3. Molecules (e.g., antibodies) Barriers: The First Line of Defense Barriers protect us from infections in four main ways: Mechanical Defenses: these defenses are physical actions or structures that prevent harmful things from entering the body. 1. Epithelial cells joined by tight junctions: These are like bricks in a wall—cells are tightly connected to stop invaders like bacteria or viruses from sneaking in. 2. Longitudinal flow of air or fluid: Air and liquids (like saliva, urine, or digestive fluids) flow through certain parts of the body to wash away harmful substances. 3. Movement of mucus by cilia: Cilia are tiny hair-like structures that sweep mucus (and the dirt or germs stuck in it) out of the respiratory system. 4. Tears and nasal cilia: Tears wash away particles from your eyes, and nasal cilia push mucus out of the nose. Skin: Key Layers of the Skin Epidermis (Outer Layer): 1. Corneal layer (keratin “shield”): The outermost part of the skin. Made of a tough protein called keratin. Think of it as a "shield" that protects the body from the outside world (like bacteria, dirt, or injuries). ❑ Keratinocytes: These are special cells in the epidermis that produce keratin. They form a tightly packed barrier that blocks harmful substances and microbes. Dermis (Inner Layer): Below the epidermis, it contains important immune cells and structures that help the skin protect the body. Immune Cells: 1. Macrophages: These cells "eat" harmful invaders like bacteria or dead cells. 2. Langerhans Cells: Found near the border of the dermis and epidermis. They act as "watchdogs," alerting the immune system when they detect something harmful. 3. Mast Cells: These release signals (like histamine) to help the body fight infections or heal injuries. 4. Dermal Dendritic Cells (DCs): These cells also help the immune system recognize and respond to harmful invaders. Chemical Defenses: these are substances the body produces to kill or stop harmful invaders. 1. Fatty acids: Found on the skin, they create an environment where bacteria struggle to survive. 2. Low pH (acidic environment): For example, the stomach has a very acidic environment that kills most bacteria in food. 3. Enzymes (pepsin): Found in the stomach and other areas, they break down harmful substances or kill bacteria. In tears and saliva (lysozyme): These enzymes break apart bacteria to stop infections. 4. Pulmonary surfactant: A substance in the lungs that helps keep harmful germs away. a. Chemical molecules that directly attack invaders: β-defensins, α-defensins, RegIII, cathelicidins, and histatins: These are special proteins that act like "natural antibiotics," killing bacteria, fungi, or other harmful organisms. Microbiological Defenses: Normal microbiota: These are the "good" bacteria that naturally live on your skin and inside your body (like in the gut). They take up space and nutrients, so harmful bacteria can’t easily grow. Nutritional Barriers The body keeps important nutrients, like iron, tightly controlled. Bacteria need these nutrients to grow, so the body actively hides or binds them to prevent bacteria from thriving. Microbes need iron to survive but can’t find it easily because the body keeps it locked up in proteins like hemoglobin, hemopexin, and haptoglobin. The body uses mechanisms like NRAMP1 to pump iron out of cells and prevent microbes from accessing it. Bacteria fight back by secreting siderophores, which are tools they use to steal iron for their growth. Innate Immunity I - Jan 8 Difference Between Innate and Adaptive Immunity Innate vs. Adaptive Immunity Working Together How Innate and Adaptive Immunity Work Together The innate system is like the body’s alarm system, detecting threats and responding immediately. The adaptive system is like a detective, learning about the threat and creating a specific plan to eliminate it. Important: Adaptive immunity cannot work without the innate system activating it first. Innate and Adaptive Immunity Overlapping Types of Immunity → Innate Immunity: General defense present in everyone. → Adaptive Immunity: Tailored defense that improves with experience. → Cellular Immunity: Carried out by immune cells like T cells and macrophages. → Humoral Immunity: Involves antibodies and other substances in body fluids. How the Immune System Responds to an Infection Primary Response: The first time your body encounters a germ, the immune system takes longer to respond and is not as strong. Secondary Response: The next time you encounter the same germ, the adaptive immune system "remembers" it. The response is faster and stronger due to memory cells. Cells of the Immune System Adaptive Immunity Cells T Cells: Attack infected cells directly. B Cells: Produce antibodies to fight specific germs. Natural Killer (NK) Cells: Kill harmful cells without needing prior instructions. Innate Immunity Cells Neutrophils: First responders to infection or injury. Eat germs (phagocytosis). Can release DNA nets (NETs) to trap and kill bacteria. Short-lived but produced in large numbers. Macrophages: Big "eaters" consume germs and clean up dead cells. Found it in tissues like the skin and liver. Monocytes in the blood can transform into macrophages in tissues. Dendritic Cells: Act as messengers between innate and adaptive immunity. Capture germs, break them down, and show their pieces to other immune cells to trigger a response. Mast Cells: Found in tissues like the skin and lungs. Release histamine, which increases blood flow to injured areas (important in allergies and inflammation). Eosinophils and Basophils: Help fight parasites and play a role in allergic reactions. Phagocytosis: How Cells "Eat" Germs 1. A germ is recognized by immune cells like macrophages or dendritic cells. 2. The immune cell surrounds and engulfs the germ, forming a bubble (phagosome). 3. The phagosome fuses with a lysosome (a cell part filled with toxic chemicals). 4. Inside the phagolysosome, the germ is broken down and destroyed. How the Phagolysosome Kills Germs Low pH (acidic environment): Kills most germs. Toxic Molecules: Hydrogen peroxide (like the disinfectant you use on wounds). Bleach (produced inside the cell!). Enzymes: Lysozyme: Breaks down bacterial walls. Defensins: Punch holes in bacteria. Nutritional Starvation: Molecules like lactoferrin remove iron, which bacteria need to survive. Blood Composition Plasma (55%): Liquid part of blood containing hormones, antibodies, and nutrients. Cells (45%): Includes: Red Blood Cells (RBCs): Carry oxygen. White Blood Cells (WBCs): Fight infections. Platelets: Help blood clot (stop bleeding). Blood Cell Formation Blood cells are made in the bone marrow from stem cells. Stem cells produce: Myeloid cells: Neutrophils, macrophages, and dendritic cells. Lymphoid cells: T cells, B cells, and NK cells. Founders of Immunology Paul Ehrlich: Discovered antibodies and coined the terms "antibody" and "antigen." Developed the "magic bullet" idea (how antibodies target germs). Elie Metchnikoff: Discovered phagocytosis (how cells eat germs). Studied macrophages and their role in immunity. Innate Immunity II - Jan 10 Overview: Anatomy of the Immune Response The immune system is designed to detect and respond to infections using a series of defenses. These defenses work in three main stages: 1. Barrier Immunity: Physical barriers like skin and mucus prevent germs from entering the body. 2. Innate Immunity: The first line of defense. It's immediate but general (not specific to any one germ). 3. Adaptive Immunity: The second line of defense. It’s slower but highly specific and remembers past infections. Immune System Organization The immune system operates like a "liquid organ," circulating cells and molecules throughout the body. Key Organs and Their Functions Primary Organs: Bone Marrow: Produces immune cells like white blood cells. Thymus: Matures T-cells, a key type of immune cell. Secondary Organs: Lymph Nodes: Small structures that act as "hubs" where immune cells meet pathogens and get activated. Spleen: o Red Pulp: Recycles old or damaged red blood cells. o White Pulp: Houses T-cells and B-cells to fight infections. Peyer’s Patches: Found in the intestine; detect germs in the gut. The Lymphatic System A network of vessels and nodes parallel to the circulatory system. Functions: Transports immune cells and germs to lymph nodes for activation. Returns immune cells to infection sites. Lymph Nodes: Cortex: Contains B-cells (antibody production). Paracortex: Contains T-cells (cell-mediated immunity). Medulla: Contains macrophages and plasma cells to finish immune responses. How the Immune System Recognizes Pathogens Immune cells use special sensors called Pattern Recognition Receptors (PRRs) to detect germs. These receptors are located: 1. On cell surfaces. 2. Inside the cell (cytoplasm). 3. Inside cellular compartments (endosomes). Major PRR Families: 1. Toll-like Receptors (TLRs): Recognize bacteria, viruses, and fungi. Example: TLR4 detects bacterial components like lipopolysaccharides (LPS). 2. C-type Lectin Receptors (CLRs): Detect fungal components. 3. Nucleotide Oligomerization Receptors (NLRs): Detect bacteria inside cells. 4. RIG-I-like Receptors (RLRs): Detect viral RNA. Pathogen-Associated Molecular Patterns (PAMPs): These are unique features of pathogens that PRRs recognize: 1. Bacteria: Lipopolysaccharides, peptidoglycans, flagellin. 2. Viruses: RNA, DNA, and capsid proteins. 3. Fungi: Zymosan and glucans. 4. Cytokines: Communication Molecules. Cytokines are proteins that help immune cells communicate and coordinate responses. Functions of Cytokines: Cause inflammation (swelling, redness). Attract immune cells to infection sites. Promote the production of antibodies. Types of Cytokine Actions: Pleiotropy: One cytokine affects different cell types in different ways. Redundancy: Multiple cytokines can produce the same effect. Cascade Induction: Cytokines trigger other cytokines, amplifying the response. Major Cytokine Families: 1. Interleukin-1 Family: Causes inflammation. 2. Hematopoietin Family (e.g., IL-2): Promotes immune cell growth. 3. Interferon Family: Defends against viruses. 4. Tumor Necrosis Factor Family: Induces inflammation and cell death. 5. Chemokines: Guide immune cells to infection sites. JAK-STAT Pathway (How Cytokines Work) 1. Signal Reception: A cytokine like IL-2 binds to its receptor on a cell. 2. Signal Transduction: Enzymes (JAKs) activate proteins (STATs) inside the cell. 3. Gene Activation: STATs move into the nucleus and turn specific genes on/off. 4. Result: The cell grows, divides, or performs other immune functions. 5. Neutrophils: Key First Responders 6. Role: Quickly arrive at wound or infection sites to fight germs. Functions: Phagocytosis: Eat and destroy germs. NETosis: Release DNA nets (Neutrophil Extracellular Traps) to trap and kill bacteria. Phagocytosis: How Germs Are Destroyed A pathogen binds to PRRs on immune cells like macrophages or neutrophils. The immune cell engulfs the pathogen into a bubble (phagosome). The phagosome fuses with a lysosome, forming a phagolysosome. The pathogen is destroyed using: Low pH (acidic environment). Enzymes (e.g., lysozyme breaks down bacterial walls). Toxic molecules: Reactive Oxygen Species (e.g., hydrogen peroxide). Bleach-like chemicals (hypochlorous acid). Nutrient deprivation: Molecules like lactoferrin remove iron, starving bacteria. DAMPs: Signals of Cellular Damage When cells are injured or die, they release molecules called Damage-Associated Molecular Patterns (DAMPs) to alert the immune system. Examples of DAMPs: ATP: Released outside the cell to signal damage. Histones: Normally inside the nucleus but signal danger when outside. Heat Shock Proteins: Trigger immune activation. Innate Immunity III - Jan 13 Self vs. Non-Self: How the Immune System Knows What to Attack Self = Your body’s own healthy cells. Non-Self = Invaders like bacteria, viruses, or abnormal cells (e.g., cancer). Why It Matters: The immune system must attack invaders but avoid harming your own cells. If it fails, it can lead to autoimmune diseases, where the immune system attacks healthy tissues. Immunological Tolerance: The Immune System’s Self-Control Immunological Tolerance: The ability of the immune system to recognize self-cells and not attack them. How It Works: MHC Proteins (Major Histocompatibility Complex): Special "ID badges" on your cells that mark them as "self." Natural Killer (NK) Cells: Immune cells that: Check cells for MHC markers. Destroy infected, stressed, or cancerous cells that lack MHC markers. Balance of Signals: Healthy cells with MHC send an "inhibitory" signal to stop NK cells from killing. Abnormal cells without MHC or with "danger signals" trigger NK cells to attack. How NK Cells Kill Fas-Fas Ligand Pathway: NK cells attach to target cells and send a signal that triggers cell suicide (apoptosis). Perforin-Granzyme Pathway: Perforin: Creates holes in the target cell’s membrane. Granzyme B: Enters through the holes and forces the target cell to self-destruct. Cytokine Release: NK cells release cytokines (e.g., interferon-gamma) to alert and activate other immune cells. The Complement System: A Backup Defense A group of about 50 proteins in the blood that: Direct Killing of Pathogens (Alternative Pathway): This is a built-in defense mechanism that doesn't require any prior help, like antibodies. Special proteins in the immune system directly target and destroy harmful cells by damaging their outer layer, causing them to die. Killing Through Antibodies (Classical Pathway): In this method, the immune system uses antibodies (proteins that recognize specific invaders) to attach to harmful cells. Once antibodies stick to the invader, they trigger other immune proteins to destroy the target. Killing Through Lectins (Lectin Pathway): Lectins are special molecules that recognize and attach to sugars found on the surfaces of certain pathogens. This attachment activates a response that kills the harmful cells. Opsonization (Marking Pathogens for Destruction): The immune system "tags" harmful cells using special proteins. This tag acts like a flag, making it easier for immune cells (like macrophages) to recognize, grab, and "eat" the pathogens. Think of it as putting a target sign on the invader for the immune army to see. Recruiting More Immune Cells and Causing Inflammation: The immune system releases chemoattractant, which are signals that attract white blood cells (like neutrophils and macrophages) to the infection site. These cells help by killing invaders and clearing out dead or damaged tissue. The process also causes inflammation, which is the body’s way of isolating the infection and speeding up the healing process. Symptoms like redness, swelling, and warmth happen during inflammation. Alternative Pathway Steps: 1. C3 Activation: Protein C3 splits into: ❑ C3a: Attracts immune cells to the infection site. ❑ C3b: Sticks to pathogens, marking them for destruction. 2. C3 Convertase Formation: C3b binds to the pathogen and recruits helper proteins to amplify the response. 3. C5 Convertase Formation: Another protein, C5, is activated and splits into: ❑ C5a: Promotes inflammation. ❑ C5b: Starts building the Membrane Attack Complex (MAC). Membrane Attack Complex (MAC): Proteins form a hole in the pathogen’s membrane, causing it to burst (lysis). Self-Protection Mechanisms: Your cells have tools to prevent accidental attack: dissociation (breaking) of C3 convertases on the surface of our body cells DAF (Decay Accelerating Factor): Stops complement activation on self-cells. (ex. CD55) Complement Receptor 1: Breaks apart harmful complexes. (ex. CD35) Inflammation: The Body’s Response to Injury or Infection Signs of Inflammation (the 5 "classical features"): 1. Redness (Rubor): Increased blood flow to the area. 2. Heat (Calor): Warmth from active immune cells. 3. Pain (Dolor): Nerves become sensitive. 4. Swelling (Tumor): Fluid and immune cells accumulate. 5. Loss of Function (Functio Laesa): Temporary impairment (weakness) of the area. Purpose of Inflammation: → Bring immune cells to the infection site to kill germs. → Control temperature (fever slows down germs and boosts immune activity). → Eliminate infection with the help of neutrophils, macrophages, and other cells. → Repair tissues after the infection is cleared. What is an Inflammatory Circuit? The inflammatory circuit explains how your body reacts to injury, infection, or harmful stimuli. How Does it Work? Triggering the Response: An inflammatory stimulus (like an injury, infection, or toxin) kicks off the process. The body detects this problem through sensors (special cells or proteins that notice something isn’t right). Sending Inflammatory Signals: Once the sensors detect the problem, they send out inflammatory signals. These signals tell the immune system to respond. Activating Two Main Targets: Effectors: These are immune cells like neutrophils, monocytes, and eosinophils. They directly work to eliminate the problem (like killing bacteria or repairing tissue). Non-effectors: These include tissues and organs (like your brain and kidneys) that adjust their functions to help support the immune response. For example: o Fever: Raises body temperature to slow down bacteria. o Sleep: Conserves energy for the body to focus on healing. Feedback Loops in the System Negative Feedback Loop (Resistance): After the immune cells (effectors) deal with the problem, the body sends signals to stop the inflammation and prevent unnecessary damage. Feedforward Loop (Adaptation): Tissues and organs adapt to inflammation, helping the body tolerate the issue while the immune system works to eliminate it. What Triggers Inflammation? External Triggers (Exogenous): Germ components like PAMPs (Pathogen-Associated Molecular Patterns), e.g., bacterial parts (LPS, flagellin). Chemicals such as alum or asbestos interacting with cellular receptors (TLRs, NLRs) Toxins and venom components (phytochemicals, bee sting) Complement proteins: C3a, C5a acting through C3aR, C5aR Internal Triggers (Endogenous): DAMPs (Damage-Associated Molecular Patterns), e.g.: ❑ ATP: Signals danger when outside cells. ❑ Histones: Normally inside cells but dangerous when released. ❑ Heat Shock Proteins: Released during injury like burns or frostbite. Key Inflammatory Molecules 1. IL-1β (Interleukin-1 beta): Triggers inflammation and fever. 2. TNFα (Tumor Necrosis Factor-alpha): Promotes inflammation and can kill cells. 3. IL-6 (Interleukin-6): Increases inflammation and helps produce fever. Introduction to Adaptive Immunity - Jan 15 Louis Pasteur and the Rabies Vaccine → Historical Milestone: Louis Pasteur created the first rabies vaccine in 1885. → How It Worked: He used a weakened (attenuated) form of the rabies virus to help the immune system recognize and fight the disease. o Famous Example: Joseph Meister, a boy bitten by a rabid dog, was successfully treated with the vaccine. → Impact: Pasteur’s work demonstrated how vaccines can train the immune system to prevent serious diseases. Paul Ehrlich’s Side Chain Theory Key Idea: Antibodies (special proteins made by the immune system) bind to antigens (foreign substances) in a specific way, like a "lock and key." ❑ Antibodies: Proteins that neutralize antigens. ❑ Side Chains: Specific parts of antibodies that match the shape of antigens. ❑ Significance: Helped explain how the immune system recognizes and attacks foreign invaders. Innate vs. Adaptive Immunity Humoral Immunity: Involves antibodies in body fluids. Cellular Immunity: Involves immune cells like T cells that directly attack infected cells. B Cells and T Cells B Cells: Develop in the bone marrow. → Produce antibodies (proteins that target specific germs). → Play a key role in humoral immunity. T Cells: Develop in the thymus. Thymus: Organ located above the heart; larger in children and shrinks with age. Essential for T cell development. It contains medulla (inner part) and cortex Discovery: Jacques Miller (1960) showed removing the thymus in mice eliminated adaptive immunity. Types of T Cells: Play a key role in cellular immunity. CD4+ T Cells (Helper T Cells): Help activate other immune cells. CD8+ T Cells (Cytotoxic T Cells): Kill infected cells directly. How B and T Cells Recognize Invaders B Cell Receptors (BCRs): Found on the surface of B cells. Made of heavy and light chains. Bind to antigens directly. (B cell receptors and antibodies can be generated to recognize any type of antigen in any three-dimensional conformation) Can either stay attached to the cell or be released as antibodies. o Each BCR is paired with helper proteins called Igα/Igβ, which help send the activation signal. The signal transduction process (sending signals) is inactive. o When an antigen binds to multiple BCRs, it crosslinks them (pulls them together) o This crosslinking brings BCRs and their helper proteins (Igα/Igβ) closer, activating a signaling process. o B-cell receptor (BCR) binds specifically to one unique antigen (or epitope). Only recognize one specific molecule on a pathogen. o Receptors: BCRs can detect a wide range of molecules, such as proteins, sugars, or fats. T Cell Receptors (TCRs): Found on the surface of T cells. Do not bind to free-floating antigens. Recognize peptide antigens (8-24 amino acids) only when they are presented by MHC molecules on other cells: ❑ Class I MHC: Presents antigens to CD8+ T cells. ❑ Class II MHC: Presents antigens to CD4+ T cells. T-cell receptor (TCR) that also binds to one specific antigen (epitope). Only recognize antigens presented by other cells (on MHC molecules). BCRs and TCRs Are Encoded by Recombined Genes BCRs and TCRs are created through a process called genetic recombination. This involves rearranging parts of DNA (called V, D, and J segments) to form a unique receptor for each cell. These rearrangements happen during the early development of B and T cells, before they encounter any antigens. Hozumi and Tonegawa (1976): Found how genes for BCRs are rearranged to create diversity. This discovery earned the Nobel Prize in 1987. Tak Mak and Mark Davis (1984): Discovered that similar rearrangement occurs to create TCRs. Antigens and Epitopes Antigen: A foreign molecule (like a piece of a virus or bacteria) that triggers an immune response. Epitope: A specific part of the antigen that is recognized by BCRs or TCRs. Clonal Selection Theory Discovery: Macfarlane Burnet developed the Clonal Selection Theory, which explains how the immune system specifically targets pathogens. He won the Nobel Prize in 1960 for this groundbreaking work. Deletion: Cells that react strongly to the body’s own molecules (self-antigens) are eliminated early in their development to prevent autoimmune diseases. Clonal Selection and Expansion: o When a foreign substance (antigen) enters the body, only the immune cell with a receptor specific to that antigen is activated. o This activated cell multiplies (clonal expansion) to create more identical cells that can fight the pathogen. Clonal selection happens during the primary response phase, leading to the production of memory cells. T cell Immunity I – Jan 17 Overview of T Cell Receptor (TCR) Recognition and MHC Interaction The immune system uses T cells and MHC (Major Histocompatibility Complex) molecules to detect infections and abnormal cells. This system plays a key role in defending the body, preventing organ transplant rejection, and maintaining immune surveillance. Key Historical Contributions E. Donald Thomas: Developed ways to overcome immune rejection in bone marrow transplants. o It was shown that matching immune systems improves transplant survival. o First successful transplant: Nancy Lowry (1960) received bone marrow from her identical twin. o Nobel Prize (1990): For groundbreaking research in transplantation. Jacques Miller: Discovered T cells by observing that removing the thymus (an organ where T cells develop) stopped immune rejection of transplants. T Cells: The Immune System’s Soldiers T Cell Receptor (TCR): Structure: Made of alpha and beta chains with two regions: o Variable Region: Detects antigens (unique for each T cell). o Constant Region: Provides stability. T Cell Antigens and Epitopes Buried Epitopes: The regions of antigens (called epitopes) that T cells recognize are often hidden deep within the protein structure of the antigen. These epitopes need to be exposed for T cells to recognize and respond to them. Antigen Breakdown: Before T cells can detect an antigen, the antigen must first be broken down into smaller pieces called peptide fragments. This is done by specialized immune cells (like dendritic cells) through a process called antigen processing. Binding to MHC Molecule: Once the peptide fragments are created, they bind to a specific MHC (Major Histocompatibility Complex) molecule. T Cell Receptor (TCR) Binding: The T cell receptor (TCR) on a T cell recognizes and binds to the peptide-MHC complex. This interaction is crucial for activating the T cell, enabling it to carry out its immune response. MHC and Antigen Presentation MHC molecules are like "display cases" that show antigens to T cells. There are two types of MHC: Class I MHC: What It Does: Presents antigens from inside the cell (e.g., viral proteins). Where It’s Found: On almost all cells in the body. Recognized By: CD8+ T cells (cytotoxic T cells), which destroy infected or abnormal cells. Class II MHC: What It Does: Presents antigens from outside the cell (e.g., bacteria). Where It’s Found: On antigen-presenting cells (APCs) like dendritic cells, macrophages, and B cells. Recognized By: CD4+ T cells (helper T cells), which activate other immune cells. How Antigens Are Processed and Presented Class I Pathway (For Intracellular Antigens): Source: Proteins from inside the cell (e.g., viruses or self-proteins). Process: Proteins are broken into small pieces (8–10 amino acids) by proteasomes. Pieces are transported into the endoplasmic reticulum by TAP proteins. MHC class I binds these pieces and displays them on the cell surface. Class II Pathway (For Extracellular Antigens): Source: Proteins from outside the cell (e.g., bacteria or parasites). Process: Proteins are engulfed by APCs via phagocytosis and broken down in lysosomes. Pieces (13–24 amino acids) are loaded onto MHC class II molecules. MHC class II displays them on the cell surface for helper T cells to recognize. Cross-Presentation: Unique to dendritic cells. Dendritic cells can present extracellular antigens on MHC class I to activate CD8+ T cells. Importance: Allows the immune system to respond to viruses that infect non-APC cells. MHC Diversity and Its Role Why Is MHC Diverse? MHC molecules differ greatly between individuals. This variation allows the immune system to recognize a wide range of pathogens, increasing survival against infections. Organ Transplant Rejection Why Rejection Happens: T cells can detect the donor's cells as "foreign" if MHC molecules or peptides don’t match. How It Works: Direct Allorecognition: T cells directly recognize the donor’s MHC molecules as foreign. Indirect Allorecognition: T cells recognize fragments of the donor’s proteins presented by the recipient’s MHC molecules. Bacteria and MHC Exploitation Some bacteria exploit the MHC system by producing superantigens: Molecules made by bacteria (e.g., in toxic shock syndrome or flesh-eating disease). They bypass normal antigen recognition by stabilizing TCR-MHC interactions non- specifically. Impact: Causes massive T cell activation. Overactivation of the immune system can lead to tissue damage, helping bacteria survive. T cell Immunity II - Jan 20 Anatomy of the Thymus Structure: 1. Cortex: The outer region where early T cell development occurs. 2. Medulla: The inner region where T cells undergo further maturation. 3. Corticomedullary Junction: The area between the cortex and medulla where developing T cells transition and interact with thymic cells. What Happens During T Cell Development in the Thymus? TCR Gene Rearrangement: Genes encoding T cell receptors (TCRs) are recombined to create diverse receptors, enabling T cells to recognize a variety of antigens. Positive Selection: T cells that can weakly bind to self-MHC molecules survive. This ensures T cells can recognize MHC molecules, which are essential for antigen presentation. Negative Selection: T cells that bind too strongly to self-MHC or self-peptides are eliminated to prevent autoimmunity. Outcome: The remaining T cells have TCRs capable of recognizing foreign antigens presented by self-MHC molecules. T Cell Receptor (TCR) Genes Gene Loci: TCR genes are located at four loci—alpha, beta, delta, and gamma. Rearrangement: TCR beta genes rearrange first and pair with a protein called "pre-T alpha" to form the pre-TCR. TCR alpha genes rearrange later to complete the receptor. Function: This rearrangement generates a unique TCR for each T cell, ensuring diversity in antigen recognition. T Cell Development Overview T cells develop in the thymus and go through specific stages characterized by the expression of CD4 and CD8 molecules. These stages ensure that T cells can function effectively in recognizing and responding to infections while avoiding harm to the body. Stages Explained: ❑ DN1 and DN2 (Double Negative): At this stage, the cells do not express CD4 or CD8 markers (hence "double negative"). ❑ DN3: The TCR beta chain undergoes rearrangement to form a functional pre-T cell receptor (pre-TCR). ❑ DN4: After passing the beta selection checkpoint, these cells stop beta rearrangement and start proliferating. ❑ DP (Double Positive): T cells now express both CD4 and CD8 molecules on their surface. This stage is critical for testing the ability of T cells to interact with self-MHC molecules. Beta Selection (Checkpoint for Quality Control): 1. The TCR beta chain has been correctly rearranged. 2. T cells can proliferate and further differentiate. 3. CD4 and CD8 expression begins. 4. The next step, TCR alpha rearrangement, is initiated. TCR Alpha Rearrangement: This process allows T cells to develop a complete TCR (T-cell receptor) capable of recognizing specific antigens. Final Differentiation: After testing interactions with MHC molecules: T cells expressing CD4 become helper T cells. T cells expressing CD8 become cytotoxic T cells. Selection Process: Positive Selection: Ensures T cells can recognize MHC molecules (needed for immune response). Negative Selection: Removes T cells that react strongly to self-antigens, preventing autoimmunity. o CD4+ and CD8+ T Cells are Positively Selected in the Cortex and Negatively Selected in the Medulla by Thymic Epithelial cells T Cell Receptor (TCR) α and β Gene Rearrangement α-chain locus: On chromosome 14, it has many "building blocks" (labeled V, J, and C) that get rearranged to make the TCR alpha chain. β-chain locus: On chromosome 7, it also rearranges segments (labeled V, D, J, and C) to make the beta chain. T Cell Tolerance Induction T cells must recognize foreign invaders but not attack the body’s own cells. This process ensures they don’t react to “self.” If T cells have low affinity for self-molecules, they die because they’re not useful (90%- 96% of cells fail and die). Intermediate affinity cells are positively selected and survive. High affinity cells may develop into special cells (T regulatory cells, or Tregs) that help prevent autoimmune responses. Very high affinity cells are deleted because they might cause harm. Thymus and Lymph Nodes: T Cell Activation Journey The Thymus: This is where T cells are "trained" to recognize foreign invaders while ignoring the body’s own cells. Lymph Nodes: After leaving the thymus, T cells travel to lymph nodes, where they encounter antigens (foreign particles) presented by dendritic cells. Dendritic cells "show" the T cells the antigen. They present antigens to both types of T cells: o CD4+ T cells: Recognize MHC class II and help activate other immune cells. o CD8+ T cells: Recognize MHC class I and directly kill infected or cancerous cells. o Special skill: Dendritic cells can present antigens to CD8+ T cells using cross- presentation, even when the antigens come from outside the cell. If the T cells recognize it, they get activated and turn into effector T cells, ready to fight infections. T Cell Activation Requires 3 Signals → Signal 1: TCR binds to an antigen presented on an MHC molecule. → Signal 2: Costimulatory interaction occurs between proteins on the T cell and antigen- presenting cell (APC). → Signal 3: Cytokines (chemical messengers) further activate the T cell. T Cell Tolerance by Anergy Anergy is a "sleep mode" for T cells. If a T cell doesn’t get proper signals (like from costimulatory receptors), it won’t activate. This is a safety mechanism to prevent autoimmunity. Natural Killer (NK) Cells vs. T Cells: NK Cells: Part of the "first responders" in an infection. They don't need to recognize specific antigens to kill infected cells. T Cells: Take more time to activate but are highly specific in targeting infected cells. HIV and CD4+ T Cells: HIV targets CD4+ T cells, reducing their numbers and weakening the immune system. This leads to AIDS, where the body becomes vulnerable to infections. Treatment: Antiretroviral therapy helps slow down the progression of the disease. B cell Immunity I - Jan 22 Antibodies are proteins made by B-cells to fight off harmful invaders like germs or toxins. Key Features: Can recognize a wide variety of harmful molecules, unlike T-cell receptors. Work by neutralizing invaders or signaling other immune cells to destroy them. Medical Uses: Antibodies are used in treatments like: Humira (for inflammation). Keytruda (for cancer). Antibody Structure Parts of an Antibody: Heavy Chains: Larger parts that determine the antibody’s class (like IgG or IgA). Light Chains: Smaller supporting parts (two types: kappa or lambda). Variable Region: The unique part that binds to specific invaders (like a lock and key). Constant Region: Decides the antibody’s job in the immune system. Shape: Two heavy chains and two light chains are connected by strong chemical bonds (disulfide bonds). Hinge Region: Gives flexibility to help antibodies attach to invaders. Fragments: Fab (Fragment Antigen Binding): Binds to the invader. Fc (Fragment Crystallizable): Signals immune cells to act. Classes of Antibodies (Immunoglobulins) IgM: First antibody made during an infection. Forms a large structure with 10 binding sites (pentamer) and 2 binding sites as monomers Two Shapes: o Planar form: When not bound to anything, IgM looks flat. o Staple form: When IgM binds to a pathogen, it changes shape to better interact with immune system components. Main Functions: o Agglutination: ▪ IgM is great at clumping invaders together (like bundling harmful bacteria), which makes it easier for the immune system to clear them. o Starting the Complement Pathway: ▪ A molecule called C1q binds to IgM when it’s attached to a pathogen. o Opsonization: ▪ IgM coats pathogens, marking them for destruction. IgD: Found on immature B-cells with IgM. The exact role is not fully understood but plays a part in early immunity. IgG: The most common antibody in blood and tissues. Long-lasting and very important in secondary infections (when encountering a germ for the second time). Neutralizes toxins, promotes phagocytosis (engulfing of invaders), and activates complement. It has four subclasses (IgG1, IgG2, etc.). IgA: Found in body secretions like saliva, tears, mucus, and breast milk. Forms dimers (with four binding sites) and protects mucosal surfaces (like the gut or lungs). IgE: Involved in allergic reactions and fighting parasites. Works with cells like mast cells and eosinophils to release chemicals (e.g., histamine) to attack invaders. B-Cell Development Stages: 1. Pro-B Cell: Rearranges heavy chain genes. 2. Pre-B Cell: Rearranges light chain genes. 3. Immature B Cell: Displays IgM and IgD antibodies on its surface. 4. Mature B Cell: Travels to the spleen or lymph nodes for activation. Negative Selection: Self-reactive B-cells (those attacking the body’s own tissues) are eliminated to prevent autoimmune diseases. Blood Groups and Antibodies Blood types (A, B, AB, O) depend on antigens present on red blood cells: Example: Type A blood has A antigens and makes anti-B antibodies. Mismatch Transfusions: If blood types don’t match, the immune system attacks foreign blood cells, causing serious reactions. Natural Passive Immunity: This type of immunity is a natural process where antibodies are passed from the mother to the baby, providing the baby with temporary protection against infections. During Pregnancy: A type of antibody called IgG is transferred from the mother's blood to the baby's blood through the placenta. This is done via a specific transport system (called FcRn receptors) to help protect the baby before birth. Vaccination During Pregnancy: If a pregnant woman receives vaccines for diseases like tetanus or diphtheria, some of this protection is passed on to the baby, helping it fight these infections early in life. After Birth: Another antibody called IgA is passed to the baby through the mother’s breast milk. This helps protect the baby’s digestive system from infections. Artificial Passive Immunity: This type of immunity is achieved by giving a person antibodies directly, usually through medical treatments, to help fight diseases or immune deficiencies. Blood contains different components, such as: Plasma (the liquid part of the blood, where antibodies are found). White Blood Cells (WBCs) (important for immunity). Red Blood Cells (RBCs) (carry oxygen). Intravenous Immunoglobulin (IVIG): In this treatment, antibodies from healthy donors are collected from their plasma. These antibodies are injected into a patient to help fight infections or strengthen the immune system. Specialized Antibodies (Monoclonal Antibodies): Scientists create specific antibodies to target certain diseases or viruses, such as COVID-19 or cancers. B cell Immunity II - Jan 24 Overview of the Immune Response: 9 Steps Pathogen Breaches Barriers: Germs like bacteria, viruses, or parasites enter the body by bypassing protective barriers like the skin or mucous membranes. Innate Immune Response (First Line of Defense): Special sensors, called Pattern Recognition Receptors (PRRs), detect unique patterns on pathogens (called PAMPs). The body sends out chemical signals (cytokines) to call immune cells like neutrophils, macrophages, and dendritic cells to the infection site. Phagocytosis and Antigen Presentation: Phagocytes (e.g., macrophages) "eat" the germs and break them down into small pieces (antigens). These antigens are displayed on special molecules called MHC (Major Histocompatibility Complex) on the surface of phagocytes. Dendritic cells, a type of antigen-presenting cell (APC), travel to lymph nodes to show antigens to T cells. T-Cell Activation: Naive T cells (newly formed T cells that haven’t encountered an invader yet) are activated through 3 signals: Signal 1: T-cell receptor (TCR) binds to the MHC-peptide complex on APCs. Signal 2: Co-stimulatory molecules (e.g., CD40-CD40L, CD28-CD80/CD86) ensure proper activation. Signal 3: Cytokines (e.g., IL-2 and IL-12) guide T cells to specialize into different types. Effector T-Cell Differentiation: CD4 T cells (helper T cells) specialize in subtypes like T follicular helper cells (Tfh), which are key to activating B cells. CD8 T cells (cytotoxic T cells) kill infected cells directly. B-Cell Activation: Naive B cells: Each has a unique receptor (BCR) to recognize a specific invader. Once activated, B cells ingest the invader, break it down, and present its antigens on MHC Class II molecules. Activation needs 3 signals: Signal 1: BCR binds to the antigen. Signal 2: CD40-CD40L interaction between B cells and Tfh cells. Signal 3: Cytokines from Tfh cells further activate the B cell. Germinal Centers and B-Cell Differentiation: Germinal Centers (specialized areas in lymph nodes): Class Switching: B cells change the type of antibody they produce (e.g., IgM → IgG, IgA, or IgE) while keeping their ability to target the same invader. Somatic Hypermutation: B cells refine their receptors to improve binding to the invader. Differentiation outcomes: Plasma Cells: Antibody-producing factories that can live in bone marrow for long-term protection. Memory B Cells: Long-lived cells that "remember" the invader and respond faster in future infections. Secondary Immune Response: When the body encounters the same invader again: The response is much faster and stronger than the first time. Memory B and T cells reactivate, producing more effective antibodies and immune cells. Vaccination: Vaccines train the immune system to recognize invaders before actual infection. Types of vaccines: 1. Whole Microbe Vaccines: Use weakened or killed germs (e.g., early rabies vaccine). Rarely, these can cause illness. 2. Subunit Vaccines: Use only parts of the germ (e.g., DTaP for diphtheria, tetanus, pertussis). Safer than whole microbe vaccines. 3. Next-Generation Vaccines: Use genetic material like mRNA (e.g., COVID-19 vaccines) to teach cells how to fight the germ. Somatic Hypermutation: Random changes in the variable regions of B-cell receptors to improve how well they bind to antigens. Steps of the Immune Response: Understand the sequence: infection → innate response → antigen presentation → T-cell activation → B-cell activation → antibody production.