Microft1 C7 PDF
Document Details
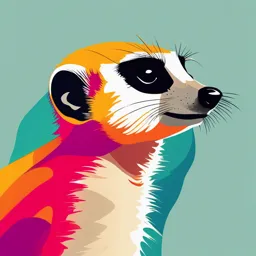
Uploaded by DecisiveFresno
Tags
Related
- Nucleic Acids Biochemistry Lecture 20 - RNA Splicing PDF
- BIOC 301 Biochemistry of Nucleic Acids Course Content Sep. 2024 PDF
- Nucleic Acids - 2024/2025 Biochemistry I - University of Babylon PDF
- CHEM 43: Biochemistry I: Nucleic Acids PDF
- Nucleic Acids CHEM 43 Biochemistry I PDF
- BCH1001 Macromolecules 4 - Nucleic Acids PDF
Summary
This document is an introduction to the study of genetics, exploring the structure and replication of genomes. It details nucleic acids, their components, and how they store genetic information.
Full Transcript
MICROFT1 C7 Pentose sugar: Either ribose (in RNA) or deoxyribose (in DNA). Genetics is the study of inheritance Nitrogenous base: One of five and inheritable traits as expressed possible...
MICROFT1 C7 Pentose sugar: Either ribose (in RNA) or deoxyribose (in DNA). Genetics is the study of inheritance Nitrogenous base: One of five and inheritable traits as expressed possible nitrogen-containing in an organism’s genetic material. molecules: Adenine (A), Guanine Geneticists study many aspects of (G), Cytosine (C), Thymine (T) (in inheritance, including the physical DNA only), or Uracil (U) (in RNA structure and function of genetic only). material, mutations, and the Base Pairing: The nitrogenous transfer of genetic material among bases can bond with each other in organisms. specific pairs: ○ DNA: Adenine (A) always pairs The Structure and Replication of with Thymine (T) with two Genomes hydrogen bonds. Guanine (G) The genome (jē’nōm) of a cell or always pairs with Cytosine (C) virus is its entire genetic with three hydrogen bonds. complement, including both its ○ RNA: Adenine (A) pairs with Uracil genes—specific sequences of (U) with two hydrogen bonds, nucleotides that code for RNA or similar to A-T in DNA. Guanine (G) polypeptide molecules—and still pairs with Cytosine (C) with nucleotide sequences that connect three hydrogen bonds. genes to one another. The genomes Genetic Information: The specific of cells and DNA viruses are sequence of these nitrogenous composed solely of mol-ecules of bases in a nucleic acid molecule deoxyribonucleic acid (DNA), encodes the genetic information, whereas RNA viruses use ribonucleic similar to how computer code uses a acid instead. sequence of zeros and ones. Deoxyribonucleotides are linked The Structure of Nucleic Acids through their sugars and Nucleic Acids: These are large phosphates to form the two molecules that store and transmit backbones of the familiar, helical, genetic information. double-stranded DNA (dsDNA) Polymers: Nucleic acids are built molecule (FIGURE 7.1d). The carbon from smaller units called nucleotides, atoms of deoxyribose are numbered linked together like beads on a 1’ (pronounced “one prime”) string. through 5’ (“five prime”). One end Nucleotides: These are the of a DNA strand is called the 5’ end building blocks of nucleic acids. because it terminates in a Each nucleotide consists of three phosphate group attached to a 5’ parts: carbon. The opposite end of that ○ Phosphate group: A phosphate DNA strand terminates with a group. hydroxyl group bound to a 3’ carbon ○ Nucleoside: A nucleoside is made of deoxyribose, so that end is called of: the 3’ end. The two strands are constructed similarly but are oriented in opposite directions to each other; one strand runs in a 5’ genome consists primarily of a 4.6 to 3’ direction, while the other runs 106 bp DNA mole-cule that is about 3’ to 5’. Scientists say the two 1600 mm long—800 times longer strands are antiparallel. The base than the cell. pairs extend into the middle of the The human genome has about 6 molecule in a way reminiscent of billion bp in 46 nuclear DNA the steps of a spiral staircase. The molecules and numerous copies of a hydrophobic bases are tucked inside unique mitochondrial DNA molecule, the molecule—away from water— and the entire genome would be while the hydrophilic sugars and about 3 meters (3 million mm) long phosphates are outside. This if all DNA molecules from a single provides an explanation for the cell were laid end to end. Most of a double-helical structure of cellular human cellular genome is packed DNA. into a nucleus that is typically only 5 The lengths of DNA molecules are mm in diameter. This is like packing not usually given in metric units; 45 miles of thread into a golf ball instead, the length of a DNA and still being able to access any molecule is expressed in base pairs. particular section of the thread. To For example, the genome of an understand how cells package such insect-dwelling bacterium prodigious amounts of DNA into tentatively named Nasuia such small spaces, we must first deltocephalinicola (nă-soo’ē-ă del- understand that bacteria, archaea, to-sef’ă-lĭn-ĭ-kō-lă) is 112,091 bp and eukaryotes package DNA in long, making it the smallest known different ways. We begin by cellular genome discovered to date. examining the structure of Most known bacteria have genomes prokaryotic genomes. of 1.5 to 6 million base pairs. The structure of DNA helps explain its ability to act as genetic material. The Structure of Prokaryotic First, the linear sequence of Genomes nucleotides car-ries the instructions Prokaryotic Chromosomes for the synthesis of polypeptides and RNA molecules—in much the Located in the nucleoid region of the way a sequence of letters carries cytoplasm. infor-mation used to form words Typically consist of a single, circular and sentences. Second, the com- DNA molecule (exceptions exist). plementary structure of the two Cell containing a single copy of the strands allows a cell to make exact genome is called haploid. copies to pass to its progeny. We No membrane surrounds the will examine the genetic code and nucleoid, but a distinct boundary is DNA replication shortly.The amount visible. of DNA in a genome can be Chromosomal DNA is folded into extraordinary, as some examples loops (50,000 to 100,000 bp long) will illustrate. A cell of the bacterium held by proteins and RNA. Escherichia coli (esh-ĕ-rik’ē-ă kō’lī) is approximately 2 mm long, but its Archaeal DNA is similar but wrapped ○ Diploid Nature : Eukaryotic cells around histones. are often diploid, meaning they Exceptions: have two copies of each ○ Epulopiscium bacteria can have chromosome. hundreds or thousands of chromosomes. ○ Linear Chromosomes : Eukaryotic nuclear chromosomes are linear and ○ Some bacteria have linear contained within a nucleus, unlike chromosomes (e.g. many prokaryotic chromosomes Agrobacterium tumefaciens with which are circular. one circular and one linear chromosome). ○ Nuclear Envelope : The nucleus is surrounded by a double membrane Plasmids known as the nuclear envelope. Small circular DNA molecules Histones and Chromatin separate from the chromosome. ○ - Eukaryotic chromosomes have Replicate independently of the histones, which are proteins that chromosome. help in the packaging of DNA. Usually 1-20% the size of a chromosome (few thousand to few ○ - DNA wraps around histones million base pairs). forming nucleosomes, which further Carry genes for various purposes, not clump together to form chromatin essential for survival but beneficial. fibers. Types of plasmids: ○ Chromatin fibers are dispersed ○ Fertility (F) plasmids: facilitate DNA throughout the nucleus except transfer between bacteria during mitosis when they condense (conjugation). into visible chromosomes. ○ Resistance (R) plasmids: carry genes for resistance to antibiotics or Euchromatin and Heterochromatin: heavy metals. ○ Euchromatin: Loosely packed ○ Bacteriocin plasmids: carry genes chromatin where genes are active. for toxins that kill competing bacteria. ○ Heterochromatin: Tightly packed ○ Virulence plasmids: carry genes for chromatin where genes are inactive. structures/enzymes/toxins that ○ Mitosis: Before mitosis, make bacteria pathogenic. chromosomes replicate and The Structure of Eukaryotic condense into pairs, becoming Genomes visible under light microscopy. Each daughter cell receives one copy of Nuclear Chromosomes each chromosome. ○ Multiple Chromosomes: Eukaryotic cells typically contain more than one nuclear chromosome. Some cells, like mammalian red blood cells, lose their chromosomes as they mature. Extranuclear Chromosomes in ○ Monomers and Energy : The Eukaryotes building blocks of DNA are deoxyribonucleotides, which provide Mitochondria and Chloroplasts: both the necessary components and ○ Both organelles contain their own energy for polymerization. chromosomes. Bacterial DNA Replication ○ Mitochondrial chromosomes are ○ Origin of Replication : Replication circular; chloroplast chromosomes begins at a specific nucleotide are now known to be linear. sequence called the origin. ○ These extranuclear chromosomes ○ DNA Helicase : Unzips the DNA resemble prokaryotic chromosomes. molecule by breaking hydrogen bonds, forming a replication fork. Gene Coding: ○ Extranuclear chromosomes code for ○ - DNA Polymerase : Binds to each about 5% of the RNA and proteins strand and synthesizes new DNA required by the organelles; the strands in a 5' to 3' direction. DNA remaining 95% is coded by nuclear polymerase III is primarily involved DNA. in bacterial replication. ○ Functional proteins in mitochondria Leading and Lagging Strands : and chloroplasts often require ○ Leading Strand: Synthesized polypeptides encoded by both continuously towards the replication nuclear and organellar DNA. fork. ○ Plasmids: Some eukaryotes like ○ Lagging Strand: Synthesized fungi, algae, and protozoa may discontinuously away from the contain plasmids. replication fork in short segments called Okazaki fragments. ○ RNA Primers : Synthesized by Summary of Genome Structure primase to provide starting points ○ Prokaryotic Genome : Consists of for DNA polymerase. haploid chromosomal DNA and extrachromosomal DNA (plasmids). ○ Proofreading : DNA polymerase III has a proofreading function to ○ Eukaryotic Genome : Comprises correct errors during synthesis. nuclear chromosomal DNA (in multiple linear chromosomes) and ○ extranuclear DNA (in mitochondria, chloroplasts, and any plasmids). Eukaryotic DNA Replication Basics of DNA Replication ○ - Different DNA Polymerases: ○ Semiconservative Process : Each Eukaryotic cells utilize different DNA daughter DNA molecule consists of polymerases for various replication one original strand and one new tasks. strand. ○ Multiple Origins of Replication: Due ○ Translation : The process where to the large size of eukaryotic ribosomes synthesize polypeptides chromosomes, replication begins at using the RNA transcript. multiple origins. ○ Exceptions : Some RNA viruses ○ Shorter Okazaki Fragments: reverse transcribe RNA to DNA. Eukaryotic Okazaki fragments are shorter (100-400 nucleotides). Types of RNA Transcribed from DNA: ○ Methylation: Eukaryotic cells primarily methylate cytosine bases. 1. RNA primer molecules : Utilized by DNA polymerase during DNA replication. 2. Messenger RNA (mRNA) : Carries Termination and Additional genetic information from chromosomes Processes to ribosomes. ○ Bidirectional Replication: In bacteria, replication is bidirectional, 3. Ribosomal RNA (rRNA) : Combines proceeding from a single origin with with ribosomal polypeptides to form two replication forks. ribosomes. ○ Methylation Roles : 4. Transfer RNA (tRNA) : Delivers the correct amino acids to ribosomes based - Genetic expression control on mRNA sequences. - Initiation of DNA replication 5. Regulatory RNA molecules : Interact with DNA to control gene - Protection against viral infection expression. - DNA repair 6. Ribozymes : RNA molecules that function as metabolic enzymes. Genotype and Phenotype ○ Genotype: The set of genes in an Transcription in Bacteria: organism's genome, including DNA ○ Occurs in the nucleoid region of the sequences that carry instructions for cytoplasm. life. ○ Three steps of RNA transcription: ○ Phenotype : The physical and functional traits of an organism, 1. Initiation: RNA polymerase determined by the expression of its binds to DNA promoters, with genotype. sigma factor aiding in recognition. 2. Elongation: RNA polymerase synthesizes RNA by adding Central Dogma of Genetics ribonucleotides in a 5’ to 3’ ○ Transcription : The process of direction. copying genetic information from DNA to RNA. 3. Termination: Release of RNA -tRNA : Delivers amino acids to polymerase and the RNA transcript ribosomes; each tRNA has an from DNA. anticodon complementary to an mRNA codon. -rRNA : Forms part of the Differences in Transcription ribosome structure. between Prokaryotes and Eukaryotes: ○ Location : Eukaryotic transcription occurs in the nucleus; prokaryotic in Genetic Code: the cytosol. ○ Codons : Triplets of mRNA nucleotides that specify amino acids. ○ RNA Polymerase Types : Eukaryotes have three types for ○ Redundancy : Multiple codons can different RNAs, whereas prokaryotes code for the same amino acid, use one. aiding in error tolerance. ○ Processing of mRNA : Eukaryotic mRNA undergoes capping, Ribosome Structure and polyadenylation, and splicing, unlike Function: prokaryotic mRNA. ○ Prokaryotic ribosomes : 70S, composed of 50S and 30S subunits. RNA Processing in Eukaryotes: ○ Eukaryotic ribosomes : 80S, composed of 60S and 40S subunits. 1. Capping : Addition of a modified guanine nucleotide to the 5’ end. ○ Binding Sites : 2. Polyadenylation : Addition of 100- -A site : Accommodates tRNA with 250 adenine nucleotides to the 3’ end. amino acid. 3. Splicing : Removal of introns and -P site : Holds tRNA with growing joining of exons to produce functional polypeptide. mRNA. -E site : Exit site for discharged tRNA. Translation: ○ Process : Ribosomes synthesize Termination of Transcription: polypeptides using mRNA sequences. ○ Self-termination : Formation of a ○ Participants : hairpin loop in the RNA sequence causing RNA polymerase to release. -mRNA : Carries codons (triplets) encoding amino acids. ○ Rho-dependent termination : Rho protein interacts to release RNA polymerase and RNA transcript. Key Differences in Transcription 2. Elongation : and Translation: ○ Transcription and translation in ○ A cyclical process involving prokaryotes : Occur sequential addition of amino acids simultaneously in the cytosol. to a growing polypeptide chain at the P site. ○ Transcription and translation in eukaryotes : Transcription occurs ○ Key Steps: in the nucleus, translation in the tRNA complementary to the next cytoplasm. codon delivers its amino acid to the A site. mRNA Differences: A ribozyme in the larger subunit ○ Prokaryotic mRNA : Can encode forms a peptide bond between the multiple polypeptides. terminal amino acid and the newly introduced amino acid. ○ Eukaryotic mRNA : Encodes a single polypeptide and requires Ribosome moves one codon down processing before translation. the mRNA, transferring tRNA from P to E site and A site to P site. Empty tRNA is released from the E Events in Translation site and recharged in the cytosol. Stages of Translation : Cycle repeats, adding amino acids 1. Initiation : at a rate of about 15 per second. ○ Ribosomal subunits, mRNA, protein Ribosomal movement exposes the factors, and tRNAfMet form an start codon, allowing polyribosome initiation complex. formation. ○ In prokaryotes, this may occur during transcription. 3. Termination : ○ - Key Steps: ○ Involves release factors recognizing Smaller ribosomal subunit stop codons and modifying the attaches to mRNA at the Shine- ribosomal subunit to sever the Dalgarno sequence, positioning polypeptide from the final tRNA. the start codon (AUG) at the P site. ○ The ribosome dissociates into its subunits. tRNAfMet (anticodon UAC) attaches to the P site. ○ Polypeptides may function alone or in quaternary structures. Larger ribosomal subunit attaches to complete the initiation complex. Eukaryotic Translation binds to CAP. CAP enhances RNA Differences : polymerase binding to the promoter. ○ Initiation occurs when the small ribosomal subunit binds to the 5’ ○ - Repression and Induction : guanine cap. Regulatory gene produces a repressor that binds to the operator, ○ First amino acid is methionine blocking transcription. Presence of rather than formylmethionine. lactose converts it to allolactose, inactivating the repressor and ○ Ribosomes attached to ER allowing transcription. synthesize polypeptides into the ER cavity. ○ Archaeal translation is more similar 2. Repressible Operons : to eukaryotes but lacks ER. ○ - Typically active until deactivated by repressors. Regulation of Genetic ○ - Example: Tryptophan (trp) Expression Operon in E. coli: General Regulation : ○ Some genes are constantly ○ - Regulatory gene codes for an expressed for essential functions. inactive repressor. ○ Other genes are regulated to ○ - Tryptophan binds to and conserve energy, producing activates the repressor, which then polypeptides only in response to binds to the operator, halting environmental changes. transcription. ○ Regulation methods include controlling transcription RNA Molecules in Regulation : initiation/termination or translation. ○ microRNA (miRNA) : Binds to mRNA to block translation or cut mRNA, regulating gene expression. Prokaryotic Operons : ○ small interfering RNA (siRNA) : 1. Inducible Operons : Double-stranded RNA that binds to and cuts target nucleic acid. ○ Usually inactive, requiring inducers to activate. ○ Riboswitches : RNA molecules that change shape in response to ○ Often code for proteins involved in environmental conditions, affecting nutrient transport and catabolism. translation. ○ Example: Lactose (lac) Operon in Mutations of Genes E. coli: ○ Types of Mutations : ○ Positive Regulation by CAP : Low glucose levels increase cAMP, which Point Mutations : Single nucleotide Frequency of Mutation changes (substitutions, insertions, ○ Rarity of Mutations : deletions). ○ Mutations are rare, occurring in Substitution : Can be silent, about 1 in every 10 million (10^7) missense, or nonsense mutations. genes. Frameshift Mutations : Insertions ○ Frequent mutations would hinder or deletions causing shifts in the organism survival and reproduction. reading frame. Gross Mutations : Inversions, duplications, or transpositions. Impact of Mutagens : ○ Mutagens increase mutation rates by 10 to 1000 times, causing mutations in 1 in every 10^6 to Effects of Point Mutations : 10^4 genes. ○ Silent Mutations : No change in the amino acid sequence due to code redundancy. ○ Deleterious and Beneficial ○ Missense Mutations : Change in Mutations : one amino acid, potentially altering protein function. ○ Most mutations are harmful, coding for nonfunctional proteins or halting ○ Nonsense Mutations : Creation of transcription, leading to cell death. a stop codon, leading to truncated proteins. ○ Occasionally, beneficial mutations occur, allowing cells to survive and reproduce, contributing to evolution (e.g., rifampin-resistant tuberculosis Mutagens : bacteria). ○ Radiation : Ionizing (X rays, gamma rays) causing breaks in DNA, and nonionizing (UV light) causing pyrimidine dimers. DNA Repair Mechanisms ○ Direct Repair : - Chemical Mutagens : ○ Base-Excision Repair : Specific ○ Nucleotide Analogs : Incorporated enzymes replace incorrect bases into DNA, causing mispairing. (e.g., replacing hypoxanthine with adenine). - Nucleotide-Altering Chemicals : Alter nucleotide structure, e.g., ○ Light Repair : Photolyase enzyme aflatoxins, nitrous acid. breaks pyrimidine dimers formed by UV light, restoring original DNA - Frameshift Mutagens : sequence. Insert/delete base pairs, e.g., benzopyrene, ethidium bromide. Single-Strand Repair : Genetic Recombination and ○ Nucleotide-Excision Repair : Horizontal Gene Transfer Removes a damaged DNA section Genetic Recombination : and uses the complementary strand ○ Exchange of nucleotide sequences to repair the gap. between DNA molecules via homologous sequences, resulting in ○ Mismatch Repair : Corrects errors recombinant DNA. from replication by recognizing new, unmethylated DNA strands and fixing mismatched bases. Horizontal Gene Transfer (HGT) : ○ Transformation : Uptake of environmental DNA by a recipient Error-Prone Repair : cell. ○ SOS Response : In severe damage, E. coli produces DNA polymerases ○ Transduction : DNA transfer via IV and V, replicating damaged DNA bacteriophages. with low fidelity, introducing many new mutations but allowing some ○ Bacterial Conjugation : DNA cells to survive. transfer through physical contact between donor (F+ cell with sex pili) and recipient (F- cell). Identifying Mutants, Mutagens, and Carcinogens ○ Positive Selection : Transposons and Transposition - Selecting mutants by Transposons : eliminating wild-type cells (e.g., ○ DNA segments that move within or isolating penicillin-resistant between DNA molecules. bacteria on penicillin-containing ○ Insertion Sequences : Simplest media). transposons with inverted repeats ○ Negative (Indirect) Selection : and a transposase gene. Identifies auxotrophic mutants by ○ Complex Transposons : Contain comparing growth on media with additional genes, such as those for and without specific nutrients antibiotic resistance. (e.g., isolating tryptophan auxotrophs using replica plating). ○ Ames Test: Mechanism of Transposition : Screens for mutagens using ○ Transposase enzyme cuts and histidine auxotrophic Salmonella. inserts the transposon into a target Reversion to wild-type phenotype DNA site, sometimes duplicating the (histidine synthesis) indicates target site. mutagenicity.