Bio1050 Exam 3 Lecture Notes PDF
Document Details
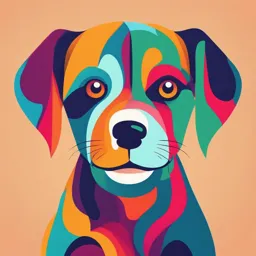
Uploaded by StrongestWalnutTree310
Wayne State University
Michaela Baho
Tags
Summary
These lecture notes cover the concepts of DNA and nucleotides, including their structure, function, and base pairings. The notes contain multiple-choice questions and explanations to help students understand the material.
Full Transcript
EXAM 3 CONTENT Bio1050 L12 241016 Cv.pptx 2.8) Describe and make a labeled drawing of the structure of deoxyribonucleotides ribonucleotides a single DNA nucleic acid (also known as a DNA molecule, DNA strand or DNA polymer) and two strands of DNA (a double helix). UNIT THEME - DNA Sections 3.15,...
EXAM 3 CONTENT Bio1050 L12 241016 Cv.pptx 2.8) Describe and make a labeled drawing of the structure of deoxyribonucleotides ribonucleotides a single DNA nucleic acid (also known as a DNA molecule, DNA strand or DNA polymer) and two strands of DNA (a double helix). UNIT THEME - DNA Sections 3.15, 10.2, 10.3 in Biology Concepts and Connections, 10th Edition Slide 1 explanations: In biology, the prime symbol (′) is a marker used to label specific parts of the sugar molecule in nucleotides (the building blocks of DNA and RNA). It distinguishes the carbon atoms in the sugar from those in the nitrogenous base. The sugar carbons are numbered 1′ to 5′: 1′ carbon: Attached to the nitrogenous base. 2′ carbon: Determines the sugar type: ○ RNA has a hydroxyl group (OH) at the 2′ position. ○ DNA has a hydrogen atom (H) at the 2′ position (hence "deoxy"). 3′ carbon: Has a hydroxyl group (OH) that forms bonds with the next nucleotide. 5′ carbon: Attached to a phosphate group. The 5′ end of DNA or RNA strands has a phosphate group, and the 3′ end has a hydroxyl group. This orientation is critical for processes like DNA replication and RNA synthesis. Multiple Choice Questions 1. What does the prime symbol (′) represent in biology? a) The position of phosphate groups b) The numbering of carbons in the sugar molecule c) The attachment site for amino acids d) The position of nitrogen in the nitrogenous base (Correct answer: b) Why it's b: The prime symbol is used specifically to label the carbons in the sugar molecule to distinguish them from the carbons in the nitrogenous base. Why not a, c, or d: ○ a) Phosphate groups attach to the sugar molecule but are not labeled with the prime symbol. ○ c) Amino acids are not involved in the structure of nucleotides. ○ d) The nitrogenous base has carbons that are numbered differently, without the prime symbol.. 2. Which carbon in the sugar molecule is attached to the nitrogenous base? a) 2′ carbon b) 3′ carbon c) 1′ carbon d) 5′ carbon (Correct answer: c) Why it's c: The nitrogenous base (A, T, C, G, or U) always attaches to the 1′ carbon of the sugar molecule. Why not a, b, or d: a) The 2′ carbon differentiates DNA and RNA but does not attach to the base. b) The 3′ carbon forms a bond with the next nucleotide in the chain. d) The 5′ carbon is where the phosphate group attaches. 3. What group is found on the 2′ carbon in RNA but not in DNA? a) Phosphate group b) Hydrogen atom c) Hydroxyl group (OH) d) Nitrogenous base (Correct answer: c) Why it's c: The nitrogenous base (A, T, C, G, or U) always attaches to the 1′ carbon of the sugar molecule. Why not a, b, or d: a) The 2′ carbon differentiates DNA and RNA but does not attach to the base. b) The 3′ carbon forms a bond with the next nucleotide in the chain. d) The 5′ carbon is where the phosphate group attaches. 4. What is attached to the 5′ carbon of the sugar molecule? a) Nitrogenous base b) Hydroxyl group (OH) c) Phosphate group d) Another nucleotide (Correct answer: c) Why it's c: RNA has a hydroxyl group (OH) at the 2′ carbon, while DNA has only a hydrogen atom (H) there, which makes it "deoxy" (lacking oxygen). Why not a, b, or d: a. a) Phosphate groups attach to the 5′ carbon, not the 2′ carbon. b. b) A hydrogen atom is found on the 2′ carbon in DNA, not RNA. c. d) Nitrogenous bases attach to the 1′ carbon, not the 2′ carbon. 5. Which end of a DNA strand has a free hydroxyl group? a) 1′ end b) 2′ end c) 3′ end d) 5′ end (Correct answer: c) Why it's c: The 3′ carbon has a free hydroxyl group (OH) that is essential for forming bonds with the next nucleotide during DNA replication or synthesis. Why not a, b, or d: a) The 1′ carbon is attached to the nitrogenous base, not the strand's end. b) The 2′ carbon has either an OH group in RNA or an H in DNA, but it’s not the strand's end. d) The 5′ end has a free phosphate group, not a hydroxyl group. 6. Why is the prime symbol (′) used in nucleotide structures? a) To label phosphate groups b) To identify carbons in the sugar molecule separately from the nitrogenous base c) To show the direction of hydrogen bonding d) To mark the position of enzymes (Correct answer: b) Why it's b: The prime symbol is necessary to distinguish the sugar's carbon atoms from the nitrogenous base's carbon atoms. Why not a, c, or d: a. a) Phosphate groups are not labeled with prime numbers. b. c) Hydrogen bonding involves nitrogenous bases, not the sugar molecule. c. d) Enzymes bind to DNA but are not labeled with the prime symbol. 7. What is the orientation of two DNA strands in a double helix? a) Parallel b) Antiparallel c) Identical d) Random (Correct answer: b) Why it's b: The two strands of DNA run in opposite directions, with one going 5′ to 3′ and the other 3′ to 5′. This is called an antiparallel orientation. Why not a, c, or d: a. a) Parallel would mean both strands run in the same direction, which is incorrect. b. c) Identical strands would mean the base sequence is the same, which is not true. c. d) Random orientation does not describe DNA’s precise structure. Slide 2: explanation: 1. Phosphate Group (Yellow Circle): ○ The phosphate group is part of the sugar-phosphate backbone of DNA. ○ It connects to the 5′ and 3′ carbons of the sugar molecule to form a strong covalent bond. ○ The phosphate backbone provides structural stability to the DNA molecule. 2. Deoxyribose Sugar (Blue Pentagon): ○ The sugar is deoxyribose (part of the nucleotide structure). ○ The carbons in the sugar are labeled 1′ to 5′. 1′: Attached to the nitrogenous base. 5′: Attached to the phosphate group. 3. Nitrogenous Bases (Green and Orange Shapes): ○ The bases pair specifically via hydrogen bonds: Adenine (A) pairs with Thymine (T) using 2 hydrogen bonds (green pair). Guanine (G) pairs with Cytosine (C) using 3 hydrogen bonds (orange pair). ○ These specific base-pairing rules are known as Chargaff's rules. 4. Hydrogen Bonds (Pink Dotted Lines): ○ Hydrogen bonds are weak interactions between complementary nitrogenous bases. ○ They allow the two strands of DNA to stay connected while still being flexible enough to separate during processes like DNA replication and transcription. 5. Antiparallel Strands: ○ The two DNA strands run in opposite directions: One strand is oriented 5′ to 3′, and the other runs 3′ to 5′. ○ This antiparallel structure is essential for the proper functioning of DNA. Key Points to Note Covalent Bonds: Found in the sugar-phosphate backbone; they are strong and provide stability to the DNA molecule. Hydrogen Bonds: Found between the nitrogenous bases; they are weaker, allowing DNA strands to unzip during replication or transcription. Complementary Base Pairing: Ensures genetic information is accurately copied and transcribed. Slide 3 explanation Key Components 1. DNA Double Helix (Left Side) ○ DNA is depicted as a twisted ladder-like structure. ○ The sides of the ladder represent the sugar-phosphate backbone. ○ The rungs of the ladder are the base pairs, which are held together by hydrogen bonds. 2. Hydrogen Bonds (Middle) ○ Definition: Weak interactions between nitrogenous bases on opposite strands of DNA. ○ Base Pairing Rules: Adenine (A) pairs with Thymine (T) using 2 hydrogen bonds. Cytosine (C) pairs with Guanine (G) using 3 hydrogen bonds. ○ The pink dotted lines in the image represent these hydrogen bonds. 3. Base Pairing and Orientation (Middle Detailed View) ○ Each base (A, T, G, C) is attached to a deoxyribose sugar (blue pentagons). ○ The sugars are connected by phosphate groups (yellow circles), forming the backbone of DNA. ○ The strands are antiparallel: One strand runs 5′ to 3′, while the complementary strand runs 3′ to 5′. 4. Hands Analogy (Bottom Left) ○ The hands represent base pairing: One hand (e.g., thumb and forefinger) fits perfectly into the other hand, symbolizing how A fits with T and C fits with G. ○ This visual analogy helps explain how the specific shapes and hydrogen bonding rules ensure proper pairing. 5. Space-Filling Model (Right Side) ○ Shows the three-dimensional structure of the DNA double helix. ○ Highlights the compact and organized nature of DNA as it appears in real life. Key Takeaways Hydrogen Bonds: Critical for holding DNA strands together while allowing them to unzip easily during replication or transcription. Complementary Base Pairing: Ensures accurate copying of genetic information (A with T, G with C). Antiparallel Strands: Essential for the double helix structure and DNA's functions. Slide 4 explanation 1. One Strand is Upside Down Antiparallel Orientation: ○ DNA strands run in opposite directions. ○ One strand is oriented 5′ to 3′, while the complementary strand is oriented 3′ to 5′. ○ The terms 5′ and 3′ refer to the carbons in the sugar molecule: The 5′ end has a free phosphate group. The 3′ end has a free hydroxyl (OH) group. 2. Base Pairs and Hydrogen Bonds Base Pairing Rules: ○ Adenine (A) pairs with Thymine (T) through 2 hydrogen bonds. ○ Cytosine (C) pairs with Guanine (G) through 3 hydrogen bonds. Hydrogen Bonds: ○ Represented by pink dotted lines, these weak bonds hold the two strands together. ○ Their flexibility allows the strands to separate during DNA replication and transcription. 3. Sugar-Phosphate Backbone The yellow circles (phosphate groups) and blue pentagons (deoxyribose sugar) form the sugar-phosphate backbone. The backbone provides structural stability to DNA and connects each nucleotide to the next via covalent bonds. 4. DNA Double Helix (Right Side) The double helix is a twisted ladder-like structure formed by the two antiparallel strands. The twisting maximizes DNA's stability and protects the bases from damage. 5. Key Takeaways Antiparallel Strands: ○ The "upside-down" orientation of one strand relative to the other is essential for DNA's functionality. Base Pairing: ○ The specificity of A-T and C-G pairing ensures accurate replication and transcription. Structural Stability: ○ The sugar-phosphate backbone provides strength, while hydrogen bonds allow flexibility. Interactive Activity The text, “Make a double helix with your neighbors,” suggests using physical models (or hands) to mimic how the DNA strands pair and twist. Slide 5 explanation 1. Antiparallel Strands The DNA strands are antiparallel, meaning they run in opposite directions: ○ One strand runs 5′ to 3′, and the complementary strand runs 3′ to 5′. ○ The 5′ end has a free phosphate group (P). ○ The 3′ end has a free hydroxyl group (OH) on the sugar molecule. This alignment is critical for DNA replication and enzymatic activity. 2. Base Pairing The rungs of the DNA ladder are formed by complementary nitrogenous bases: ○ Adenine (A) pairs with Thymine (T) using 2 hydrogen bonds (green). ○ Cytosine (C) pairs with Guanine (G) using 3 hydrogen bonds (orange). Hydrogen bonds: ○ Represented by dashed lines, they are weaker than covalent bonds, allowing DNA strands to unzip during replication or transcription. 3. Sugar-Phosphate Backbone The phosphate groups (yellow circles) and deoxyribose sugars (blue pentagons) form the sugar-phosphate backbone. The backbone provides structural stability and is connected by strong covalent bonds. 4. Carbon Numbering in the Sugar Molecule The carbons in the sugar are labeled 1′ to 5′: ○ 1′ Carbon: Attached to the nitrogenous base. ○ 3′ Carbon: Has a hydroxyl (OH) group that forms bonds with the next nucleotide. ○ 5′ Carbon: Attached to the phosphate group. 5. Key Takeaways Antiparallel Alignment: Ensures that DNA can replicate and function correctly. Base Pairing Rules: ○ A pairs with T (2 hydrogen bonds). ○ C pairs with G (3 hydrogen bonds). Structural Stability: The sugar-phosphate backbone provides strength, while hydrogen bonds allow flexibility and separation. Slide 6 explanation 1. Straight Ladder-Like DNA (Leftmost Image) Structure: ○ The DNA is initially shown as a simple ladder with: Sugar-phosphate backbones forming the sides of the ladder. Nitrogenous base pairs forming the rungs. Base Pairing: ○ Adenine (A) pairs with Thymine (T) through 2 hydrogen bonds. ○ Cytosine (C) pairs with Guanine (G) through 3 hydrogen bonds. 2. Beginning of the Twist (Middle Images) Formation of the Helix: ○ The ladder begins to twist into a helical shape. ○ This twisting is driven by: Hydrophobic interactions: Bases stack to avoid water. Electrostatic forces: Stabilization from interactions between the sugar-phosphate backbone and the bases. ○ The helical twist optimizes the stability and compactness of DNA. 3. Double Helix (Rightmost Image) Final Structure: ○ The double helix consists of two antiparallel strands running in opposite directions: One strand runs 5′ to 3′. The other strand runs 3′ to 5′. ○ The strands are twisted around an imaginary axis, forming a right-handed helix. Twist Details: ○ The twist creates major and minor grooves, which are crucial for interactions with proteins during replication and transcription. Key Features of the Double Helix Hydrogen Bonds: ○ Weak bonds between complementary base pairs hold the two strands together. ○ These bonds are flexible, allowing the strands to separate when needed. Sugar-Phosphate Backbone: ○ The strong covalent bonds in the backbone provide structural support. Compactness: ○ The twist allows the DNA to fit into the cell nucleus while maintaining its function. Significance of the Helix Stability: ○ The double helix is a stable structure, protecting the genetic information. Functionality: ○ The structure allows for: Accurate replication. Interaction with proteins for gene expression. Versatility: ○ DNA can coil further into chromosomes for efficient storage. Slide 7 explanation 1. Central Theme The Genetic Code in DNA: ○ DNA contains sequences of bases (A, T, C, G) that form a code. ○ This code determines the order of amino acids in a protein, which ultimately defines the protein’s structure and function. 2. DNA Double Helix Several images of DNA show its double helix structure, which holds genetic information in the form of nitrogenous base sequences. Key features: ○ DNA is a tightly coiled structure, compact enough to fit into the nucleus. ○ The sequence of base pairs (A-T and C-G) is unique to every organism and dictates the genetic code. 3. Chromosomes (Top Right) Chromosomes: ○ DNA is organized into chromosomes within the cell nucleus. ○ The image shows human chromosomes (karyotype), which are pairs of DNA molecules condensed and visualized during cell division. ○ Humans have 23 pairs of chromosomes, with one set inherited from each parent. ○ Each chromosome contains thousands of genes coding for different proteins. 4. Protein Synthesis (Bottom Left) Connection Between DNA and Amino Acids: ○ DNA is transcribed into messenger RNA (mRNA) in the nucleus. ○ mRNA is then translated into amino acid sequences by ribosomes in the cytoplasm. ○ This process occurs within the cell, as shown in the cell diagram with the nucleus and ribosomes highlighted. The Genetic Code: ○ Groups of three DNA bases (codons) code for specific amino acids. 5. Molecular Structure Hands Analogy: ○ The "hands" holding molecules represent how the bases in DNA pair: A pairs with T, and C pairs with G. These pairings are crucial for accurate DNA replication and protein synthesis. Molecular Models: ○ The colorful 3D representations show the precise atomic structure of the DNA molecule. 6. Key Takeaways DNA as a Blueprint: ○ The code in DNA determines the exact order of amino acids in proteins. Protein Function: ○ Proteins perform most cellular functions, including enzymes, structural components, and signaling molecules. Chromosomes as Carriers: ○ DNA is packed into chromosomes, ensuring efficient storage and accurate transfer of genetic information during cell division. Iclicker explanation: In double-stranded DNA, Adenine (A) always pairs with (T)** through 2 hydrogen bonds, following the rules of complementary base pairing. Cytosine (C) pairs with Guanine (G) through 3 hydrogen bonds, which are slightly stronger than the A-T pairing. Uracil (U) replaces Thymine (T) in RNA but is not present in DNA. Adenine does not pair with itself or with Cytosine or Guanine, making Thymine its specific complementary base in DNA. These pairing rules ensure the stability and accurate replication of the DNA molecule. Pyrimidines are one of the two types of nitrogenous bases found in nucleic acids and are characterized by their single-ring structure. The pyrimidines include Cytosine (C), which is found in both DNA and RNA; Thymine (T), found exclusively in DNA; and Uracil (U), which replaces Thymine in RNA. These bases are distinct from purines, which have a double-ring structure and include Adenine (A) and Guanine (G). Pyrimidines pair specifically with purines through hydrogen bonds: Cytosine pairs with Guanine, and Thymine (or Uracil in RNA) pairs with Adenine. This complementary pairing is essential for the stability and function of DNA and RNA molecules. In double-stranded DNA, the two strands are held together by hydrogen bonds between complementary nitrogenous bases, making e) hydrogen bonds the correct answer. Hydrogen bonds are weak interactions that provide just enough stability to hold the strands together while allowing them to separate during processes like DNA replication and transcription. For example, Adenine (A) pairs with Thymine (T) through two hydrogen bonds, while Cytosine (C) pairs with Guanine (G) through three hydrogen bonds, which are slightly stronger. The other options are incorrect because the backbone of DNA is formed by covalent bonds, not hydrogen bonds; dehydration and hydrolysis reactions are involved in forming and breaking down macromolecules, respectively, but do not hold the DNA strands together. Water plays no direct role in binding the DNA strands. The hydrogen bonds' strength and flexibility are key to DNA's function and stability. a) 3′ is at the top. Correct: This refers to the left strand, which has its 3′ end (sugar end) at the top as stated in the question. DNA strands are antiparallel, so the top of the left strand is 3′. b) 5′ is at the top. Incorrect: The right strand has its 5′ end at the top due to the antiparallel orientation, but the question asks about the left strand’s position, which is 3′. c) Either 3′ or 5′ can be at the top. Incorrect: DNA strands are always antiparallel, meaning the orientation is fixed. The top of one strand is always 3′ while the complementary strand’s top is 5′. There is no flexibility here. d) A phosphate end is at the top. Correct: The phosphate group is attached to the 5′ carbon of the sugar, so the 5′ end at the top of the right strand has a phosphate group. This matches the description of the antiparallel structure. e) The nucleotide G is at the top. Incorrect: The position of specific nucleotides, such as guanine (G), depends on the sequence, which is not provided in the question. This answer is unrelated to the strand’s orientation. 1. Carbohydrates (Top Left) Monomers: Monosaccharides (simple sugars like glucose). Structure: ○ Represented by rings (monosaccharides) or chains (polysaccharides). ○ Monosaccharides are linked by glycosidic bonds to form larger molecules like: Cellulose: Structural polysaccharide in plants. Starch and glycogen: Energy storage polysaccharides. Function: Energy source, structural support (e.g., in cell walls). 2. Proteins (Top Right) Monomers: Amino acids. Structure: ○ Each amino acid consists of: An amino group (-NH₂). A carboxyl group (-COOH). A variable R group (determines the properties of the amino acid). ○ Amino acids are linked by peptide bonds to form polypeptides and proteins. Function: ○ Proteins have diverse roles, including enzyme activity, structural support, signaling, and transport. 3. Fats and Lipids (Bottom Left) Monomers: Fatty acids and glycerol. Structure: ○ Composed of long hydrocarbon chains (fatty acids) attached to glycerol. ○ Linked by ester bonds to form: Triglycerides: Used for energy storage. Phospholipids: Form the lipid bilayer of cell membranes. Function: ○ Energy storage, insulation, and forming the cell membrane. 4. Nucleic Acids (Bottom Right) Monomers: Nucleotides. Structure: ○ A nucleotide consists of: A phosphate group (yellow circle). A sugar: Deoxyribose in DNA. Ribose in RNA. A nitrogenous base: DNA: Adenine (A), Thymine (T), Cytosine (C), Guanine (G). RNA: Uracil (U) replaces Thymine. ○ Nucleotides are linked by phosphodiester bonds to form DNA and RNA strands. Function: ○ Store and transmit genetic information (DNA). ○ Synthesize proteins (RNA). Key Takeaways: Carbohydrates: Quick energy and structural roles. Proteins: Versatile molecules for structure and function. Lipids: Energy storage and membrane formation. Nucleic Acids: Genetic information storage and transmission. 1. Carbohydrates Examples: Glucose, glycogen, starch. Function: ○ Provide a quick and primary source of energy for cells. ○ Serve as energy storage molecules: Glucose: Immediate energy source. Glycogen: Energy storage in animals. Starch: Energy storage in plants. 2. Lipids Examples: Phospholipids, triglycerides. Function: ○ Form the cell membrane (phospholipids). ○ Serve as long-term energy storage (triglycerides). ○ Provide insulation and protection for organs. 3. Proteins Examples: Insulin, glucose channels, enzymes. Function: ○ Perform a variety of roles in the body: Insulin: Regulates blood sugar levels. Glucose channels: Facilitate the transport of glucose across cell membranes. Enzymes: Speed up chemical reactions, essential for metabolism. 4. Nucleic Acids Examples: DNA, RNA. Function: ○ Store and transmit genetic information: DNA: Long-term genetic storage. RNA: Plays a role in protein synthesis. ○ Direct the synthesis of proteins by encoding genetic instructions. Key Takeaways: Each macromolecule has a distinct structure and function critical to life: Carbohydrates: Energy. Lipids: Storage and membranes. Proteins: Structural and functional roles. Nucleic Acids: Genetic information and protein synthesis Learning Objective 2.09 Use the codon chart to predict sequences. 1. Transcription (DNA → RNA): Location: Occurs in the nucleus. Process: ○ DNA is used as a template to synthesize a complementary strand of messenger RNA (mRNA). ○ Enzyme involved: RNA polymerase. ○ mRNA carries the genetic code from the DNA out of the nucleus and into the cytoplasm. Purpose: Converts genetic information in DNA into a form that can be read and used to build proteins. 2. RNA in the Cytoplasm: After transcription, the mRNA exits the nucleus and enters the cytoplasm, where it serves as the blueprint for protein synthesis. 3. Translation (RNA → Protein): Location: Occurs in the cytoplasm, specifically at the ribosome. Process: ○ Ribosomes read the mRNA sequence in groups of three bases (called codons). ○ Each codon specifies a particular amino acid. ○ Transfer RNA (tRNA) molecules bring the correct amino acids to the ribosome, where they are linked together to form a protein. Purpose: Converts the genetic code in RNA into a sequence of amino acids, which fold into functional proteins. 4. Protein Formation: The string of amino acids created during translation folds into a specific three-dimensional shape, forming a functional protein. Proteins perform essential cellular functions, including acting as enzymes, hormones, or structural components. Key Takeaways: Transcription: DNA is converted into mRNA in the nucleus. Translation: mRNA is used to assemble amino acids into proteins in the cytoplasm. This process ensures that the genetic information stored in DNA can be expressed as functional proteins necessary for life. 1. DNA (Top Section) DNA is the genetic material located in the nucleus. It contains a sequence of nucleotides (A, T, C, G) that store the instructions for building proteins. Transcription begins when a segment of DNA is used as a template to produce a complementary RNA strand. 2. Transcription (DNA → RNA) During transcription, the DNA sequence is transcribed into RNA (specifically, mRNA). DNA bases are paired with complementary RNA bases: ○ A pairs with U (Uracil in RNA). ○ T pairs with A. ○ C pairs with G, and G pairs with C. For example, a DNA sequence like AACCGG is transcribed into an mRNA sequence UUGGCC. 3. RNA and Codons (Middle Section) The mRNA is organized into codons, which are groups of three nucleotides. Each codon specifies a particular amino acid. For example: ○ Codon UUG codes for Leucine. ○ Codon GGC codes for Glycine. 4. Translation (RNA → Protein) In the cytoplasm, ribosomes read the mRNA codons during translation. Each codon is matched to its corresponding amino acid using tRNA molecules. tRNA carries amino acids to the ribosome, where they are linked together in the correct order to form a protein. 5. Protein (Bottom Section) The sequence of amino acids forms a protein, which folds into a specific structure to perform a function in the cell. Proteins are essential for countless cellular processes, from enzyme activity to structural roles. Key Takeaways: Codons are the three-letter "words" of the genetic code. Each codon corresponds to one amino acid. Transcription converts DNA into RNA, and translation converts RNA into protein. This process ensures the accurate transfer of genetic information from DNA to functional proteins. 1. DNA Strand The process starts with the DNA strand (top, blue sequence). DNA contains the genetic instructions in the form of sequences of nucleotides (A, T, C, G). Example DNA sequence: AAC CGG CAA AAA. 2. Transcription (DNA → RNA) In the nucleus, DNA is transcribed into RNA (pink sequence). During transcription, the base pairing rules are applied: ○ A pairs with U (in RNA, T is replaced with U). ○ T pairs with A. ○ C pairs with G. ○ G pairs with C. Example RNA sequence: UUG GCC GUU UUU. 3. Codons The RNA sequence is divided into triplets, called codons. Each codon consists of three nucleotides and codes for a specific amino acid. ○ Example codons: UUG, GCC, GUU, UUU. 4. Translation (RNA → Polypeptide) In the cytoplasm, the RNA is read by ribosomes during translation. Each codon is matched with a specific amino acid using the genetic code. ○ Example: UUG = Leucine. GCC = Alanine. GUU = Valine. UUU = Phenylalanine. 5. Polypeptide Formation The amino acids specified by the codons are linked together by peptide bonds to form a polypeptide chain. The polypeptide chain folds into a functional protein that performs various roles in the cell. Key Takeaways Triplet codons are the fundamental units of the genetic code. Each codon specifies one amino acid. Transcription converts DNA into RNA, and translation converts RNA into a sequence of amino acids, creating a polypeptide. This process ensures the accurate expression of genetic information into proteins, which are essential for cellular function. 1. General Structure of Amino Acids All amino acids share these components: Central Carbon (C): The backbone of the molecule. Amino Group (NH₂): Located on the left, this makes amino acids basic. Carboxyl Group (COOH): Located on the right, this makes amino acids acidic. Hydrogen Atom (H): Attached to the central carbon. R-Group (Side Chain): Unique to each amino acid and determines its properties, such as polarity, charge, or hydrophobicity. 2. Amino Acid Examples A. Leucine (Leu): R-Group: A branched hydrocarbon chain (CH₂-CH-(CH₃)₂). Properties: ○ Nonpolar and hydrophobic. ○ Often found in the interior of proteins, away from water. Role: ○ Essential for protein structure and energy metabolism. B. Serine (Ser): R-Group: Contains a hydroxyl group (CH₂-OH). Properties: ○ Polar and hydrophilic. ○ Can participate in hydrogen bonding. Role: ○ Often involved in active sites of enzymes and phosphorylation reactions. C. Aspartic Acid (Asp): R-Group: A carboxylic acid group (CH₂-COOH). Properties: ○ Acidic and negatively charged at physiological pH. ○ Hydrophilic and soluble in water. Role: ○ Plays a role in enzyme active sites and ionic interactions in proteins. Key Takeaways R-Groups define the chemical properties of each amino acid, influencing protein structure and function. Leucine is nonpolar and hydrophobic, Serine is polar and hydrophilic, and Aspartic Acid is acidic and negatively charged. Together, amino acids form peptide bonds to create proteins, with their diverse side chains enabling complex protein functions. Sequence Determines Shape: The specific order of amino acids in a polypeptide (its sequence) determines how the protein will fold. Interactions between amino acids (like hydrogen bonds, ionic bonds, and hydrophobic interactions) create secondary structures, such as alpha-helices and beta-sheets, and eventually fold into a 3D shape (tertiary structure). Shape Determines Function: The final 3D structure of the protein is crucial for its function. Proteins must fold into the correct shape to perform specific roles in the cell, like enzymes binding to substrates, structural proteins providing support, or transport proteins carrying molecules. DNA Template Strand: The sequence in blue represents the DNA template, which is transcribed to create RNA. Transcription: RNA is synthesized based on the DNA template. The RNA sequence (pink) includes codons (sets of three nucleotides), starting with the start codon (AUG). Translation: RNA codons are translated into amino acids to form a polypeptide chain. Each codon corresponds to a specific amino acid (e.g., AUG = Methionine, UUU = Phenylalanine). Translation ends at the stop codon (e.g., UAG), completing the polypeptide chain. Directionality: DNA strands are antiparallel, meaning one strand runs from 5′ to 3′ while the complementary strand runs 3′ to 5′. In RNA transcription, the RNA sequence is complementary to the DNA template strand and follows the 5′ to 3′ direction. Notation: When writing DNA or RNA sequences, the convention is to place the 5′ end on the left and the 3′ end on the right. Amino Acid Coding: The RNA codons (e.g., GAA) are translated into specific amino acids (e.g., Glutamic acid, Glu). This highlights the flow of genetic information. This image shows the process of gene expression: 1. DNA Strands: ○ The non-coding strand serves as the template during transcription. ○ The coding strand matches the RNA sequence (except RNA uses U instead of T). 2. Transcription: ○ DNA is transcribed into mRNA in the nucleus. The mRNA sequence is complementary to the non-coding strand and identical to the coding strand (with U replacing T). 3. Translation: ○ The mRNA is translated in the cytoplasm into a polypeptide. ○ Translation starts at the start codon (AUG) and ends at the stop codon. ○ Each codon in the mRNA specifies an amino acid (e.g., Met, Lys, Phe). This is the central dogma of molecular biology: DNA → RNA → Protein. This question asks about the process where RNA's nucleotide sequence is converted into a sequence of amino acids to form a protein. Correct answer: a) Translation. Translation occurs in the cytoplasm at ribosomes, where mRNA codons are read and matched with tRNA carrying specific amino acids, forming a polypeptide chain. Transcription (option b) involves making RNA from DNA, not protein synthesis. Hydrolysis reactions (option c) break down molecules, not synthesize proteins. https://create.kahoot.it/details/6fc3319d-d0f9-4489-9de6-7746be31cb3b