Mitochondria CAS Study Notes PDF
Document Details
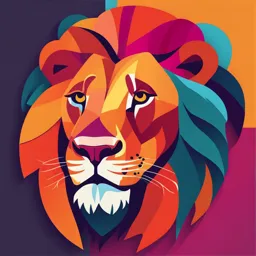
Uploaded by UserReplaceableDieBrücke
Tags
Summary
These notes detail the structure and function of mitochondria, focusing on their role in energy production within a cell. The document explains different aspects like the electron transport chain, oxidative phosphorylation, and the endosymbiotic theory.
Full Transcript
Mitochondria Resource: Molecular Biology of the Cell 9. Life requires energy Through a set of reactions that ocur in the cytosol, energy dervied from the partial oxidation of energy-rich carbohydrate molecules is used to form ATP. An efficient way to generate energy (i.e. ATP) in the cells...
Mitochondria Resource: Molecular Biology of the Cell 9. Life requires energy Through a set of reactions that ocur in the cytosol, energy dervied from the partial oxidation of energy-rich carbohydrate molecules is used to form ATP. An efficient way to generate energy (i.e. ATP) in the cells requires a membrane. For example; this could be achieved by capturing light and converting it into chemical energy through photosynthesis. Or using oxygen to produce ATP through aerobic respiration. Prokaryotes use their plasma membrane to produce ATP In eukaryotes, the plasma membrane is for transport of substances. Gram-negative Eukaryotes use the specialized membranes inside energy-converting organelles to produce most of their ATP. Prokaryotes use their plasma membrane to produce ATP. The plasma membrane is, on the other hand, reserved for transport processes in eukaryotes. Instead, specialized membranes enclosed in organelles (i.e. mitochondria) of the eukaryotic cells produce most ATP. The internal membranes provide the framework for a set of electron- transport processes that produce the ATP. Chemiosmotic Coupling Chemi: chemical bond-forming reactions that generate ATP Osmotic: membrane-transport processes The coupling occurs in two linked stages, which are performed by protein complexes embedded in the specialized membranes: Stage 1: High-energy electrons are transferred through a series of electron carriers embedded in the membrane. These electron transfers release the energy that is used to pump protons (H+) across the membrane. An electrochemical proton gradient is established. Stage 2: H+ flows back down its electrochemical gradient through a protein machine called ATP synthase which catalyzes ATP synthesis from ADP and inorganic phosphate (Pi). -membrane -a source of high energy electrons A turbine that enables the production of ATP by the proton gradient. The entire set of proteins in the membrane, together with the small molecules involved in the orderly sequence of electron transfers, is called an electron-transport chain. In biological systems, electrons are carried from one site to another by diffusible molecules that can pick up electrons at one location and deliver them to another. For mitochondria, the first of these electron carriers is NAD+. NAD+: Nicotinamide adenine dinucleotide NAD+ takes up two electrons and a H+ to become NADH. NADH: a water-soluble molecule that carries electrons from the sites where food molecules are degraded to the inner mitochondrial membrane. The mechanism of electron transport chain is analogous to an electric cell driving a current through a set of electric pumps. The free energy released as the electrons flow down the path from a Electrons released from a food high energy state to a low energy molecule while it is degraded to state drives a series of 3 H+ pumps in carbon dioxide are transferred the inner mitochondrial membrane through the membrane by a and the third H pump catalyzes the chain of electron carriers, finally transfer of the e- to oxygen. reducing oxygen to form water. Energy from chemical fuels Endosymbiotic Theory Endosymbiotic Theory Endosymbiotic theory (Lynn Margulis et al.) Mitochondria and chloroplasts (organelles) arose independently 2 billion years ago from free-living bacteria Organelles possessed attributes of aerobic respiration and photosynthesis, respectively Endosymbiotic Theory Main points of endosymbiotic theory Bacteria were engulfed by larger eukaryotic cells Beneficial symbiotic relationship developed Bacteria lost ability to function autonomously Eukaryotic cells gained oxidative respiration and photosynthesis mtDNA: Mitochondrial DNA Exists in eukaryotes as double-stranded circular DNA Smaller than DNA in chloroplasts cpDNA: Chloroplast DNA Genes encode products involved in photosynthesis and translation The Mitochondrion The Mitochondrion Has an Outer Membrane and an Inner Membrane The Mitochondrion Has an Outer Membrane and an Inner Membrane Different protein components The Mitochondrion Has an Outer Membrane and an Inner Membrane Two membranes have very different functions. Together they create two separate mitochondrial compartments: the internal matrix and the intermembrane space. Porins are abundant in the outer membrane. They are transport proteins that form large channels through the lipid bilayer. Small molecules can pass through these porins into the intermembrane space but not to the inner membrane. The major working part of the mitochondrion is the matrix and the inner membrane that surrounds it. The inner membrane is highly specialized. Matrix contains the enzymes used in the citric acid cycle. The Mitochondrion Has an Outer Membrane and an Inner Membrane The end products of the citric acid cyle in the matrix are CO2 and NADH. NADH is the main source of electrons for transport along the respiratory chain: electron transport chain in the mitochondria. The enzymes of the respiratory chain are embedded in the inner membrane. These enzymes are essential for oxidative phosphorylation which generates the majority of ATP. The inner membrane has many foldings called cristae. They increase the surface area. The number of cristae in a cardiac muscle cell are 3 times higher than a liver cell. Mitochondrial enzymes are different in different cell types. Acetyl groups of the acetyl coA are oxidized in the matrix via the citric acid cycle. Electrons are carried by the activated carrier molecules NADH. These high energy electrons are then transferred to the inner membrane, where they enter the electron transport chain. Although the citric acid cycle is considered to be part of the aerobic metabolism, it does not itself use the oxygen. O2 is directly consumed during the catabolic rxns that take place in the inner membrane. Nearly all the energy available from burning carbohydrates, fats and other food is initially saved in the form of high energy electrons removed from substrates by NAD. These electrons carried by NADH then combine with O2. The inner membrane harnesses the large amount of energy released to drive the conversion of ADP and inorganic phosphate to ATP. This is why the process is called oxidative phosphorylation. The generation of ATP by oxidative phosphorylation via the respiratory chain depends on a chemiosmotic process. In a chemical combustion rxn, most of the energy would be released as heat if hydrogen was simply burned. In biological oxidation, by contrast, most of the released energy is stored in a form useful to the cell by means of the electron-transport chain in the inner mitochondrial membrane (the respiratory chain). The energetically favorable rxn H2 + ½ O2 > H2O is made to occur in many small steps, so that most of the energy released can be stored instead of being lost to the environment as heat. The hydrogen atoms are first separated into protons and electrons. The electrons pass through a series of electron carriers in the inner mitochondrial membrane. The mitochondrion releases rest of the oxidation energy as heat. In reality, the protons and electrons are being removed from hydrogen atoms that are covalently linked to NADH. (a) The electron transport chain is a set of molecules that supports a series of oxidation-reduction reactions. (b) ATP synthase is a complex, molecular machine that uses an H+ gradient to regenerate ATP from ADP. (c) Chemiosmosis relies on the potential energy provided by the H+ gradient across the membrane. https://courses.lumenlearning.com/wm-nmbiology1/chapter/citric-acid-cycle-and-oxidative-phosphorylation/ The close association of the electron carriers with protein molecules makes oxidative phosphorylation possible. The proteins guide the electrons along the respiratory chain so that the electrons move sequentially from one enzyme complex to another. The transfer of electrons is coupled to H+ uptake and release as well as allosteric changes in energy converting protein pumps. The net result is the pumping of H+ across the inner membrane-from the matrix to the intermembrane space, driven by the energetically favorable flow of electrons. H+ movement across the inner membrane has two consequences: 1. It generates a pH gradient (ΔpH) across the inner mitochondrial membrane. pH is higher in the matrix than in the cytosol and the inner membrane. 2. It generates a voltage gradient (ΔV, membrane potential) across the inner mitochondrial membrane. The inside negative, outside positive. The Energy Derived from Oxidation Is Stored as an Electrochemical Gradient 2. ΔV attracts + ions into the matrix and push – ions out 1. ΔpH drives H+ back into the matrix and reinforces the effect of ΔV. The Proton Gradient Drives ATP Synthesis The electrochemical proton gradient across the inner mitochondrial embrane drives ATP synthesis-oxidative phosphorylation. As a high-energy electron is passed along the electron transport chain, some of the energy released drives the three respiratory enzyme complexes that pump H+ out of the matrix. The resulting electrochemical proton gradient across the inner membrane drives H+ back through the ATP synthase: a transmembrane protein that uses the energy of the H+ flow to synthesize ATP from ADP and Pi in the matrix. ATP synthase creates a hydrophilic pathway across the inner mitochondrial membrane that allows protons to flow down their electrochemical gradient. As these ions move thru the ATP synthase, they are used to drive the energetically unfavorable rxn between ADP and Pi that makes ATP. ATP yield Q. How many ATP do we get per glucose in cellular respiration? A. 30-32 net ATP Q. Where does the figure of 30-32 ATP come from? A. Two net ATP are made in glycolysis, and another two ATP (or energetically equivalent GTP) are made in the citric acid cycle. Beyond those four, the remaining ATP all come from oxidative phosphorylation. https://www.khanacademy.org/science/ap-biology/cellular-energetics/cellular-respiration-ap/a/oxidative-phosphorylation-etc https://www.khanacademy.org/science/ap-biology/cellular-energetics/cellular-respiration-ap/a/oxidative-phosphorylation-etc The mitochondrion has many critical roles in cell metabolism The mitochondrion has many critical roles in cell metabolism The critical role of mitochondria is to produce the ATP that cells need to maintain themselves. Also supply NADPH to the reactions in the cytosol when there is plenty of food and ATP. Excess citrate produced in the mitochondrial matrix by the citric acid cycle is transported to the cytosol, where it is metabolized to produce NADPH. Mitochondria are also critical for buffering the redox potential in the cytosol. Bacteria also use chemiosmotic mechanisms to harness energy Bacteria use different energy sources: Aerobes: Synthesize ATP from sugars they oxidize to CO2 and H2O by glycolysis, the citric acid cycle, and a respiratory chain in their plasma membrane. Strict anaerobes: Derive their energy from glycolysis alone (fermentation) or from an electron-transport chain that uses a molecule other than oxygen (e.g. Nitrogen, sulfur, carbon compound) as the final electron acceptor. Chemiosmotic Mechanisms First Arose in Bacteria Despite this diversity, the plasma membrane of most bacteria contain an ATP synthase. In bacteria that use an electron transport chain to harvest energy, the e transport chain pumps H+ out of the cell and establishes a proton motive force across the plasma membrane that drives ATP syhtase to make ATP. In other bacteria, the ATP synthase works in reverse, using the ATP produced by glycolysis to pump H+ and establish a proton gradient across the plasma membrane. Chemiosmotic Mechanisms First Arose in Bacteria In other bacteria, the ATP synthase works in reverse, using the ATP produced by glycolysis to pump H+ and establish a proton gradient across the plasma membrane. Most bacteria including the strict anaerobes maintain a proton gradient across their plasma membrane. It can be harnessed to drive a flaggellar motor, and it is used to pump Na+ out of the bacterium via a Na+-H+ antiporter. This gradient is also used for the active inward transport of nutrients such as most amino acids and many sugars. Review Questions TRUE (T) or FALSE (F) ( ) Mitochondria are small round organelles that are often associated with actin filaments of the cytoskeleton. ( ) Mitochondria are large enough to be seen with modern light microscopy, and can occupy as much as 20% of cytoplasmic volume. ( ) The outer mitochondrial membrane is freely permeable to ions and small molecules. Review Questions TRUE (T) or FALSE (F) ( F ) Mitochondria are small round organelles that are often associated with actin filaments of the cytoskeleton. ( T ) Mitochondria are large enough to be seen with modern light microscopy, and can occupy as much as 20% of cytoplasmic volume. ( T ) The outer mitochondrial membrane is freely permeable to ions and small molecules. Indicate whether each of the following descriptions better matches the outer mitochondrial membrane (O), the intermembrane space (S), the crista membrane portion of the inner mitochondrial membrane (I), or the matrix (M). ( ) It is where NADH is produced. ( ) It is where the respiratory chain is located. ( ) It has the same electrochemical potential for H+ as the cytoplasm. ( ) It contains porins. Indicate whether each of the following descriptions better matches the outer mitochondrial membrane (O), the intermembrane space (S), the crista membrane portion of the inner mitochondrial membrane (I), or the matrix (M). (M) It is where NADH is produced. ( I ) It is where the respiratory chain is located. ( S ) It has the same electrochemical potential for H+ as the cytoplasm. (O ) It contains porins. A C B D