Mechanical and Electrical Equipment for Building PDF
Document Details
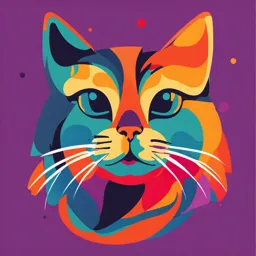
Uploaded by SignificantSymbolism2108
Grondzik Kwok Stein Reynolds
Tags
Related
- Module 1: Building Utilities 2 PDF
- Building Utilities 2 – Electrical, Electronics, and Mechanical Systems Module 1: Thermal Comfort PDF
- Mechanical and Electrical Equipment for Buildings (11th Edition) PDF
- Mechanical and Electrical Systems in Architecture, Engineering, and Construction PDF
- MEP 1.pdf PDF
- Mechanical Ventilation PDF
Summary
This document discusses different aspects of Mechanical and Electrical Equipment for Building design in detail. It covers topics like room acoustics to improve speech intelligibility and music performance, including various aspects of designing a music hall.
Full Transcript
782 CHAPTER 18 SOUND IN ENCLOSED SPACES situations, or if high- and low-end frequencies are of the ideal room must ensure the ear’s undistorted interest, NRC is nearly useless, and detailed analysis reception of these phonemes. This requires ke...
782 CHAPTER 18 SOUND IN ENCLOSED SPACES situations, or if high- and low-end frequencies are of the ideal room must ensure the ear’s undistorted interest, NRC is nearly useless, and detailed analysis reception of these phonemes. This requires keeping of absorption over the entire frequency range must reverberation to a minimum. We can obtain a good be made. Two materials with the same NRC can per- approximation of the subjective feeling of liveness of form quite differently, since NRC is an average, and a room, for purposes of speech, from the relation few materials have a flat absorption characteristic. V Losing sight of such a difference by reliance upon TR (speech) = 0.3 log (18.18) 10 NRC is not a good move. Even more importantly, where sound absorption is not the same as noise reduc- tion. An acoustical ceiling tile, which exhibits high TR (speech) = optimum reverberation time in seconds, sound absorption, is a poor noise control barrier. for speech V = room volume, m3 For instance, a typical classroom might have a vol- RO O M D ESIGN ume of 150 m3 (5300 ft3). Optimum reverberation ACOUSTICS time is TR = 0.3 log 15 = 0.35 s 18.11 REVERBERATION CRITERIA FOR SPEECH ROOMS Reverberation times longer than this would sound live, shorter ones dead and flat. Indeed, an increase The overriding criterion for speech is intelligibility. of 20% in reverberation time would make the room Since speech consists of short, disconnected sounds excessively live and boomy and would negatively 30 to 300 ms in length (see Section 17.4), among affect speech intelligibility. Figure 18.13 gives which are high-frequency, low-energy phonemes, optimum midfrequency reverberation times as a Fig. 18.13 Optimum reverberation times for the frequency range 500 to 1000 Hz as a function of room volume. (The cubic foot volume figures are based upon the approximate conversion factor of 35 ft3/m3). (Reprinted by permission from E. B. Magrab. 1975. Environmental Noise Control. Wiley, New York. p. 206.) REVERBERATION CRITERIA FOR SPEECH ROOMS 783 Fig. 18.14 Maximum recommended reverberation time for speech in auditoriums and lecture halls. (From P. V. Brüel. 1951. Sound Insulation and Room Acoustics. Chapman & Hall, London.) ACOUSTICS function of room size and use. Figure 18.14 gives path is no greater than 56 ft (around 17 m), and maximum reverberation times for speech in large preferably 46 ft (14 m) or less at midfrequency (Fig. rooms. For good speech intelligibility, reverberation 18.15). For more details concerning this and related time should remain essentially flat down to 100 Hz. factors on reflected paths, refer to Section 18.13. The reflected sounds associated with rever- Too low a reverberation time (very high beration can have either a salutary or a deleterious absorption, minimum reflection) is also undesirable effect. The ear cannot distinguish between sounds because: that arrive within a maximum of 50 ms (1 20 s) of 1. It limits the size of the room to that which can each other (some authorities use 40 ms, i.e., 1 25 s). be covered by direct sound only. Sounds arriving within this time reinforce the direct- 2. It is disturbing to the speaker, since absence of path signal and appear to come from the source. reflection prevents him or her from gauging the Sounds arriving after this time are apprehended as proper voice level and tends to cause excessive a fuzzy echo or elongation of the sound, reducing effort (shouting, as when outdoors). intelligibility and directivity. Since the range of 40 to 50 ms corresponds at Thus, proper design of a room for speech is a com- 1128 fps (344 m/s) to 45 to 56 ft (13.7 to 17.2 m), promise between the need for some reflection a speech room should be so arranged that the differ- and the desire to minimize reflection to preserve ence between the first reflection path and the direct intelligibility. Fig. 18.15 Sound paths in a typical medium-sized lecture room. Note that for both extremes of listener position, the maximum path- length difference between direct and first reflection is 11 ft (3.4 m). Thus signal is reinforced, and intelligibility should be excellent if room absorption is provided to limit reverberation time to about ½ second maximum (see Fig. 18.14). Numbers in parentheses are dimensions in meters. 784 CHAPTER 18 SOUND IN ENCLOSED SPACES 18.12 CRITERIA FOR MUSIC researchers, 35% to 75% longer than TR at the cen- PERFORMANCE ter frequencies. 4. Short TR at upper frequencies adds directiv- Adequate design for a music space requires recogni- ity to the music. With large ensembles, directivity tion of the following: gives the sense of depth and instrument location necessary for proper appreciation. This is often 1. Large-volume spaces require direct-path referred to as clarity or definition in music. With a sound reinforcement by reflection. solo instrument, this problem is diminished. 2. Relatively long reverberation time is needed 5. Brilliance of tone is primarily a function of to enhance the music—the exact amount depend- high-frequency content. Since these frequencies ing upon the type of music (Figs. 18.13 and 18.16). are most readily absorbed, a good direct path must Designers should keep in mind that reverberation exist between sound source and listener. Since our time recommendations vary as much as 100% eyes and ears are close together, a good sound path among respected sources. exists when a good vision path exists. At the other 3. It is generally agreed that reverberation end of the spectrum, lack of sufficient bass expresses time should vary inversely with frequency (i.e., ACOUSTICS itself as a loss of “fullness,” which is often caused by TR should be longer at lower frequencies [than the resonant absorption. midfrequency recommendation] and shorter at higher frequencies). The longer TR at low frequen- The actual design of a music performance cies adds fullness to music and “body” to speech. space is a very complex procedure involving Thus, TR at 100 Hz should be, according to most extensive calculations of absorption, reverberation Fig. 18.16 Optimum reverberation times at midfrequencies (500–1000 Hz) for various types of facilities. The wide range shown reflects the effects of room proportions, shape, and volume on the optimum TR, as well as differences of opinion among designers. SOUND PATHS 785 time, and ray diagramming, as well as juggling of music performance. Most modern design solutions materials, dimensions, and wall angles. Simula- also use movable reflector panels and other active tion techniques and acoustic models are also often variables. After construction is completed, exten- employed. sive tests are conducted and field adjustments are Recent research and simulation studies of made. concert and recital halls have demonstrated that the sensation of fullness of music, or what is today referred to as sound envelopment, is enhanced by 18.13 SOUND PATHS lateral reflections that reinforce the direct signal. It has also been found that the subjective judgment Ideally, every listener in a lecture hall, theater, or of reverberance is more strongly affected by early concert hall should hear the speaker or performer decay time (the time required for a 10-dB decrease with the same degree of loudness and clarity. Since in signal strength) than by the conventional 60- this is obviously impossible by direct-path sound, dB decay time. Finally, crispness or clarity of the the essential design task is to devise methods for music (particularly important in recital halls and reinforcing desirable reflections and minimizing ACOUSTICS for chamber music) depends upon reflections arriv- and controlling undesirable ones. Normally only ing within 40 to 70 ms. All of these factors are con- the first reflection is considered in ray diagramming sidered both in the original design and in the often (discussed in the next section) since it is strongest. lengthy “tuning” process of a space intended for Second and subsequent reflections are usually Fig. 18.17 Use of an angled reflector panel (b) creates an image source that stands in approximately the same relation to the audience as does the performer in the classic Greek theater (a). 786 CHAPTER 18 SOUND IN ENCLOSED SPACES attenuated to the point that they need not be con- frequency under consideration. Figure 18.18 is a sidered except for the special situations of flutter, chart for converting from frequency to wavelength echoes, and standing waves, discussed in the fol- in feet and meters. lowing sections. (b) Echoes (a) Specular Reflection As explained in Section 18.11, a clear echo is caused Specular reflection occurs when sound reflects off when reflected sound at sufficient intensity reaches a a hard, polished surface. This characteristic can be listener more than 50 ms after he or she has heard used to good advantage to create an effective image the direct sound. (Some authorities place this fig- source. In ancient Greek and Roman theaters, seats ure as high as 80 ms.) Echoes, even if not distinctly were arranged on a steep conical surface around discernible, are undesirable. They make speech less the performers. The virtue of this arrangement (Fig. intelligible and make music sound “mushy.” The 18.17a) is that the sound energy travels to each relative undesirability depends upon the time delay location with minimal attenuation. The same effect and loudness relative to the direct sound, which, in ACOUSTICS can be accomplished by placing the sound source turn, are dependent upon the size, position, shape, above the seats. This is not practical physically, and absorption of the reflecting surface. but it can be accomplished effectively by the use of Typical echo-producing surfaces in an audi- a reflecting panel (Fig. 18.17b). The panel dimen- torium are the back wall and the ceiling above the sion must be at least one wavelength at the lowest proscenium. Figure 18.19 shows these problems and suggests remedies. Note that the energy that produced the echoes can be redirected to places where it becomes useful reinforcement. If echo con- trol by absorption alone were used on the ceiling and back wall, that energy would be wasted. The rear wall, since its area cannot be reduced too far, may have to be made more sound-absorptive to reduce the loudness of the reflected sound. (c) Flutter A flutter, perceived as a buzzing or clicking sound, comprises repeated echoes traversing back and forth between two nonabsorbing parallel (flat or concave) surfaces. Flutters often occur between shallow domes and hard, flat floors. The remedy for a flutter is either to change the shape of the reflectors or their parallel relationship or to add absorption. The solution chosen will depend upon reverbera- tion requirements, cost, and aesthetics. (d) Diffusion This is the converse of focusing and occurs primar- ily when sound is reflected from convex surfaces. A degree of diffusion is also provided by flat horizon- tal and inclined reflectors (Fig. 18.20). In a diffuse Fig. 18.18 Nomograph for determining wavelength in feet or sound field, the sound level remains relatively con- meters, given frequency in hertz, or vice versa. Speed of sound is taken as 1128 ft/s (344 m/s). To use it, hold a straightedge hori- stant throughout the space, an extremely desirable zontally across the nomograph and read the figures directly. property for musical performances. SOUND PATHS 787 Fig. 18.19 Auditorium section showing the causes and remedies for two typical echoes. (e) Focusing when the parallel walls are spaced apart at some integral multiple of a half-wavelength. Concave domes, vaults, or walls will focus reflected ACOUSTICS When parallel walls are exactly one-half wave- sound into certain areas of rooms. This has several length apart, the tone will sound very loud near the disadvantages. For example, it will deprive some walls and very quiet halfway between them. This is listeners of useful sound reflections and cause hot because at the center, the reflected waves traveling spots at other audience positions (Fig. 18.21a). in one direction are exactly one-half wavelength away from those traveling in the other direction, (f) Creep and are thus equal but opposite in pressure, which results in total cancellation. In other rooms, stand- This describes the reflection of sound along a curved ing waves are noted as points of quiet and loudness surface from a source near the surface. Although in the room. Standing waves are important only the sound can be heard at points along the surface, in rooms that are small with respect to the wave- it is inaudible away from the surface. Creep is illus- lengths generated (smallest room dimension