MCB 32 Intro to Human Physiology Final Exam Study Guide PDF
Document Details
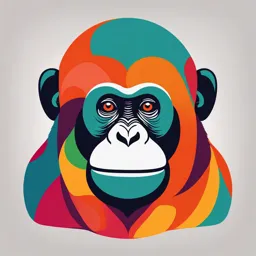
Uploaded by InfluentialChimera3760
UC Berkeley
Tags
Summary
This document provides a study guide for an exam in human physiology. It covers initial topics such as homeostasis. The document also looks at the different chemical structures and processes occurring in biological organisms.
Full Transcript
MCB 32 Intro to Human Physiology Final Exam Study Guide Module 1: Homeostasis Human physiology is the study of how the human body functions. It involves the integration of multiple organ systems working together. Organ systems are all working together towards one common goal or function There...
MCB 32 Intro to Human Physiology Final Exam Study Guide Module 1: Homeostasis Human physiology is the study of how the human body functions. It involves the integration of multiple organ systems working together. Organ systems are all working together towards one common goal or function There are parts of the human body that are in the external environment, like the inside of the digestive tract. Parts of the body that are directly connected to the outside are called external environment Material from the external environment is brought into the internal environment by epithelial cells and into blood vessels Blood vessels carry the matter all around the body This is the same process that removes the waste from our bodies The human body is made up of water that is inside cells (intracellular) and outside of cells (extracellular). Intracellular fluid: makes up about ⅔ of all the water in our body Extracellular fluid: fluid outside of cells (in internal environment) ○ In blood called the plasma ○ Outside of blood called the interstitial fluid In the space outside of cells that's called the interstitial space The internal environment is kept within a normal range of parameters, which is known as homeostasis. Negative feedback control helps to maintain homeostasis. Negative feedback looks like regulating our temperature are more common than positive feedback loops Stimulus is measured by a sensor Information from the sensor is compared to the set point in the integrating center Effectors are cells and tissues that bring about the final response An example of homeostasis is how we maintain our internal body temperature within a normal range. Negative feedback involves sensors that can sense the change in stimulus, an integrating center to compare the current conditions to the set point, and effectors that cause the response. Sensors for body temperature in our body are thermoreceptors ○ Located in the skin and the hypothalamus Sensors send information to the integrating center which is in the hypothalamus as well Signals to the effectors such as muscles to shiver to produce heat and warm the body up Another response would be vasoconstriction Other examples include ○ Baroreceptor reflex ○ Regulation of osmolarity in by the kidney ○ insulin/glucagon regulation of blood glucose levels Positive feedback occasionally occurs in the body and does not help maintain homeostasis. During positive feedback the response amplifies the stimulus. Occurs in during ovulation when there is a release of the egg Additionally in blood clotting that causes a wound to heal Module 2: Chemistry and Biomolecules Atoms in a molecule are held together by covalent bonds. Electrons are shared between two atoms Two nonmetals Covalent bonds can be nonpolar, which means that the electrons are shared evenly between atoms. Carbon-carbon and carbon-hydrogen bonds are nonpolar. Covalent bonds can also be polar, which means that electrons are not shared evenly. This occurs when there is an unequal sharing of electrons Oxygen and nitrogen pull electrons towards them away from hydrogen and carbon. Molecules that have a lot of nonpolar bonds are hydrophobic/lipophilic. They interact better with fats than with water. Triglycerides and steroids Molecules that have a lot of polar bonds are hydrophilic/lipophobic. They can form hydrogen bonds with water and interact better with water than with fats Amino acids, proteins, sugars, nucleic acids. Ions are charged atoms or molecules Na+, K+, Ca+2, Cl- Ionic bonds occur with the complete transfer of one or more electrons pH is a measure of H+ ions in a solution Most of the body is near pH 7, which is neutral Acids have a lower pH, there is decreased H+ Bases have a higher pH, H+ is in access Biomolecules are synthesized by the body and consist of four groups: carbohydrates/sugar, lipids, proteins, nucleic acids. Each type of biomolecule serves an important function inside of cells Carbohydrates (cell recognition, broken down for energy, added to proteins and lipids to change their functions) ○ Found in rings with carbon, hydrogen, and oxygen ○ Monosaccharides ○ Disaccharides (two monosaccharides covalently bonded together) ○ Polysaccharides (many monosaccharides all together) Lipids (hydrophobic) ○ Two structures Long carbon hydrogen chains: fatty acids 4 carbon rings: steroids Synthesized from cholesterol ○ Triglycerides Made of a glycerol and three fatty acid chains ○ Phospholipids Made up of a glyceride, one fatty acid, and phosphate containing group Proteins (chemical messengers, receptors, move molecule across the membrane, provide structural support for cells, act as enzymes, catalyze reactions ) ○ Made up of amino acids ○ There are 20 different amino acids that have a differing R group ○ 50 amino acids together it's called a protein Nucleic acids ○ Monomer is nucleotide ○ Contains phosphate group, 5 carbon sugar, and a nitrogenous base ○ ATP is a nucleotide that is used to store cellular energy Memorize this one STAT Module 3: Enzymes and Energy Anabolic vs. Catabolic reactions Anabolic reactions involve the synthesis of larger molecules. ○ Energy is needed in order to do an anabolic reaction. ○ Energy comes from ATP, which is made by catabolic reactions. Catabolic reactions involve breaking down larger molecules into smaller ones ○ Energy is released by catabolic reactions, but there is an activation energy needed in order to initiate a catabolic reaction ○ Enzymes help by lowering the activation energy that is needed for a certain reaction ○ Activation energy is good because it makes it so the body can regulate what reaction happen where/when inside of the body Enzymes are proteins that bind specific substrates and speed up chemical reactions. Substrates bind enzymes in the active site Cells can synthesize more enzymes to speed up a reaction, or destroy an enzyme to stop the reaction from occurring With a lower activation energy cells can speed up reactions Enzymes are not used up in a reaction and can be used over and over again Does so by increasing affinity for the enzyme in the substrate Enzymes can also be regulated by small activators or inhibitors in a process known as allosteric regulation Activators and inhibitors are small molecules that reside outside of the active site They bind it causes the enzyme to change its affinity for the substrate Can bind using hydrogen bonds This bond is weak and easily reversible Enzymes can be regulated by covalent modification, like having a phosphate group added to them Covalent modification occurs when an enzyme is covalently bonded to something else Often a phosphate group Due to the covalent bond being stronger than the hydrogen bond of allosteric regulation it is longer lasting Module 4: Tissues and Cells There is a hierarchical structure in the body from molecules, to cells, tissues, organs and organ systems Cells make up tissues Tissues make up organs Organs make up organ systems That's how our bodies operate There are four types of tissues: epithelial, connective, muscle, nervous Epithelial ○ Continuous sheet-like layer of cells ○ Lines the inside of body tubes ○ Are held together by tight junctions ○ Are glands Exocrine glands: secrete chemicals into the external environment Endocrine glands: secrete hormones into the bloodstream ○ Epithelial tissue separates the internal environment from the external environment, surrounds tubes (like blood vessels), and forms exocrine/endocrine glands. Connective ○ Provides structural support ○ Blood is an example of connective tissue ○ Bones are examples of connective tissues in the body ○ Loose connective tissue ○ Connective tissue is made up of scattered cells in an extensive extracellular matrix, like bones, blood, tendons, fat, under the skin, etc. Muscle ○ Contracts and has the ability to produce force ○ Three types Smooth muscle tissue Cardiac muscle tissue Skeletal muscle tissue ○ Muscle tissue contracts and produces force. Nervous ○ Neurons and their support cells called glia ○ Nervous tissue consists of neurons and glia cells that are important for regulating the rest of the body. Know the main function and structure of the following cell structures: Plasma membrane: surrounds the cell and is made up of the phospholipid bilayer ○ Membrane is dynamic and not solid Extracellular matrix: everything that's going on outside of the cell Nucleus: surrounded by two membranes and contains all of the genetic material (DNA associated proteins) ○ Gene transcriptions occurs here in the nucleus Cytoplasm: inside of the cell aside from the nucleus, made up of a gel-like substance called cytosol made up of organelles Endoplasmic reticulum: network of membranes surrounding the nucleus ○ Smooth ER (endoplasmic reticulum): synthesizes lipids ○ Rough ER: has ribosomes attached and is responsible for protein synthesis Golgi apparatus: associated with the ER, receives proteins and finished processing them ○ Packages everything up all perfect to be shipped off Vesicle: carry proteins to the plasma membrane where its membrane fuses with the plasma membrane and causes releases the contents outside of the cell ○ This is called exocytosis Lysosome: digest and remove debris and old organelles from the cell Mitochondria: made of two sets of membranes, has its own DNA and is a critical part of ATP and the making of cellular energy Cytoskeleton: specialized proteins that provide cell structure and cellular support ○ Very dynamic and allows the cells to to grow and change their shape based off of the need of the cell as a whole Module 5: Membrane Transport The main function of the plasma membrane is to act as a selective barrier between intracellular and extracellular spaces Keeps everything in one place Selectively permeable and regulates the movement ions in and out of the cell Involved in cell to cell communications Membrane attaches cells to extracellular matrix The plasma membrane is a lipid bilayer composed of phospholipids, proteins and sugar. Phospholipids can interact with both lipids and water Polar heads and nonpolar tails Many of the functions of the plasma membrane are carried out by the proteins that are present there ○ Receptors ○ Transporters ○ Ion-channels Only small non-polar molecules can simply diffuse across the membrane. The rate of simple diffusion depends on the concentration gradient, surface area, membrane permeability and distance Simple diffusion molecules include ○ Oxygen ○ Carbon ○ Steroid hormones ○ Fatty acids Polar molecules (glucose) or charged molecules need help from transmembrane proteins to cross this is called facilitated diffusion When molecules diffuse from high concentration to low concentration, it does not require energy and is called passive transport Moving with its concentration gradient it is passive transport and does not require help Once sides have equalized there is no net movement but molecules will still move back and forth Passive transport consists of simple diffusion for non-polar molecules and facilitated diffusion for polar molecules. Osmosis is the passive transport of water through aquaporins Simple diffusion for non polar molecules Facilitated diffusion is for polar molecules (due to the size of these molecules they use transporters or carriers) Factors that affect the rate of diffusion Concentration gradient Surface area of the membrane Membrane permeability Size of molecule diffusing Diffusion distance When molecules diffuse from low to high concentration, it requires energy and is called active transport Directly uses ATP to transport substances across their gradient Na+/K+ pump in 3 na out and 2 k into the cell Active transport is only used for polar molecules and requires energy from ATP (primary active transport) or energy from diffusion of another molecule (secondary active transport) Net movement of low to high concentration (against the concentration gradient) Primary transport: energy comes from ATP Secondary transport: energy comes from another molecule moving down its concentration gradient (uses pumps) ○ Cotransport: transport of two molecules in the same direction ○ Countertransport: transport of two molecules in opposite directions Module 6: Intracellular Signaling Cells communicate to each other via chemical messengers, or ligands, that bind receptors in the target cell Critical to maintain homeostasis throughout the body Chemical messengers ○ Some cells have gap junctions enables cells to communicate by passing small chemicals ○ Most cells communicate with chemical messengers ○ Three types Paracrine signal (short distance) Neurotransmitters (short distance between cells) The nervous system uses neurotransmitters that travel a short distance between cells. Neurotransmitters are always polar and lipophobic. Hormones (released into the blood from endocrine cells and travel very long distances) The endocrine system uses hormones that can travel long distances in the blood Peptide hormones are lipophobic Steroid hormones are lipophilic. Lipophilic Messengers Lipophilic messengers (steroid hormones) are nonpolar so they can diffuse through the membrane of the target cell Lipophilic messengers use intracellular receptors inside the target cells Cannot be made and stored ahead of time because they will simply diffuse right out of the cell Bind intracellular receptors Lipophobic messengers Cannot go through membranes because they are polar, so they are released by exocytosis and bind receptors that are in the membrane of the target cell. Receptors are on the membrane of the cell Different types ○ Amino acids ○ Amines ○ Peptides/protein messengers There are three types of membrane receptors: ligand-gated channels (ionotropic receptors), enzyme-linked receptors, and G protein coupled receptors (metabotropic receptors). Ligand-gated ion channels (neurotransmitters) ○ open/close when a specific ligand binds them Enzyme-linked receptor (for hormones) ○ Receptor is an enzyme ○ Catalyzes chemical reactions in the cell from its location in the membrane ○ Kinases: catalyze the addition of a phosphate group ○ Only used for lipophilic hormones G-protein coupled receptor (for both neurotransmitters and hormones) ○ Ligand binds a GPCR ○ Activates the G-proteins, triggers signaling to open/close ion channels ○ Activation of a second messenger ○ cAMP pathway is an example of this GPCR → g-protein is activated and searates from its receptor → g-protein travels along the inside of the cell membrane → bind the ace → ace catalyzes the reaction ATP → cAMP → activates the enzyme PKA that phosphorylates other proteins → creates a cellular response Module 7: Endocrine System The endocrine system releases hormones that travel through the blood to target cells Everything that secretes hormones into the bloodstream There are three types of hormones: peptide, steroid and amines. We will focus on peptide (lipophobic) hormones and steroid (lipophilic) hormones. Peptide: lipophobic, insulin, ACTH, oxytocin Steroid: lipophilic, cortisol, aldosterone, testosterone, estrogen, progesterone Primary Endocrine Organs The hypothalamus and pituitary are primary endocrine organs, because one of their main functions is to release hormones Secondary Endocrine Organs: Secrete hormones but its not their main function ○ Heart, liver, kidney The pituitary has two distinct areas posterior pituitary that releases lipophobic neurohormones ADH (water balance) and oxytocin (milk release, uterus contraction, social bonding) anterior pituitary which releases a lot of different lipophobic hormones, including ACTH The hypothalamus releases hormones that regulate the release of other hormones from the anterior pituitary This is called a trophic hormone This system consists of two capillaries in a row, which is known as a portal system. HPA axis CRH is released from the hypothalamus and signals release of ACTH from the anterior pituitary, which causes cortisol release from the adrenal cortex Cortisol is released when the body is under stress and it stimulates breakdown of proteins and fat for energy, and it increases glucose in the blood Long term stress stimulates CRH secretion ○ Surgery, burns, illness, exercise Stereotype threat Occurs when someone is concerned that they will confirm a stereotype about their group Cortisol levels increase due to a stress response and there is increased cognitive load, causing the individual to underperform Learning about stereotype stress will help decrease stereotype stress Hypersecretion Is often causes by tumors in the endocrine cells Module 8: Reproductive System One of the main functions of the reproductive system is to produce gametes (sperm or eggs) that carry one copy of the genome. During fertilization, the genetic material from a sperm and egg combine to make a diploid zygote. Sperm and egg each only have one copy of the genes they come together to make a diploid zygote during fertilization Sperm and egg contain the genetic material of most cells Each contain 26 chromosomes Everyone produces all of the three sex hormones but in different amounts which changes the way that we are as individuals Sperm are produced in the seminiferous tubules in the testes. As sperm travel from the testes to the penis, glands secrete fluids and chemicals to produce semen Seminiferous tubules → epididymis → vas deferens → urethra → penis Secrete things to add to sperm that make semen ○ Seminal vesicles, prostate gland, and bulbourethral gland All considered exocrine glands Sperm is very basic to neutralize the acidity in the vagina Sperm production is regulated by testosterone, which is released from cells in the seminiferous tubules. Testosterone is regulated by LH and FSH, hormones secreted by the anterior pituitary. GnRH from the hypothalamus regulates LH/FSH GnRH is released by the hypothalamus in the brain Regulates the release of LH/FSH which is released from the anterior pituitary LH/FSH regulates the amount of testosterone that is released from cells in the seminiferous tubules Testosterone is what regulates sperm production Each month one egg is released from a follicle in the ovary. The egg travels through the fallopian tube to the uterus. Fertilization occurs in the fallopian tube and the resulting embryo grows in the lining of the uterus follicle in the ovary → fallopian tube (fertilization would happen here) → uterus The reproductive system for egg production undergoes structural, functional and hormonal changes each month, which is called the menstrual cycle. The menstrual cycle affects the ovaries (i.e. ovarian cycle) and the uterus (i.e. uterine cycle) Menstrual cycle consists of the ovarian cycle and the uterine cycle Ovarian Cycle ○ Follicular phase: several follicles begin to grow, but only one matures Secretes estrogen ○ Ovulation: egg is released ○ Luteal phase: ruptured follicle transformed into endocrine structure called the corpus luteum (secretes progesterone and estrogen) Uterine Cycle ○ Menstrual cycle: endometrium is shed if there is no pregnancy ○ Proliferative phase: endometrium affs new layers, new blood vessels grow ○ Secretory phase: release mucus, fats, glycogen (nutrients for the embryo) The menstrual cycle is controlled by the hormones FSH and LH from the anterior pituitary, as well as estrogen and progesterone released by the ovaries (follicles and corpus luteum). There are both negative and positive feedback cycles regulating these hormones. Birth control pills are a combination of estrogen and progesterone, which cause negative feedback on the anterior pituitary. 1. Early follicular phase (day 1-11) a. FSH levels increase b. Growing follicles secrete estrogen (one follicle becomes dominant) c. One the dominant follicle is going it doesn't need FSH to continue to secrete estrogen d. Uterine lining continues to grow e. Does negative feedback on the anterior pituitary which causes FSH levels to decrease 2. Late follicular phase (day 11-15) a. Estrogen reaches a certain concentration and goes from being inhibitory to stimulating the anterior pituitary and hypothalamus b. POSITIVE FEEDBACK c. Estrogen goes up → more LH and FSH → more estrogen → more LH (creates LH surge) d. Triggers ovulation → egg is released into the fallopian tube 3. Early Luteal Phase (day 16-25) a. Corpus luteum matures and secretes progesterone and estrogen b. Progesterone and estrogen inhibit the hypothalamus and anterior pituitary (decreased LH and FSH) 4. Late Luteal Phase and Menstruation (day 26-1) a. If there is no pregnancy the corpus luteum degenerates after a week i. As it degrades it stops secreting ii. Without the hormones the tissue is shed iii. There is no negative feedback on the anterior pituitary so FSH levels will increase again and trigger the start of another menstrual cycle b. If there is pregnancy the corpus luteum will keep going until placenta takes over Module 9: Neurons and Membrane Potential Know the key structures in a neuron: dendrites, cell body, axon, axon terminal, initial segment, synapse. Dendrites Cell body Axon hillock Axon Axon terminal: most likely to have Vg Ca2+ channels Initial segment Synapse Neurons have electrical signals that travel from dendrite to cell body, through the axon and then cause release of neurotransmitters. Basic unit of the nervous system Do most of the signaling Glia cells are support cells. We will hear more about oligodendrocytes and Schwann cells when we learn about myelin in the next module. Astrocytes are important for a number of functions, like the blood brain barrier. Provide support for neurons Schwann cells: wrap around the neuron axon, making up the myelin sheath and speeds up action potential conduction Astrocytes: form the blood brain barrier Recent studies show that the glia cells may be more active and participate in more than was initially thought The charge difference across the plasma membrane is known as the membrane potential (Vm). At rest, neurons are usually -70mV inside. Plasma membrane is selectively permeable to different ions Ions have two forces acting on them: the concentration/chemical force (i.e. the concentration gradient) and the electrical force caused by attraction/repulsion based on charge. The direction and strength of these two forces determines which direction the net movement of an ion will be across the membrane. Ions can go against their gradient There is ALWAYS more K+ inside of the cell and more Na+ outside of the cell When the chemical and electrical forces are equal and opposite, the ion is at equilibrium. The charge inside the cell when the ion is at equilibrium is known as its equilibrium potential. EK = -90mV, ENa = +60mV. Concentration gradient is where the ion would want to go based off of where there is more of it in the cell membrane When it leaves the cell gets more negative as the positive ions move outside This causes the electrical gradient to pull the K+ back into the cell The resting membrane potential is around -70mV at rest, because K+ is most permeable and drives the membrane potential close to its equilibrium potential. There are also negatively charged proteins inside the cell and the Na/K pump contributes to the negative membrane potential. Every ion has an equilibrium potential Neither Na+ or K+ are at equilibrium potential in an average neuron but K+ is sort of close K+ continuously leaks out of a cell Na/K pump 3 Na+ go out of the cell and 2 K+ go into the cell When the Vm gets closer to zero, the cell is depolarizing. When the Vm gets even more negative, this is known as hyperpolarization. Depolarization: when the cell is more positive inside (Vm is more positive) Hyperpolarization: when the cell is more negative (Vm is more negative) Module 10: Action Potentials Voltage-gated ion channels open when the membrane depolarizes above a certain threshold (close to -55 mV) This occurs in the initial segment of the axon, thats why its so important Some of the deadliest toxins target voltage gated channels that are essential for neuron function Voltage-gated Na+ and K+ channels are found only in the axon and are responsible for causing the action potential Action potentials are a large depolarization that happens in the axon. They are all or nothing (they are always the same amplitude). They can travel a long distance without decreasing in strength. All or nothing Can travel far without decreasing due to the myelin sheath and the schwann cells They only occur when the threshold for an action potential has been reached What happens during an action potential Voltage-gated Na+ channels open and Na+ enters the axon, causing the large depolarization during an action potential Then voltage-gated K+ channels open and K+ leaves the axon, causing the repolarization at the end of the action potential. Voltage-gated Na+ channels inactivate after being open for about 1 millisecond. This causes the refractory period when an axon cannot fire another action potential. ○ Absolute refractory period: NO AP, first 2ms ○ Relative refractory period: action potential can happen but has to depolarize father than -55 mV, next 5-15 ms Most axons are myelinated by glia cells. The myelin helps the action potential conduct faster down the axon Made up of schwann cells Module 11: Synaptic Transmission The presynaptic neuron releases neurotransmitter onto the postsynaptic cell, which expresses the receptors. Electrical synapse: some neurons are directly connected by gap junctions, AP can spread directly from cell to cell using these Chemical synapse: use neurotransmitters to communicate between cells, most common Synaptic transmission: The action potential causes voltage-gated Ca+2 channels to open in the axon terminal, which triggers the exocytosis of synaptic vesicles filled with neurotransmitters. Neurotransmitters diffuse across the synaptic cleft and bind receptors, causing a response in the postsynaptic cell. Neurotransmitters can be cleared out of the synaptic cleft using reuptake transporters in the presynaptic neuron or nearby glial cells. Some postsynaptic cells have enzymes in the membrane that breakdown the neurotransmitters. The nervous system uses two different types of membrane receptors Ionotropic receptors (ligand-gated ion channels): cause a fast graded potential Metabotropic receptors (GPCRs) that cause a slower, longer-lasting response that could be a graded potential, activating enzymes, or changing gene expression Graded potentials can be depolarizing (excitatory) or hyperpolarizing (inhibitory), depending on which channels are opened in the postsynaptic cell Variable amplitudes not all or nothing really just the same throughout Graded potentials can summate Integration of all the graded potentials happens in the cell body If the summated graded potentials cause the initial segment of the axon to depolarize to threshold, an AP is triggered ○ It can still generate an action potential even if its sum of graded potentials The main neurotransmitters Glutamate (excitatory): most common neurotransmitter in the brain GABA (inhibitory): most common inhibitory neurotransmitter in the CNS Acetylcholine: used in the autonomic nervous system Norepinephrine It's important that the brain has a good balance of excitatory (glutamate) and inhibitory (GABA) ○ Too much excitation: seizures and neuron death ○ Too much inhibition: coma and perhaps DEATH Agonists vs. Antagonists Agonists: drugs that mimic a neurotransmitter and cause the same effects Antagonists: inhibit the receptor, the neurotransmitter cannot bind Module 12: Central Nervous System The central nervous system (CNS) consists of the brain and spinal cord. Neurons that are completely in the CNS are called interneurons. Consists of the brain and the spinal cord Billions of neurons and trillions of synapses The peripheral nervous system (PNS) consists of afferent sensory neurons bringing information to the CNS, and efferent neurons sending information from the CNS to the rest of the body. PNS is made up of neurons going into (afferent) or out of (efferent) the CNS Afferent: send signals towards the CNS, sense the external or internal environment Efferent: transmit information from the CNS to the PNS, they tell muscles what to do The CNS is protected by bones, meninges, cerebrospinal fluid and the blood-brain barrier Protected why a skull Spinal cord protected by vertebrae Meninges are tissue membranes Space is full of cerebrospinal fluid The blood brain barrier physically separates the blood and cerebrospinal fluid ○ Protects the brain from harm in the blood The axons of sensory neurons enter the spinal cord through the dorsal side. Cell bodies are in the dorsal root ganglion. White matter: cluster of axons Grey matter: cell bodies and dendrites SAME DAVE ○ Sensory afferent, motor efferent dorsal afferent, ventral efferent Efferent neuron axons leave the spinal cord through the ventral side The cerebral cortex is the outer region of the brain. It consists of four lobes ○ Frontal ○ Parietal ○ Occipital ○ Temporal The brain stem is important for regulating basic physiological mechanisms like blood pressure and breathing rate Midbrain: controls eye movement, orients head to sound/light Pons: controls sleep, breathing, swallowing Medulla Oblongata: controls blood pressure, breathing Thalamus: relay station for sensory information Hypothalamus: hormone production, temp regulation Damage to the brain can cause very specific symptoms, which helps us understand the main functions for each part of the brain. Very clear connections between symptoms and which part of the brain is damages there should be a way to lock this down a little more (COME BACK) Module 13: Somatosensory System Sensory neurons are specialized to detect specific physical stimuli. Reception of stimulus → sensory transduction → to CNS → sensory coding Sensory transduction occurs in sensory neurons when the physical stimulus causes a change in membrane potential in the cell (graded potential or action potential). The body has to uncode the stimulus Mechanoreceptors sense touch using mechanically-gated Na+ channels that open when the cell membrane is bent. Light touch and pressure Thermoreceptors sense temperature using Na+ channels that open when the temperature changes Some thermoreceptors are active when it is warm and others are active when it is cold Sense temperature A hot day or perhaps chilli peppers Nociceptors sense painful stimuli that can cause damage to tissues Sense painful stimuli Stimuli that could severely damage tissues Extreme pressure or chemicals that are released by damaged tissue during an injury Proprioceptors Are located in the skeletal muscles, tendons, and joints and send information about the body's position to the brain ○ Critical for voluntary movement ○ Help maintain balance (cerebellum) Information from the somatosensory receptors is sent to the thalamus and then the primary somatosensory cortex (S1) in the parietal lobe The primary somatosensory cortex is organized by body position. Body parts that have finer discrimination (fingers, face) take up more area in S1 Synapse onto the 2nd order neurons in the thalamus onto the 3rd order neurons that go into the primary somatosensory cortex Module 14: Autonomic Nervous System The autonomic nervous system (ANS) is part of the efferent peripheral nervous system It is comprised of the sympathetic and parasympathetic nervous systems There are two branches of the ANS ○ parasympathetic controls the body at rest ○ sympathetic gets the body ready for action We cannot control it which is why its called the autonomic nervous system Helps us maintain homeostasis without our conscious input Neurons from CNS to ANS and they’re synapses It takes two neurons to get from the CNS to the ANS target organ The preganglionic neuron has its cell body in the CNS and the axon synapses on the postganglionic neuron in the periphery The postganglionic neuron synapses onto the target cells In both branches, the synapse between pre- and postganglionic neurons uses acetylcholine (ACh) and nicotinic acetylcholine receptors (nAChR) Both branches use the Ach on nicotinic AChR receptors At the SECOND synapse between the postganglionic neuron and target cells The sympathetic branch ○ Uses norepinephrine to signal to adrenergic beta receptors ○ Shorter presynaptic neuron ○ Longer postsynaptic neuron The parasympathetic branch ○ Uses acetylcholine and muscarinic acetylcholine receptors (mAChR) ○ Longer presynaptic neuron ○ Shorter postsynaptic neuron Epinephrine is a neurohormone released by chromaffin cells in the adrenal medulla into the blood, but it binds the same receptors as norepinephrine and causes the same sort of effects Epinephrine is adrenaline Adrenal medulla is associated with the sympathetic nervous system ○ secretes epinephrine and a little norepinephrine ○ Sits on top of the kidney Adrenal cortex: exocrine gland secretes hormones (cortisol and aldosterone) Many medicines affect the neurotransmitter receptors in the ANS, as either agonists or antagonists. Agonist: binds and activates the receptor, just like a neurotransmitter or hormone would Antagonist: binds and inhibits prevents the ligand from activating the receptor Module 15: Skeletal Muscle Skeletal Muscle: attached to bones and controls body There are three types of muscle tissue: skeletal muscle, cardiac muscle and smooth muscle. movement, striated Cardiac Muscle: muscle in the heart, striated Smooth Muscle: muscle in organs and tubes, not striated (hence the smooth part) One motor neuron will make a synapse with several muscle fibers. The synapse is called the neuromuscular junction (NMJ). Motor is the other part of the efferent system and contains the skeletal muscle Under conscious control via the somatic motor system Some things are controlled unconsciously however The entire thing is called a motor unit Acetylcholine is released by the motor neuron and binds nicotinic acetylcholine receptors on the muscle. This is always an excitatory synapse and the graded potential almost always leads to an action potential in the muscle Uses acetylcholine and AChRs The signal is terminated by an enzyme called acetylcholinesterase (AChE) which is located in the postsynaptic membrane Muscles are filled with actin and myosin arranged in structures called the sarcomere. Muscle fibers have some specialized cell structures ○ Sarcoplasmic reticulum: smooth ER, filled with Ca2+ ○ Myofibril: made of actin and myosin, which causes contraction ○ T-tubules: ling tubes in the membrane Myosin is a motor protein that binds actin and causes the actin filaments to move towards each other during muscle contraction Tropomyosin wraps around actin and is bound by troponin This is known as the crossbridge cycle and requires ATP Calcium is stored in the sarcoplasmic reticulum Calcium binds troponin, which causes tropomyosin to move off of the myosin binding sites on actin. This is a necessary step for muscle contraction. The force in a single muscle fiber can summate and can lead to a maximum force known as tetanus The muscles maximum possible force ever Z-lines are close together during muscle contraction Multiple muscle fibers in a muscle will be recruited to contract at the same time, causing a stronger force There are three main types of muscle fibers that differ in their usage of oxygen, their speed and strength and their resistance to fatigue Slow oxidative: slow to generate force, produces weak force, highly resistant to fatigue Fast oxidative: medium speed, medium force, medium resistance to fatigue Fast glycolytic: fast to generate force, produces strong force, low resistance to fatigue ○ Rely on anaerobic anaerobic respiration (glycolysis) Module 16: Voluntary Movement and Reflexes Proprioceptors are part of the somatosensory system and send sensory information about the skeletal muscles to the brain. There are two types ○ Muscle spindles: sense muscle length ○ Golgi tendon organs: sense muscle tension Located in muscles and tendons A reflex is an involuntary and rapid response to a stimulus. Spinal reflexes only involve the spinal cord (not the brain). Follow the same pathway as mechanoreceptors ○ Cross over in the medulla oblongata ○ Nervous system is always receiving signals from the proprioceptors Reflex The patellar stretch reflex uses just a few neurons to cause the leg to straighten when the tendon below the knee is stretched When the quads lengthen, the muscle spindles senses this and sends sensory signals to the spinal cord An inhibitory interneuron in the spinal cord causes the hamstrings to relax while the quads contract This is called an antagonistic muscle pair (one contracts while the other relaxes) The motor cortex has neurons that extend down into the spinal cord to control motor neurons and voluntary movement Is used for voluntary motor control and spinal reflexes Descending pathway from the brain on out The right motor cortex controls the left side of the body and vice versa Left motor cortex controls the right side of the brain Module 17: Circulatory The circulatory system transports molecules and cells in blood through the body The heart provides the force for blood movement There are two loops (connected by the heart) in the circulatory system Pulmonary circulatory system: takes blood to the lungs Systemic circulatory system: that carries oxygenated blood to all the tissues in the body Blood exits the heart via arteries The aorta and pulmonary arteries directly leave the heart and branch into smaller arteries The aorta leaves on the left side of the heart And the pulmonary artery leaves on the right side of the heart Heart has four chambers Left ventricle Right ventricle Left atrium Right atrium Blood flows atria through the ventricle on each of the sides Capillaries: the site of exchange between blood and interstitial fluid Blood vessels Heart → arteries → arterioles → capillaries → venules → veins → heart ○ Arteries: pressure reservoirs ○ Arterioles: sites of variable resistance (vasodilate and vasoconstrict) ○ Capillaries and venules: sites of exchange ○ Veins: volume reservoirs Arteries give rise to arterioles, which have variable resistance that can be controlled by the sympathetic nervous system, hormones and paracrine signals. After the arterioles, blood goes into capillaries, which is where molecules diffuse into and out of the blood. From the capillaries, the blood enters the venules and then the veins. The veins can stretch to hold more volume of blood. There are one way valves in veins to help get blood back to the heart. The two veins that enter the heart are the pulmonary vein and vena cava. Module 18: Heart and Electric Conduction The heart is composed of cardiac muscle cells (cardiomyocytes) that contract and generate the force to push blood through the circulatory system Heart sits in the pericardium for protection Have intercalated disks between neighboring cells for conduction ○ Dososomes: strong cell-adhesion protein structures ○ Gap junctions: allow direct and rapid spread of ions Action potential generated is 100X stronger than a neural AP because of the calcium The heart has four chambers: two atria and two ventricles Blood enters the atria first and then goes to the ventricles and then the arteries. There are one-way valves between the atria and ventricles and between the ventricles and arteries that prevent the backflow of blood ○ AV valves: between the atrium and the ventricles ○ SL valves: between the ventricles and arteries The valves open when there is a pressure difference ○ AV open when Patria>Pvent ○ SL open when Pvent>Parteries Arteries leaves from the top of the heart FYI Pacemaker cells generate their own action potentials without input from the nervous system. The primary pacemaker is the SA node in the right atrium It initiates the heartbeat, that is its main function The action potentials in cardiomyocytes and pacemaker cells are different than in neurons and involve different voltage-gated channels Use different channels at different times Pacemaker cells have the funny channels that the contractile cells do not have when they create action potentials (memorize so hard) Cells in the heart have gap junctions that allow the depolarization from action potentials to spread from cell to cell, starting at the SA node and going through the atria and then the ventricles. When the SA node is depolarized the positive ions spread out That initiates action potentials in the contractiles cells by bringing them to threshold SA node depolarizes → atria depolarize → AV node depolarizes → Spreads through conducting fibers into the ventricles → spread through ventricles → ventricles depolarize and the whole thing starts all over again The large wave of depolarization that spreads through the heart during one cardiac cycle can be recorded with electrodes on the skin surface. This is called the electrocardiogram and it can be used in medicine to diagnose heart conditions The EKG is not intracellular but rather the summed activity from the cells P wave: atria depolarize QRS complex: ventricles depolarize T wave: ventricles repolarize What we can see Bradycardia: heart is beating too slow Tachycardia: heart is beating too fast Fibrillation: heart depolarization is no longer synchronized, can be deadly Module 19: Cardiac Cycle Each heart beat, the heart goes through a set of coordinated events to pump blood out of the ventricles and into the arteries. This is known as the cardiac cycle Contains two parts ○ Diastole: when the ventricles are relaxed and filling with blood ○ Systole: when the ventricles are contracting and pushing blood into the arteries There are four main phases in the cardiac cycle Ventricle filling ○ During diastole: atria and ventricles fill passively ○ AV valves are open ○ SA node and atrial depolarization at the end Called the atrial systole ○ Due to the high pressure the last of the blood is driven from the atria into the ventricles Isovolumetric contraction ○ First part of the systole ○ Depolarization has spread to the ventricles ○ High pressure causes the AV valves to close Lub sound ○ All the valves are closed so there is no change in the blood volume Ventricular ejection ○ Second part of systole ○ Pressure increases enough to open the SL valves ○ Blood goes from the ventricles to the arteries ○ Increase in arterial pressure Isovolumetric relaxation ○ Diastole ○ Ventricles depolarize and relax ○ SL valves close causing the dub sound ○ AV valve open and the ventricles begin to fill up again Know The isovolumetric phases are short and happen when all the valves are closed, so there is no change in ventricle blood volume. The heart sounds happen when the AV valves close ('lub') and when the semilunar valves close ('dub'). Stroke volume is the total blood released from the left ventricle into the aorta during one heartbeat EDV - ESV End diastolic volume: volume of blood in the ventricle at the end of the diastole phase End systolic volume: volume of blood in the ventricle at the end of the systole phase Systolic pressure vs Diastolic Pressure Systolic pressure is the maximum pressure in the arteries Diastolic pressure is the minimum pressure in the arteries The average pressure is known as the mean arterial pressure (MAP). You should be able to read a diagram of ventricle volume, ventricle pressure and arterial pressure and be able to figure out which phase of the cardiac cycle the heart is in at different points. Module 20: Blood Pressure The mean arterial pressure (MAP) is the average pressure in the systemic arteries and it is highly regulated by the body, so there is always enough pressure to get the blood to all our tissues. Average pressure between diastolic and systolic pressures MAP = cardiac output x total peripheral resistance MAP = HR x SV x TPR MAP = EDV-ESV ○ Cardiac output is the volume of blood pumped out of the left ventricle per minute (HR x SV) ○ TPR: the total resistance in the blood vessels in the circuit Heart rate is regulated by the autonomic nervous system by changing the frequency of action potentials in the SA node Parasympathetic: pacemakers (AV and SA node) ○ Decreased the frequency of action potentials in the SA nodes ○ Acetylcholine binds muscarinic AChR in the SA node activating G-proteins Sympathetic: pacemakers and ventricle muscle ○ Increases action potentials in the SA node ○ NE binds beta adrenergic receptors in SA nodes ○ Activates the cAMP pathway causes release of Ca2+ that causes the muscles to contract with greater force and push out more of the 02 Stroke volume is determined by the contractility of the ventricles and venous return. The sympathetic nervous system regulates these factors. Decreasing ESV can increase SV ○ Sympathetic neurons project to the ventricle heart muscles ○ NE binds receptors and activates the cAMP pathway ○ Parasympathetic DOES NOT affect stroke volume Increasing EDV ○ Venous return increase → increased end diastolic volume in the ventricles → increased stroke volume Total peripheral resistance is the total resistance in the blood vessels of the systemic circuit and it is regulated in the arterioles by the sympathetic nervous system. Three ways arteriole resistance is controlled ○ Local control based on tissue needs (O2 and CO2 levels can affect vasodilation) ○ Hormones ○ Sympathetic nervous system (release NE that makes the muscles contract) Parasympathetic DOES NOT affect TPR Baroreceptors sense blood pressure and trigger the baroreceptor reflex to maintain homeostasis of blood pressure The sensory neurons for blood pressure Maintain a near constant blood pressure very critical Located in the aorta and the carotid artery that is in the neck Pathway ○ Increased arteriole pressure → increased stretch in arteriole walls → sensed by baroreceptors → increased action potential (AP) frequency of baroreceptors Information from the baroreceptors goes to the cardiovascular control center that is located in the medulla oblongata It regulates the ANS Baroreceptor thing is a negative feedback loop Chronic high blood pressure (hypertension) can lead to heart attacks and stroke. Medicines that affect the autonomic nervous system are effective treatments for high blood pressure. Module 21: Ventilation The main function of the respiratory system is to bring oxygen into the body and get rid of carbon dioxide. Other main functions O2 into the body and CO2 out of the body Regulate pH Protect from inhaled pathogens Vocalization The respiratory system consists of tubes (trachea, bronchi and bronchioles) and air sacs called alveoli. There are two types of alveolar cells - Type I are thin epithelial cells that the gases diffuse through and Type II cells secrete surfactant. Nose/mouth → pharynx → larynx → trachea → bronchi → bronchioles Bronchioles terminate in the alveolar ducts Two cell types ○ Type 1: makes up the single layer epithelium ○ Type 2: are also in the alveoli but they secrete surfactant The diaphragm sits under the lungs and is a skeletal muscle. It is considered an inspiratory muscle, along with the rib muscles, because they cause inhalation when they contract. Surrounding the lungs and the ribs are the intercostal muscles Diaphragm sits under the lungs and is in part responsible for the way that the lungs inhale/exhale Air flows from high pressure to low pressure, so in order to bring air into the lungs, we need to decrease the pressure in the alveoli by increasing the lung volume. Inhale when Patm>Palv Exhale when Palv>Patm Lung volume changes before or after you inhale/exhale Inhalation ○ Diaphragm contracts and flattens ○ Intercostal muscles contract, expanding the chest ○ Increased lung volume → decreased Palv → air flows in, increasing the pressure ○ When Palv = Patm then the inhale stops Exhalation ○ Expiration is passive ○ Diaphragm and ribs relax ○ Lungs are elastic so they recoil back to their starting position ○ Decreased lung volume → increased Palv → air flows out Lung compliance is a measure of the ability of the lungs to stretch during an inhale. Higher lung compliance means thats its easier to inhale/exhale cause the lungs can scratch out Diseases can affect lung compliance. Surfactant helps decrease surface tension in the alveoli, which increases lung compliance. Scar tissue: makes it hard to inhale because there is so much dense tissues that is surrounding the lungs Emphysema: loss of elastic fibers makes it hard to breathe because its very easy to inhale but the lungs don't recoil so you can’t exhale Surfactant is a mixture of lipids and proteins ○ Lowers surface tension and increased the overall lung compliance The sympathetic nervous system causes bronchodilation which opens up the airways and parasympathetic nervous system causes bronchoconstriction which closes the airways. Bronchoconstriction: smooth muscle that surround the bronchioles contracts ○ Parasympathetic nervous system ○ Increase resistance ○ Decrease air flow Bronchodilation: sympathetic nervous system induces an effect that does the opposite ○ Smooth muscle around the bronchioles relaxes ○ Decrease resistance ○ Increase air flow The autonomic nervous system does not cregulate breathing rate Module 22: Gas Exchange and Transport Concentration of gases is measured as partial pressures. Gases diffuse from high to low partial pressure (just like any other molecule diffusing down its concentration gradient) Partial pressure: the proportion of the total pressure that is due to the presence of a specific gas In the lungs, CO2 diffuses from the deoxygenated blood into the alveoli. O2 diffuses from the alveoli into the blood, making it 100% oxygenated. O2 is out of the alveoli and into the blood ○ Decreases local concentration in the alveoli Fresh air high PO2 In the other tissues, CO2 diffuses from high levels in the cell into the blood O2 follows its partial pressure gradient and diffuses from the blood into the cells. O2 is transported in the blood bound to hemoglobin in red blood cells. In oxygenated blood, hemoglobin is 100% saturated At rest, only about 25% of oxygen is unloaded to tissues Hemoglobin has four subunits each ○ Each can bind one oxygen ○ Binds to the iron in the heme group % Hb saturation = (O2 bound to the Hb/Total O2 binding sites)*100 Temperature, CO2 levels and pH can change the affinity of hemoglobin for oxygen, affecting how much O2 gets unloaded to the tissues. Move the saturation curve to the left: Hb has a higher affinity for oxygen Curve shifts right: Hb has a lower affinity for oxygen doesn’t bind as strongly and is unloaded more easily Most CO2 is converted into bicarbonate by the enzyme carbonic anhydrase in red blood cells. Bicarbonate can travel in the blood directly, and gets converted back to CO2 in the lungs Red blood cells express an enzyme called carbonic anhydrase (CA) ○ CO2 → bicarbonate ○ When carbonate is made H+ is also made, which can affect pH In tissue the CO2 diffuses back into the blood, and then travels the body In the lungs the reaction reverses ○ CO2 made in the RBC’s from bicarbonate and CO2 then diffuses out of the blood Module 23: Urinary System The kidneys filter the blood to help regulate ion concentration and blood osmolarity. The kidneys make urine to get rid of material from the blood. Main functions ○ Regulate the concentration of ions in the blood plasma ○ Maintain water balance (osmoregulation) Other functions ○ Regulation of ions in the plasma (Na+, Ca+2, K+, Cl-, Mg+2) ○ Regulation of blood volume and blood pressure ○ Regulation of plasma osmolarity (water conservation) ○ Regulation of blood pH (via H+ and HCO3-) ○ Removal of waste (metabolic waste like urea, foreign substances) ○ Produce EPO (hormone), renin, vitamin D3 and glucose via gluconeogenesis The functional unit of the kidney is a tube called the nephron. Blood gets filtered into the nephron and molecules are secreted or reabsorbed back into the blood throughout the nephron. Blood enters via the renal artery and leaves via the renal vein Filtered into nephrons (functional unit) Kidney produce urine Renal cortex: outer region Renal Medulla: inner region Nephron Tubule Structure Renal artery → afferent arteriole → glomerulus → efferent arteriole → peritubular capillary (renal cortex, OUTER) and vasa recta (loop of henle) → renal vein Tubule Structure ○ Bowman's capsules (glomerulus) → proximal tubule → loop of Henle (descending limb + ascending limb) → distal tubule → collecting duct → renal pelvis Juxtaglomerular apparatus ○ Macula densa: cluster of cells in the distal that sense the Na+ concentration in the filtrate ○ Granular cells: in the wall of the afferent arteriole secrete renin Filtration is non-specific -- all small molecules get filtered into the nephron Hooray! Occurs from glomerulus to Bowman’s capsule Three exchange processes for renal exchange ○ Filtration ○ Reabsorption ○ Secretion Glomerular Filtration Rate The rate at which substances are filtered through the glomerulus It's used to diagnose chronic kidney disease eGFR can be calculated using the amount of creatinine in the urine Creatinine is freely filtered but not absorbed but rather secreted into the nephron Equations have traditionally taken race unto account which is not a helpful part of the process AT ALL and often led to patients inability to get access to kidney transplants Glucose gets filtered into the nephron, but it is completely reabsorbed back into the blood in the proximal tubule. Reabsorption is the movement of a molecule from the nephron back into the blood K+, H+ and foreign substances in food are all secreted from the blood into the nephron ALL glucose in a healthy individual is reabsorbed into the blood ○ Glucose in pee is a sign of diabetes ALL glucose is reabsorbed in the proximal tubule Most water gets reabsorbed in the proximal tubule, but reabsorption of water can only be regulated in the collecting duct by the hormone ADH, based on the body's needs. Water is absorbed all throughout the tubule but most is in the proximal tubule Only place that the body can regulate the reabsorption of water is in the collecting ducts Its regulated by the hormone ADH (antidiuretic hormone) that comes from the posterior pituitary ADH controls the amount of aquaporins are put in the membrane of the tubule epithelial cells Na+ reabsorption is regulated by the steroid hormone aldosterone acting on the distal tubule. Ion concentrations in the blood are also regulated in the kidneys via reabsorption Na+ gets transported via active and passive transport Na+ reabsorption in the distal tubule is regulated by the steroid hormone aldosterone In summary Glucose is reabsorbed only in the proximal tubule Water and Na+ are reabsorbed most places Water reabsorption is regulated in the collecting duct by ADH Na+ reabsorption is regulated in the distal tubule by aldosterone. Module 24: Osmoregulation The kidneys regulate water balance and blood osmolarity, which is called osmoregulation. It is important to maintain the blood osmolarity within a normal range (around 300 mOsm) Body is in balance if ○ Ingested water + water from metabolism = water output + water used in reactions If you are dehydrated, the kidneys will produce less urine to conserve water. If you are overhydrated, the kidneys will produce more urine to get rid of the excess water. Also get rid of water via sweat and feces Only way to increase water in the blood is to drink more water Water reabsorption in the collecting duct is regulated by the hormone antidiuretic hormone (ADH) released by the posterior pituitary. Water will want to flow from the dilute filtrate to the more concentrated interstitial fluid and plasma ○ Hyposmotic to hyperosmotic ADH causes more aquaporins to be inserted into the membrane, increasing water reabsorption. Only happens in the collecting ducts Osmoreceptors are sensory neurons in the hypothalamus that measure blood osmolarity. Located in the hypothalamus When blood osmolarity is high (dehydrated) the osmoreceptors will signal the release of ADH ○ Does not bring the osmolarity down but it prevents it from going more up If the blood osmolarity is low (overhydrated) the osmoreceptors will fire fewer APs and less ADH will be released ○ Less aquaporins, less water will be reabsorbed ○ More pee trying to rehydrate Module 25: Renal Control of Blood Pressure Aldosterone (steroid hormone) regulates Na+ reabsorption in the distal tubule when Na+ levels are low Aldosterone is released from the adrenal cortex Steroid hormone ○ Lipophilic and requires a carrier protein Aldosterone release is regulated by another hormone called Angiotensin II (peptide hormone), which is synthesized by two enzymes - renin and ACE (this pathway is known as RAAS) Aldosterone is a part of the RAAS pathway Renin: an enzyme and not a hormone that is secreted into the bloodstream by the granular cells in the afferent arterioles When Na+ concentration in filtrate decreases the macula densa cells (distal tubule) sense it and tell the granular cells (afferent arteriole) to release renin Renin release into the blood is the key regulatory step in this pathway. Renin is released from granular cells in the juxtaglomerular apparatus when Na+ levels are low, or when the sympathetic nervous system signals the granular cells Renin catalyzes the reaction of angiotensinogen (inactive) to angiotensin I (inactive) Another enzyme called ACE (angiotensin converting enzyme) turns that angiotensin I (inactive) into angiotensin II (active yay) Renin is key because angiotensinogen and ACE are literally always there ENZYMES ARE PROTEINS Angiotensin II regulating aldosterone release causes the release of ADH to regulate water reabsorption Angiotensin II increasing MAP Ang II signaling in the hypothalamus makes you feel thirsty → increased water intake Vasoconstriction → increasing TPR and overall MAP Release of aldosterone → increased Na+ reabsorption A number of hypertension medications inhibit the RAAS pathway, most commonly ACE inhibitors. Anything that inhibits ace is the most common thing that would help slow the RAAS pathway or inhibit the RAAS pathway Module 26: Digestive System The digestive system breaks down the food we eat with enzymes secreted by the accessory glands, and absorbs nutrients into the body Other functions ○ Absorption - small molecules from food move from lumen of intestines into internal environment ○ Secretion - enzymes and hormones are secreted by cells to aid with digestion and absorption ○ Motility - smooth muscle helps get digested food through GI tract Made up of two divisions ○ Gastrointestinal tract: where the food travels Mouth → pharynx → esophagus → stomach → small intestine (duodenum, jejunum, ileum) → colon (large intestine) → rectum anus ○ Accessory glands: secrete fluid and enzymes into the GI tract Salivary glands, liver, gallbladder, and pancreas Salivary glands secrete saliva into the mouth to help lubricate the food during chewing, so it can be swallowed. Carbohydrate digestion starts in the mouth using salivary amylase Saliva contains ○ Water to moisten ○ Mucus to lubricate the food ○ Bicarbonate HCO3 to neutralize acid in foods ○ Lysozyme: enzyme that destroys bacteria ○ Salivary amylase: breaks down starch Three distinct layers in the walls of the GI tract Epithelial cells on the interior do exchange between external environmental and internal environment There are smooth muscle around the GI tract that aid in mobility There are nerves in the GI tract that are known as the enteric nervous system ○ All of the changes in the digestive system are monitored by the parasympathetic nervous system as part of the ANS The stomach secretes acid into the lumen to protect the body from pathogens and to denature proteins in the food. The stomach also secretes pepsin, which is a protease that starts the process of protein digestion Pepsin is secreted from the walls of the stomach in an inactive form called pepsinogen and then the HCl in the stomach activates it so it can do its job Secretion of everything from the the stomach lining is regulated by the PNS Most digestion occurs in the first segment of the small intestines, called the duodenum. The pancreas secretes enzymes into the duodenum, and the liver/gallbladder secrete bile salts. Most digestion occurs in the duodenum because the pancreatic enzymes and biles from the liver are all secreted there Small intestine is good at absorption because it has a very large surface area Villi increase surface area and aid in fat absorption Pancreas secretes enzymes into the duodenum ○ Amylase: digests starch ○ Lipase: digests fats ○ Proteases: digests proteins Carbohydrates are further digested by pancreatic amylase and brush border enzymes in the membrane of the epithelial cells in the small intestines. Proteins are digested by proteases secreted by the pancreas. Carbs are further digested with pancreatic amylase in the small intestine These enzymes continue working until the digesting into monosaccharides is finished Transporters pump the sugar monomers into the cell and then the interstitial fluid Amino acids and monosaccharides are absorbed from the small intestines into the blood and go to the liver first before entering general circulation. Travel via the hepatic portal vein then the heart ○ Portal system Liver performs a general filtering of the materials to make to remove any harmful toxins Fats are broken down by bile salts and lipase in the duodenum before forming chylomicrons in the epithelial cells. Most fat digestion occurs in the small intestine Lipids from large droplets that float on top of the chyme Bile breaks down fat globules ○ Bile salts are a part of the bile ○ Stored in the gallbladder Once inside of the epithelial cell in the bloodstream they get repackaged into large molecules Water has to be reabsorbed in large amounts throughout the small and large intestines. We ingest 2L of water ish a day but we secrete an additional 7L of water into the GI tract (saliva, juice, bile) Most is reabsorbed in the small intestines ○ Passively occurs via osmosis Module 27: Metabolism and Diabetes After a meal, the body converts excessive nutrients into glycogen and fat storage. Metabolism is the collection of all the chemical reactions that are occuring in our bodies at one time This is known as the absorptive state (fed state) ○ Food is being digested, absorbed, used, and stored Excess molecules are converted into larger polymers such a glycogen and fat ○ Glycogenesis: synthesis of glycogen from glucose ○ Lipogenesis: synthesis of fat storage from fatty acids After a couple of hours of fasting, the body breaks down glycogen and fat storage to provide more sugar and fatty acids for our cells to use as energy. This is called the postabsorptive state After nutrients form a meal are no longer in your bloodstream ○ Body turns to stored energy for energy ○ Glycogenolysis: breakdown of glycogen ○ Lipolysis: breakdown of fat When blood sugar levels are high, the beta cells in the pancreas release insulin. Insulin signals our cells to take up glucose and to form glycogen, which brings glucose levels back down Pancreas contains the beta and alpha cells ○ Beta cells: insulin ○ Alpha cells: glucagon Regulation of insulin ○ Insulin binds receptors in many cells ○ Causes an increased synthesis of GLUT4 transporters as well as insertion of stored transporters into the membrane ○ Glucose can only enter cells if there are transporters in the membrane ○ Increased GLUT4 transporters in the membrane will lead to increased glucose uptake from the blood Blood glucose will decrease (negative feedback) Insulin also regulates enzymes that are essential for When blood sugar levels are low, the alpha cells in the pancreas release the hormone glucagon, which signals the breakdown of glycogen. Released from alpha cells in the pancreas Released when blood glucose levels fall Glucagon acts on the liver to stimulate the breakdown of glycogen (glycogenolysis) AND the production of glucose from other molecules like amino acids, lactate, and glycerol In someone with type I diabetes, the immune system attacks beta cells, decreasing insulin production. This can be treated by taking synthetic insulin. Appears before the age of 20 Immune disorder that attacks beta cells There is simply no insulin that is made Must inject with synthetic insulin, the patient become the integrating center The causes of type II diabetes are complex and not entirely understood, but we do know that the insulin receptor becomes less sensitive, decreasing insulin signaling. This is the most common type of diabetes. Receptor becomes less sensitive to stimuli At first the body compensates by releasing more and more insulin and then after some time less insulin will be released The early symptoms of hyperglycemia caused by diabetes are glucose in the urine, polyuria and excessive thirst. Normal glucose concentrations the blood has no issues reabsorbing all of the glucose that is in the nephron The transporters in the proximal tubule have absolutely got it When blood glucose is to high transporters become fully saturated and not all of the glucose goes back into the blood If diabetes progresses, it can cause damage to blood vessels, neurons, the heart and kidneys. Glucose can get chemically bonded to blood proteins which affects the ways proteins function ○ Leads to an inability for blood to clot ○ Cell damage Damages blood vessels ○ Leads to blindness and amputations Module 28: Exercise Physiology The physiological responses to exercise help bring more oxygen and energy to the working muscles. This makes it so we can produce ATP ○ We need O2 and energy such a glucose or fat So all of our exercise increase are designed to increase the delivery of these muscles Intensity is measured in %VO2max ○ Max amount of oxygen that can be used during the most intense exercise Chemoreceptors sense O2, CO2 and pH and signal the respiratory control center to increase the breathing rate during exercise. Sense gas levels Located in the medulla oblongata and the pons ○ Respiratory control centers Neurons fire action potentials rhythmically without conscious input When Pco2 levels are high the blood pH decreases (more acidic) Two types of Chemoreceptors ○ Peripheral chemoreceptors: located in the carotid artery Mainly sense pH but also O2 and CO2 ○ Central Chemoreceptors: medulla oblongata sense pH only O2 levels decrease and CO2 levels increase when you’re exercising ○ Activates the chemoreceptors SYMPATHETIC NERVOUS SYSTEM IS NOT INVOLVED During exercise the sympathetic nervous system is activated, which helps increase cardiac output, so more blood can go to the active tissues. Venous return increases Increases SV which increases the cardiac output in one beat LARGE increase in stroke volume Active muscles release paracrine signals, which cause local vasodilation, so more blood will flow to them. Active muscles sense paracrine signals which cause local vasodilation and make more blood flow to them to increase O2 and CO2 This causes a decrease in total peripheral resistance during exercise This also causes the inactive muscles to receive a decrease in blood flow because there is no signal When muscles are at rest they store energy in creatine phosphate, which can provide enough ATP for the first minute of exercise. Steps in how we power through exercise: ONE Creatine phosphate helps the body through the first minute of exercise When muscles are active the phosphate is removed and ATP is created This provide enough ATP to make it through the first minute or so of exercise TWO Aerobic and anaerobic respiration ○ Start of exercise we rely on anaerobic respiration (GLYCOLYSIS) until the breathing rate increases enough to start to match the oxygen usage ○ anaerobic respiration Produces to ATP per glucose that is broken into pyruvate THREE As we continue to exercise ventilation and CO increase more O2 to muscles This makes it so muscles can increase increase aerobic respiration using stored glycogen and fat ○ Happens in the mitochondria and makes much more ATP per glucose Know the graph that is below Fat is the main energy source for low intensity exercise, whereas carbohydrates are used during high intensity exercise. Fat stores more energy than glucose but is slower to breakdown Due to this fat is used more at low intensity exercise and then as intensity increases energy comes from carbs Exercise affects all different parts of the body and has a number of benefits for the different organ systems. Lowers the risk of cardiovascular disease Exercise helps counteract type II diabetes Boost your immune system Help you deal with stress and depression