DNA Replication Lecture 3 PDF
Document Details
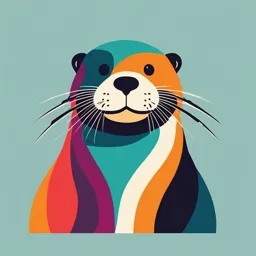
Uploaded by EndearingBlack5833
Tags
Related
- University of Canterbury BIOL/BCHM 111 Cell Biology Test 2023
- Molecular Biology of The Cell: Chapter 5 - DNA Replication, Repair, and Recombination PDF
- Molecular Biology of The Cell, Chapter 5 Part 2 PDF
- Life Sciences I - Cell Biology Lecture 2 PDF
- Replication in Prokaryotes (E-Coli) PDF
- Nuclear and Chromosomal Structure - Molecular Biology PDF
Summary
This document appears to be a lecture presentation on DNA replication. It covers several aspects of the process including base-pairing, origin of replication, different models of replication, and the role of various enzymes. Diagrams related to DNA Replication. It includes details such as equilibrium density centrifugation and the concept of telomeres and telomerase. This is likely an educational resource for undergraduate students.
Full Transcript
BIOM-2131 Introductory Molecular Biology LECTURE 3 DNA Replication LECTURE OVERVIEW ▪ 3.1 Base-pairing enables DNA replication ▪ 3.2 DNA synthesis begins at replication origins ▪ 3.3 Semiconservative...
BIOM-2131 Introductory Molecular Biology LECTURE 3 DNA Replication LECTURE OVERVIEW ▪ 3.1 Base-pairing enables DNA replication ▪ 3.2 DNA synthesis begins at replication origins ▪ 3.3 Semiconservative replication of DNA ▪ 3.4 DNA replication ▪ 3.5 Telomere and Telomerase DNA Replication the ability of cells to survive and proliferate requires accurate duplication of its genetic information DNA replication must occur before a cell can divide to produce two genetically identical daughter cells DNA is replicated during synthesis phase Base-pairing enables DNA replication each strand of a DNA double helix contains a sequence of nucleotides that is exactly complementary to the nucleotide sequence of its partner stand each strand acts as a template for producing a complementary strand Alberts, et. al, Essential Cell Biology, 6th edition, Figure 6-2, p. 210 DNA Replication the ability of each strand of a DNA molecule to act as a template for producing a complementary strand enables a cell to copy, or replicate, its genes copying billions of nucleotides with incredible speed and accuracy cluster of proteins that form together a replication machine Alberts, et. al, Essential Cell Biology, 6th edition, Figure 6-3, p. 211 DNA copying mechanisms? three models of DNA replication make different predictions Watson and Crick Delbruck model #3 1953 Alberts, et. al, Essential Cell Biology, 6th edition, Figure 6-5, p. 212 Labelling of DNA with stable isotopes Meselson and Stahl E. coli heavy 15N-containing DNA light 14N-containing DNA cg in a CsCl gradient allows the separation of heavy and light DNA equilibrium density centrifugation Alberts, et. al, Essential Cell Biology, 6th edition, Figure 6-6, p. 213 Test out various DNA replication hypothesis Alberts, et. al, Essential Cell Biology, 6th edition, Figure 6-7 (partial), p. 214 Test out various DNA replication hypothesis one generation of growth DNA hybrid: heavy and light isotopes Alberts, et. al, Essential Cell Biology, 6th edition, Figure 6-7 (partial), p. 214 Test out various DNA replication hypothesis distinguish between semiconservative vs dispersive model subject DNA to high temperature H-bonds holding two strands together break DNA helix comes apart heat DNA hybrid before cg one strand heavy, other strand light DNA synthesis begins at replication origins DNA double helix is normally very stable opening up the DNA double helix thermal energy (temp ~ boiling H2O) action of initiator proteins bind to specific DNA sequences called replication origins replication origins bacteria circular chromosome - 1 human genome ~ 10,000 origins Alberts, et. al, Essential Cell Biology, 6th edition, Figure 6-4, p. 211 ~ 220 per chromosome Two replication forks form at each replication origin DNA synthesis occurs at Y-shaped junctions called replication forks two replication forks are formed at each replication origin at each fork, a replication machine moves along the DNA using each strand as the two forks move a template to make a new away from the origin daughter strand in opposite direction 1,000 nt/s bacteria bidirectional replication 100 nt/s humans Alberts, et. al, Essential Cell Biology, 6th edition, Figure 6-8, p. 215 Bidirectional replication Alberts, et. al, Essential Cell Biology, 6th edition, Figure 6-9 (partial), p. 215 DNA polymerase synthesizes DNA using a parent strand as a template replication machine DNA polymerase uses one of the parent DNA strands as a template catalyzes addition of nucleotides to the 3’ end of growing DNA strand synthesises the new strand in 5’-to-3’direction Alberts, et. al, Essential Cell Biology, 6th edition, Figure 6-10, p. 216 DNA polymerase synthesizes DNA using a parent strand as a template DNA polymerase catalyzes formation of phosphodiester bond between the 3’ end of the growing DNA chain and the 5’-phosphate group of the incoming nucleotide, which enters the reaction as deoxyribonucleoside triphosphate pyrophosphate is further hydrolyzed to two molecules of phosphate makes the polymerization reaction effectively irreversible DNA polymerase adds a deoxyribonucleotide to the 3’ end of a growing DNA strand Alberts, et. al, Essential Cell Biology, 6th edition, Figure 6-11 (partial), p. 216 DNA polymerase adds a deoxyribonucleotide to the 3’ end of a growing DNA strand x-ray structure of DNA polymerase from E. coli Alberts, et. al, Essential Cell Biology, 6th edition, Figure 6-11 (partial), p. 216 The replication fork is assymetrical each strand of DNA has polarity the two strands are antiparallel as a consequence, at each replication fork one new DNA stand is made on a template that runs in one direction (3’ to 5’) whereas the other new strand is being made on a template that runs in the opposite direction (5’ to 3’) DNA polymerase functions only in 5’ → 3’ direction Alberts, et. al, Essential Cell Biology, 6th edition, Figure 6-12, p. 217 Strand synthesis by DNA polymerase DNA polymerase functions only in 5’ → 3’ direction copies the 3’ to 5’ template strand continuously leading strand copying from the other template strand occurs discontinuously, in successive, separate, small pieces lagging strand Okazaki fragments backstitching mechanism Google search Semiconservative DNA Replication Quizlet Strand synthesis at each replication fork Alberts, et. al, Essential Cell Biology, 6th edition, Figure 6-13, p. 217 DNA polymerase is self-correcting DNA polymerase makes about 1 error in 107 nucleotide pairs it copies DNA polymerase properties that increase the accuracy of DNA replication carefully monitors the base-pairing between each incoming nucleotide triphosphate and the template strand when it makes a rare mistake and adds the wrong nucleotide it can correct the error through proofreading DNA polymerase proofreads its own work during DNA synthesis DNA pol contains separate sites for DNA synthesis (P: polymerization) and proofreading (E: editing activity) concurrent process Alberts, et. al, Essential Cell Biology, 6th edition, Figure 6-14 and 6-15, p. 218 3’ → 5’ exonuclease activity Initiation of DNA synthesis requires the accuracy of DNA replication depends on the requirement of the DNA pol for correctly base-paired 3’ end before it can add more nt to the growing DNA strand how then can the polymerase begin a completely new DNA strand? different enzyme is needed to get the process started use DNA template to make RNA fragment primase, RNA polymerase Initiation of DNA synthesis requires complementary, short lengths of RNA ~10 nt acts as primer for DNA polymerase primase can start a new polynucleotide chain by joining together two ribonucleoside triphosphates without the need for base-paired 3’ end as a starting point one RNA primer leading strand Alberts, et. al, Essential Cell Biology, 6th edition, Figure 6-17, p. 220 Multiple enzymes are required for… … DNA synthesis from lagging strand template discontinuous DNA synthesis new RNA primers must be laid down at intervals along the newly exposed, ssDNA stretch DNA polymerase adds deoxyribonucleotide to the 3’ end of each new primer to produce Alberts, et. al, Essential Cell Biology, 6th edition, Figure 6-18 (partial), p. 220 Okazaki fragments Multiple enzymes are required … to produce a continuous new DNA strand from the many separate pieces of nucleic acid three additional enzymes: nuclease degrades RNA primer repair polymerase replaces RNA primer with DNA proofreading activity DNA ligase join remaining fragments Alberts, et. al, Essential Cell Biology, 6th edition, Figure 6-18 (partial), p. 220 together DNA Ligase joins the 5’-phosphate end of one DNA fragment to the adjacent 3’-hydroxyl end of the next joins together Okazaki fragments on the lagging strand during DNA synthesis Alberts, et. al, Essential Cell Biology, 6th edition, Figure 6-20, p. 221 Proteins at replication fork cooperate to form a replication machine DNA replication requires the double helix to be continuously pried apart DNA helicase unwinding of the parent DNA double helix ATP dependent Single-strand DNA binding proteins latch onto ssDNA prevent reforming of double helix and keeping them in elongated form Replication Machine DNA synthesis is carried out by a group of proteins that act together DNA polymerase 5’-to-3’ direction simplified diagram sliding clamp keeps DNA pol attached to DNA template Alberts, et. al, Essential Cell Biology, 6th edition, Figure 6-20 (partial), p. 221 Replication Machine DNA synthesis in cells proteins held together in a large replication machine clamp loader locks sliding clamp around newly formed DNA double helix ATP dependent Alberts, et. al, Essential Cell Biology, 6th edition, Figure 6-20 (partial), p. 221 Does localized unwinding of DNA double helix present a problem? as helicase moves forward, prying open the double helix, the DNA ahead of the fork gets wound more tightly if tension in the DNA allowed to build up, it would ultimately impede the forward movement of the replication machinery Alberts, et. al, Essential Cell Biology, 6th edition, Figure 6-21 (partial and modified), p. 222 DNA Topoisomerases enzymes that relieve the tension that builds up in front of a replication fork DNA topoisomerase: produce a transient, single-stranded break in the DNA backbone, which releases the build-up tension reseals the nick before falling off the DNA Alberts, et. al, Essential Cell Biology, 6th edition, Figure 6-21 (partial), p. 222 Nuclease Repair polymerase DNA replication in living cells https://dnalc.cshl.edu/resources/3d/04- mechanism-of-replication-advanced.html Telomerase replicates the ends of eukaryotic chromosomes at the end of linear chromosomes…. leading strand can be replicated all the way to the chromosome tip lagging strand cannot when the final RNA primer is removed, there is no enzyme that can replace it with DNA Alberts, et. al, Essential Cell Biology, 6th edition, Figure 6-22, p. 222 Dealing with ‘end-replication problem’ telomeres long, repetitive nucleotide sequences present at the ends of every chromosome provide replication machinery with “extra” DNA to complete the lagging strand telomerase carries its own RNA template uses the RNA primer to add multiple copies of the same repetitive DNA sequence to the lagging-strand template Telomeres and telomerase Alberts, et. al, Essential Cell Biology, 6th edition, Figure 6-23, p. 224 Telomeres allow replication of chromosome ends form structures that mark the true ends of a chromosome these structures allow cells to distinguish unambiguously between the natural ends of chromosomes and the double-strand DNA breaks that sometimes occur accidently in the middle of the chromosomes must be immediately repaired Telomeres and telomerases prevent linear eukaryotic chromosomes from shortening after each cell division repetitive DNA sequences attract other telomere-binding proteins not only protect chromosome ends, but maintain telomere length Telomeres and telomerases telomere length varies by cell type and with age rapidly dividing cells keep their telomerase fully active many other cell types gradually reduce their telomerase activity after many rounds of cell divisions, the telomeres in these descendant cells will shrink, until they essentially disappear at this point, the cell will cease dividing Lecture 3 Reading Chapter 6: DNA Replication and Repair pages 179-225 Upcoming: Lecture 4 Reading Chapter 6: DNA Replication and Repair pages 225-236 Room change for Labs 55 and 60 please see UWinsite student for updated rooms