Molecular Biology of The Cell: Chapter 5 - DNA Replication, Repair, and Recombination PDF
Document Details
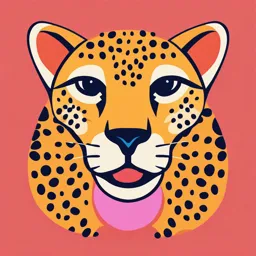
Uploaded by CalmFairy
جامعة البترا-الأردن & كلية الطب-جامعة الأزهر-مصر
Alberts et al.
Tags
Related
- Becker's World of the Cell, Tenth Edition - Chapter 17 PDF
- Chapter 4 Part I DNA Biology & Cell Division PDF
- Module 1 PPT PDF
- Molecular Biology and Genetics - Explorations: An Open Invitation to Biological Anthropology (2nd Edition) PDF
- DNA Structure and Replication PDF
- Replication in Prokaryotes and Eukaryotes PDF
Summary
This document is Chapter 5 from the seventh edition of the Molecular Biology of the Cell textbook, focusing on DNA replication, repair, and recombination. It provides explanations and diagrams about various mechanisms involved in maintaining accurate DNA sequences. The processes of DNA replication and repair are detailed.
Full Transcript
Chapter 5 DNA Replication, Repair, and Recombination Copyright © 2022 W. W. Norton & Company, Inc. The Maintenance of DNA Sequences Mutation Rates Are Extremely Low Low Mutation Rates Are Necessary for Life as We Know It The Maintenance of DNA Sequences In all cells, DNA sequences are maintained and...
Chapter 5 DNA Replication, Repair, and Recombination Copyright © 2022 W. W. Norton & Company, Inc. The Maintenance of DNA Sequences Mutation Rates Are Extremely Low Low Mutation Rates Are Necessary for Life as We Know It The Maintenance of DNA Sequences In all cells, DNA sequences are maintained and replicated with extremely high fidelity(precision). The mutation rate, approximately one nucleotide change per 1010 nucleotides each time the DNA is replicated, is very similar for organisms as different as bacteria and humans. Remarkable accuracy: the sequence of the human genome (approximately 3.1 X 109 nucleotide pairs) is unchanged or changed by only a few nucleotides each time a typical human cell divides. This allows humans to pass accurate genetic instructions from one generation to the next and also— for most of us—to avoid the changes in somatic cells that lead to cancer. The Germ cells must be protected against high rates of mutation to maintain the species. the somatic cells of multicellular organisms must also be protected from genetic change to properly maintain the organized structure of the body. DNA Replication Mechanisms A Sliding Ring Holds a Moving DNA Polymerase Onto the DNA The Proteins at a Replication Fork Cooperate to Form a Replication Machine DNA Replication Is Fundamentally Similar in Eukaryotes and Bacteria A Strand-directed Mismatch Repair System Removes Replication Errors That Remain in the Wake of the Replication Machine The Accidental Incorporation of Ribonucleotides During DNA Replication Is Corrected DNA Topoisomerases Prevent DNA Tangling During Replication DNA Replication Mechanisms Base-pairing Underlies DNA Replication and DNA Repair DNA Replication Mechanisms: The DNA Replication Fork Is Asymmetrical DNA Replication Mechanisms The High Fidelity of DNA Replication Requires Several Proofreading Mechanisms The High Fidelity of DNA Replication The high fidelity of DNA replication, depends on: the initial base-pairing. several “proofreading” mechanisms; to correct any initial mispairings that might have occurred. DNA polymerase performs the first proofreading step just before a new nucleotide is covalently added to the growing chain. After complementary nucleotide binding, but before the nucleotide is covalently added to the growing chain, the enzyme must undergo a conformational change in which its “grip” tightens around the active site. Because this change occurs more readily with correct than incorrect basepairing, it allows the polymerase to “double-check” the exact base pair geometry before it catalyzes the addition of the nucleotide. Incorrectly paired nucleotides are harder to add and therefore more likely to diffuse away before the polymerase can mistakenly add them. The next error-correcting reaction is : exonucleolytic proofreading, takes place immediately after those rare instances in which an incorrect nucleotide is covalently added to the growing chain. 3’to-5’proofreading exonuclease : DNA polymerase molecules correct such a mismatched primer strand by means of a separate catalytic site (either in a separate subunit or in a separate protein domain of the polymerase molecule, depending on the polymerase). This clips off any unpaired or mispaired residues at the primer terminus, continuing until enough nucleotides have been removed to regenerate a correctly base-paired DNA polymerase functions as a “self-correcting” enzyme that removes its own polymerization errors as it moves along the DNA. DNA polymerase can’t start DNA synthesis de novo without an existing primer. DNA Replication Mechanisms DNA Replication in the 5'-to-3' Direction Allows Efficient Error Correction. A Special Nucleotide-polymerizing Enzyme Synthesizes Short RNA Primer Molecules Special Proteins Help to Open Up the DNA Double Helix in Front of the Replication Fork The DNA double helix is very stable under physiological conditions: the base pairs are locked in place so strongly that it requires temperatures approaching that of boiling water to separate the two strands in a test tube. Two types of replication proteins: DNA helicases and single-strand DNA-binding proteins. DNA Helicase: A special Protein Helps to Open Up the DNA Double Helix in Front of the Replication Fork DNA helicases hydrolyze ATP when they are bound to single strands of DNA. The binding and hydrolysis of ATP can change the shape of a protein molecule in a cyclical manner that allows the protein to perform mechanical work. DNA helicases use this principle to propel themselves rapidly along a single DNA strand. This unidirectional movement can occur at rates of up to 1000 nucleotides per second. The two strands of DNA have opposite polarities, a helicase could unwind the DNA double helix in front of a replication fork by moving either in the 5′-to-3′ direction along one strand or in the 3′-to-5′ direction along the other strand. Single-strand DNA-binding (SSB) proteins; bind tightly and cooperatively to the single-stranded DNA that is produced by helicases. SSB proteins coat and straighten out all regions of single-stranded DNA, thereby preventing the formation of the short hairpin helices that otherwise form in these single strands. These regions occur routinely on the lagging strand template, and if not removed, they can impade(inhibit or delay) the DNA synthesis catalyzed by DNA polymerase. A Sliding Ring Holds a Moving DNA Polymerase onto the DNA DNA polymerase molecules will synthesize only a short string of nucleotides before falling off the DNA template. An accessory protein (called PCNA in eukaryotes) forms a sliding clamp that keeps the polymerase firmly on the DNA when it is moving but releases the polymerase as soon as it runs into a double-strand region of DNA. The three-dimensional structure of the clamp protein revealed that it forms a large ring around the DNA double helix. One face of the ring binds to the back of the DNA polymerase, and the whole ring slides freely along the DNA as the polymerase moves. The assembly of the clamp around the DNA requires a special protein complex, the clamp loader, that can open and close the ring in a regulated manner. The moving DNA polymerase is tightly bound to the clamp, and, on the leading strand, the two remain associated for a very long time. The DNA polymerase on the lagging-strand template also makes use of the clamp, but each time the polymerase reaches the 5′ end of the preceding Okazaki fragment, the polymerase releases itself from the clamp and dissociates from the template. With the help of the clamp loader, which hydrolyzes ATP as it loads a new clamp onto a primer– template junction, this lagging-strand polymerase molecule then associates with the new clamp that is assembled on the RNA primer of the next Okazaki fragment.