DNA Structure and Replication Lecture PDF
Document Details
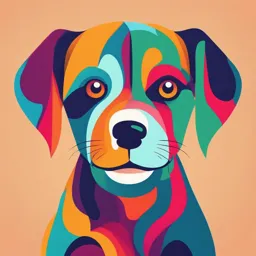
Uploaded by SensationalQuasimodo
Reynaldo O. Tabang Jr., RMT
Tags
Summary
This lecture presents an overview of DNA structure and replication. It details the key experiments and discoveries that led to the understanding of DNA's structure and functions, highlighting the significance of key figures in the field of molecular biology.
Full Transcript
DNA Structure and Replication REYNALDO O. TABANG JR., RMT LEARNING OURCOMES: 1. Describe the Experiments that demonstrated DNA as the Genetic material 2. Explain how Watson and Crick deduced the 3D structure of DNA 3. List the components of a DNA nucleotide 4. Explain how to chains of nucle...
DNA Structure and Replication REYNALDO O. TABANG JR., RMT LEARNING OURCOMES: 1. Describe the Experiments that demonstrated DNA as the Genetic material 2. Explain how Watson and Crick deduced the 3D structure of DNA 3. List the components of a DNA nucleotide 4. Explain how to chains of nucleotides form a DNA molecule 5. Describe how a long DNA molecules folds and loops 6. Explain the semi conservative mechanism of DNA Replication 7. List the steps of DNA replication Deoxyribonucleic acid (DNA) DNA - a polymer of deoxyribo- nucleotides. Usually double stranded. And have double-helix structure. found in chromosomes, mitochondria and chloroplasts. It acts as the genetic material in most of the organisms. Carries the genetic information demonstrated DNA as the Genetic Material Friedrich Meischer Friedrich Miescher discovered DNA in 1869 while studying white blood cells, initially aiming to isolate proteins. Instead, he found a unique substance, "nuclein," later identified as DNA. This pivotal discovery laid the groundwork for understanding DNA as the genetic material. Griffith's Transformation Experiment Griffith's experiment was a landmark(1928): study that provided the first evidence that genetic material could be transferred between bacteria. Transferred killing ability between types of bacteria It laid the foundation for later discoveries about DNA Avery, MacLeod, and McCarty Experiment (1944) This experiment further confirmed Griffith's findings by demonstrating that DNA, not protein, was the transforming principle. They isolated DNA from pathogenic bacteria and showed that it could transform non- pathogenic bacteria. Phoebus Levene (1869- a pioneering biochemist 1940) who made significant contributions to understanding the structure of nucleic acids. He identified the basic building blocks of nucleic acids, called nucleotides, and correctly differentiated the sugars in DNA Erwin Chargaff Chargaff was an Austrian- (1905-2002 American biochemist who conducted experiments on the composition of DNA. He discovered that the amount of adenine (A) in DNA always equals the amount of thymine (T), and the amount of guanine (G) always equals the amount of cytosine (C). These rules were crucial for Maurice Wilkins (1916-2004) Wilkins was a New Zealand- born physicist who worked with Rosalind Franklin at King's College London. He played a key role in developing techniques for X- ray diffraction studies of DNA, which produced the crucial images that revealed the helical structure of DNA. Rosalind Franklin (1920-1958) Franklin was a British chemist and X-ray crystallographer who made groundbreaking contributions to understanding DNA structure. She produced the famous "Photo 51," an X-ray diffraction image of DNA Watson and Crick (1953) James Watson and Francis Crick were American and British scientists, respectively, who used the data from Chargaff's rules and Franklin's X-ray diffraction images to build the first accurate model of DNA's double helix structure. Watson and Crick deduced the double helix structure of DNA using a combination of experimental data and model building. X-ray Diffraction Data: They utilized the X-ray diffraction images of DNA produced by Rosalind Franklin and Maurice Wilkins. These images revealed the helical nature of DNA and provided crucial information about its dimensions. Watson and Crick deduced the double helix structure of DNA using a combination of experimental data and model building. X-ray Diffraction Data: They utilized the X-ray diffraction images of DNA produced by Rosalind Franklin and Maurice Wilkins. These images revealed the helical nature of DNA and provided Watson and Crick deduced the double helix structure of DNA using a combination of experimental data and model They incorporated building. Chargaff's rules, which stated that the amount of adenine (A) in DNA always equals the amount of thymine (T), and the amount of guanine (G) always equals the amount of cytosine (C). This hinted at a complementary Model Building: Using cardboard cutouts representing the DNA components (bases, sugars, and phosphates), they built various models, trying to fit them together based on the available data. Double Helix Model: After numerous trials and errors, they arrived at a double helix model where two strands of DNA run antiparallel to each other, with the bases facing inwards and forming hydrogen bonds between NUCLEIC ACID NUCLEIC ACID is a polymer in which the monomer units are nucleotides NUCLEOTIDE is a three-subunit molecule in which a pentose sugar is bonded to both a phosphate group and a nitrogen-containing heterocyclic base PENTOSE SUGAR The sugar unit of a nucleotide is either the pentose ribose or the pentose 2- deoxyribose PHOSPATE Phosphate, the third component of a nucleotide, is derived from phosphoric acid (H3PO4). Under cellular pH conditions, the phosphoric acid loses two of its hydrogen atoms to give a hydrogen phosphate ion (HPO4 2) NITROGEN-CONTAINING HETEROCYCLIC They are divided into two groups BASES Pyrimidines and purines Pyrimidines (made of one 6 member ring): PyCUT Thymine Cytosine Uracil Purines (made of a 6 member ring, fused to a 5 member ring): PuGA Adenine Guanine NUCLEOTIDE FORMATION Base + sugar nucleoside Example Adenine + ribose = Adenosine Adenine + deoxyribose = Deoxyadenosine Base + sugar + phosphate(s) nucleotide Example Deoxyadenosine monophosphate (dAMP) Deoxyadenosine diphosphate (dADP) Deoxyadenosine triphosphate (dATP) Deoxyadenosine triphosphate Doxyadenosine diphosphate Deoxyadenosine monophosphate Deoxyadenosine Adenine NH2 Phosphoester bond N N H O O O N N –O P O P O P O CH2 5′ O O– O– O– 4′ H 1′ Base always H H H attached here Phosphate groups 3 2′ HO H Phosphates are attached here Deoxyribose Nucleotide nomenclature All of the names end in 5’- monophosphate, which signifies the presence of a phosphate group attached to the 5’ carbon atom of ribose or deoxyribose. Suffix Purine- osine Pyrimidine- idine Prefix deoxy- signifies deoxyribose sugar is DNA STRUCTURE Each strand of DNA is a polynucleotide chain DNA nucleotide has three main components 1. Deoxyribose (a pentose sugar) 2. Base (there are four different ones) 3. Phosphate Nucleotides are linked together by covalent bonds called phosphodiester linkage. P 5 1 Base 4 Suga r 3 2 P 5 1 Base 4 Suga r 3 2 Backbone Bases O 5′ CH3 N Thymine (T) – H N O O O P O CH2 5′ O – O 4′ 1′ H H H H 3′ 2′ NH2 H N N Phosphodiester H Adenine (A) linkage N O N O P O CH2 5′ O – O 4′ 1′ H H H H 3′ 2′ NH2 H H N Cytosine (C) O H N O O P O CH2 5′ O – O 4′ 1′ H H H H Guanine (G) 3′ 2′ O H N H N H O N N NH2 O P CH2 O Single 5′ O – nucleotide O 4′ 1′ H H Phosphate H H 3′ 2′ OH H Sugar (deoxyribose) 3′ Complimentary Base pairing The nitrogenous bases on one chain pair with the complementary bases on the other chain. Adenine pairs with thymine (A-T) guanine pairs with cytosine Hydrogen bonding The base pairs are held together by hydrogen bonds, which are weak but numerous, giving the DNA molecule its stability. A chemical bond consisting of a hydrogen atom between two electronegative atoms (e.g., oxygen or nitrogen) with one side be a covalent bond and the other being an ionic bond. 3 Hydrogen bonds 2 Hydrogen bonds Directionality of DNA structure It refers to the specific orientation of each strand within the DNA double helix, which is essential for proper base pairing, replication, and transcription. The two strands of DNA run in opposite directions, one from 5' to 3' and the other from 3' to 5'. This is known as antiparallel orientation. This antiparallel arrangement allows for the formation of DNA Double Helix There are two asymmetrical grooves on the outside of the helix: a)Major groove: The Minor groove Major groov major groove is wider e and deeper b)Minor groove:while the minor groove is narrower and shallower. SIGNIFICANCE 1. Protein Binding Sites: Proteins like transcription factors bind to specific DNA sequences in the major groove to regulate gene expression. They can either activate or repress the transcription of genes, controlling which proteins are produced in a cell. 2. DNA Replication and Repair: Enzymes involved in DNA replication and repair also bind to the grooves to recognize specific sequences and carry out their functions. SIGNIFICANCE 3. Structural Stability: The grooves contribute to the overall shape and stability of the DNA double helix. The helical twist and the orientation of base pairs in these grooves affect the shape and stability of the DNA double helix. 4. DNA Sequencing and Analysis: The grooves are also important for DNA sequencing and analysis techniques. The unique patterns of bases and their accessibility within the grooves help DNA CONFIGURATION IN THE DNA DNA is incredibly long, about 14 millimeters in a single human chromosome. To fit inside the tiny nucleus, it's compacted by a factor of 7,000, shrinking to 2 micrometers. DNA CONFIGURATION IN THE DNA The Basic Unit: The Nucleosome Nucleosome Formation: DNA wraps around a core of eight histone proteins (an octamer), forming a structure called a nucleosome. Imagine it like a bead on a string, with the DNA wrapped around the histone core (the spool) and the linker DNA connecting the DNA CONFIGURATION IN THE DNA Higher-Order Folding Solenoids: Nucleosomes are further folded and coiled into higher-order structures. One common structure is the solenoid, where nucleosomes are arranged in a helical structure, like a spring. Loops: Solenoids can then be further organized into loops, which are anchored to a protein scaffold within the nucleus. DNA CONFIGURATION IN THE DNA Compaction and Condensation Chromatin Fiber: The process of folding and looping creates a highly compact structure called a chromatin fiber. Chromosomes: During cell division, chromatin fibers are further condensed and packaged into structures called chromosomes. This is Significance Efficient Packaging: Supercoiling and looping work together to compact and organize the vast amount of DNA within the nucleus. This efficient packaging allows for the storage and regulation of a significant amount of genetic information in a limited space. Gene Regulation: Both supercoiling and looping are involved in the complex process of gene regulation. By influencing DNA accessibility and the interaction of proteins with DNA, these processes play a critical role in determining which genes are expressed and at what levels. DNA Replication Replication means the process of duplication. In molecular biology, DNA replication is the primary stage of inheritance. Central dogma explains how the DNA makes its own copies through DNA replication DNA REPLICATION IS SEMICONSERVATIVE Meselson and Stahl Experiment was an experimental proof for semiconservative DNA replication. DNA replication is a semiconservative process, meaning that each new DNA molecule consists of one original strand and one STEPS OF DNA REPLICATION Step 1 An enzyme called Helicase breaks the hydrogen bonds between the bases of the two antiparallel strands. The strands are initially split apart in areas that are rich in A-T base pairs forming a replication fork. DNA Gyrase (also called Topoisomerase) relieves tension that builds up as a result of unwinding. Single strand binding proteins (SSBs) help to stabilize the single stranded DNA. STEPS OF DNA REPLICATION Step 2 RNA polymerase (also known as RNA Primase) synthesizes short RNA nucleotides sequences that act as primers (starters). These essentially provide a starting point for DNA replication. STEPS OF DNA REPLICATION Step 3 DNA Polymerase III can now start synthesizing the new DNA strand using free DNA nucleotides. However, DNA polymerase can only read the original template (parent strand) in the 3’ → 5’ direction (making DNA 5’ → 3’). This is not a problem on the leading strand, because the DNA polymerase can simply continue to read along as the two parent stands continue to unzip. STEPS OF DNA REPLICATION Step 4 On the lagging strand DNA polymerase moves away from the replication fork. As the strands continue to unzip more DNA is exposed and new RNA primers must be added. As a result the lagging strand is synthesized in short bursts as DNA polymerase synthesizes DNA in- between each of the RNA primers. STEPS OF DNA REPLICATION Step 5 The newly synthesized lagging strand now consists of both RNA and DNA fragments. The DNA fragments are known as Okazaki fragments STEPS OF DNA REPLICATION Step 6 DNA Polymerase I now removes the RNA primers and replaces them with DNA STEPS OF DNA REPLICATION Step 7 DNA Ligase joins the DNA fragments of the lagging strand together to form one continuous length of DNA. Replication ends when the entire DNA molecule is copied, and telomerase maintains chromosome ends (in eukaryotes). STEPS OF DNA REPLICATION Step 8: TERMINATION DNA replication ends when either the replication forks meet or when they reach the end of a chromosome, which is marked by telomeres. These mechanisms ensure that the process is completed accurately and that the cell's genetic material is properly copied. ENZYME FUNCTION Helicases They break the hydrogen bonds between the nitrogenous bases in the DNA double helix, unwinding the two strands. This creates the replication fork, where the two strands are separated and ready to be copied. Single-Strand Prevents Parents strand from re annealing. This keeps the Binding Proteins strands apart so that DNA polymerase can access them. (SSBs) Primase Primase synthesizes a short RNA primer, which provides a starting point for DNA polymerase. DNA Polymerase It adds nucleotides to the new strand, building a complementary III copy of the template strand. DNA Polymerase I For primer removal and gap filling DNA Ligase It joins the Okazaki fragments on the lagging strand together, creating a continuous DNA strand. Topoisomerases As the DNA unwinds, it can create tension ahead of the replication fork. Topoisomerases relieve this tension by cutting and rejoining the DNA strands. Prokaryotic DNA Feature Eukaryotic DNA Replication Replication Origin of Multiple origins per chromosome Single origin per chromosome Replication Bidirectional (two forks per Replication Forks Bidirectional (two forks per origin) origin) Multiple DNA polymerases with Fewer DNA polymerases, DNA Polymerases specialized functions often with multiple functions Okazaki Longer (1000-2000 Shorter (100-200 nucleotides) Fragments nucleotides) Telomeres Present at chromosome ends Absent Chromatin DNA is not packaged with DNA is packaged with histone proteins Structure histone proteins Continuous throughout the Replication Timing S phase of the cell cycle cell cycle GENE ACTION: DNA TO PROTEIN REYNALDO O. TABANG JR., RMT 1. Structure of RNA 2. List the major types of RNA 3. Explain the importance of transcription factors 4. List the steps of transcription 5. List the steps of protein synthesis Ribonucleic acid (RNA) RNA is a polymer of ribonucleotides linked together by phosphodiester linkage. RNA is a single-stranded nucleic acid composed of nucleotides. It function as messenger(mRNA), adapter(tRNA), structural(rRNA) and in some cases as a catalytic molecule(Ribozyme). RNA STRUCTURE Each strand of RNA is a polynucleotide chain Nucleotides are linked together by covalent bonds called phosphodiester linkage. DNA nucleotide has three main components 1. Ribose (a pentose sugar) 2. A nitrogenous base: Adenine (A), Uracil (U), Cytosine (C), or Guanine (G). 3. Phosphate Major Types of RNA molecules Type of RNA Function molecule Messenger RNA (mRNA) Carries the genetic code from DNA to the ribosomes, where proteins are synthesized Transfer RNA (tRNA) Transport amino acids to the ribosomes during protein synthesis Ribosomal RNA (rRNA Associates with proteins to form ribosomes, which structurally support and catalyze protein synthesis DNA TRANSCRIPTION Is the process of making an RNA copy of a gene’s DNA sequence. This copy, called messenger RNA (mRNA), carries the gene’s protein information encoded in DNA. Selective transcription allows cells to regulate gene expression efficiently, supporting cell specialization, conserving resources, ensuring development and response to signals, and preventing the production of unnecessary or harmful proteins. Transcription factors Are proteins that bind to specific DNA sequences to regulate the transcription of genetic information from DNA to mRNA. Assist RNA polymerase in binding to the promoter region, crucial for starting transcription. They stabilize the transcription initiation complex, enabling the proper assembly of RNA polymerase and other essential proteins. Act as activators or repressors, regulating gene expression in response to signals like hormones, stress, or nutrients to ensure genes are expressed only when needed. Steps of Transcription It occurs in three main steps: Initiation Elongation termination. 1. Initiation Unwinding of DNA: The DNA double helix unwinds at the promoter region (a specific DNA sequence that signals where transcription should start). RNA Polymerase Binding: RNA polymerase, the enzyme responsible for transcription, binds to the promoter along with other transcription factors (proteins that help initiate transcription). In prokaryotes, RNA polymerase directly recognizes the promoter, while in eukaryotes, transcription factors help RNA polymerase locate and bind to the promoter. Formation of the Transcription Bubble:The DNA strands separate, forming an open complex (transcription bubble) where one of the strands serves as the template strand. R NA polymerase begins to synthesize RNA by reading the DNA template strand in the 3' to 5' direction. 2. Elongation RNA Synthesis: RNA polymerase moves along the DNA template strand, adding ribonucleotides (RNA nucleotides) that are complementary to the DNA template. The ribonucleotides (A, U, C, G) are added in the 5' to 3' direction. Adenine (A) pairs with uracil (U), cytosine (C) pairs with guanine (G), and vice versa. Formation of mRNA: The growing RNA strand, known as pre-mRNA in eukaryotes or mRNA in prokaryotes, elongates as RNA polymerase moves downstream along the DNA. Rewinding of DNA: As RNA polymerase moves forward, the DNA behind it rewinds back into its double-helix structure, while the transcription bubble 3. Termination Reaching the Terminator Sequence: RNA polymerase continues to elongate the RNA strand until it encounters a terminator sequence on the DNA. In prokaryotes, terminator sequences signal RNA polymerase to release the RNA transcript and detach from the DNA. In eukaryotes, termination is more complex and often involves the polyadenylation signal (a sequence that signals where the mRNA will be cleaved and polyadenylated). Release of the RNA Transcript: The newly synthesized RNA molecule (pre-mRNA in eukaryotes) is released from the DNA template. 4. Post- Transcriptional Modifications (Eukaryotes Only) Capping: A 5' cap is added to the beginning of the pre-mRNA for protection and to aid in translation. Splicing: Non-coding regions (introns) are removed, and coding regions (exons) are spliced together to form a mature mRNA molecule. Polyadenylation: A poly-A tail (a sequence of adenine Exons: Coding regions of a gene. Contain the sequences that are expressed and translated into proteins. Remain in the final mRNA after splicing. Introns: Non-coding regions of a gene. Do not code for proteins and are removed from the pre-mRNA during splicing. They are present in the DNA and pre-mRNA but are not part of the mature mRNA. Translation Translation converts the mRNA sequence into a polypeptide chain, which folds into a functional protein. Occurs in the cytoplasm of the cell at the ribosome (in both prokaryotes and eukaryotes). Key Components mRNA: Carries the genetic code from DNA. tRNA: Brings the appropriate amino acids to the ribosome; each tRNA has an anticodon that matches a specific codon on the mRNA. Ribosome: The molecular machine that reads the mRNA and catalyzes the formation of peptide bonds between amino acids. Codon A codon is a specifics sequence of nucleotides on an mRNA that corresponds to a specific amino acid or to a stop signal during protein translation. Start and Stop Codons Start Codon: AUG (codes for methionine), where translation begins. Stop Codons: UAA, UAG, and UGA, which do not code for any amino acids and signal the end of translation. Steps of translation 1. Initiation The small ribosomal subunit binds to the mRNA at the start codon (AUG) which codes for the amino acid methionine. A tRNA molecule carrying methionine (the initiator tRNA) binds to this start codon. The large ribosomal subunit then joins the small subunit to form the complete ribosome. The ribosome has three sites: E (exit), P (peptidyl), and A (aminoacyl). The initiator tRNA occupies the P site of the ribosome. Steps of translation 2. Elongation The next codon on the mRNA sequence is read by the ribosome, and the appropriate tRNA carrying the corresponding amino acid enters the A site. A peptide bond forms between the amino acid in the P site and the new amino acid in the A site, linking them together. The ribosome moves along the mRNA (a process called translocation): The tRNA in the P site shifts to the E site and exits the ribosome. The tRNA that was in the A site now moves to the P site, making room for a new tRNA to enter the A site. This process repeats, adding amino acids one by one and elongating the polypeptide chain. Steps of translation 3. Termination The ribosome reaches a stop codon on the mRNA (e.g., UAA, UAG, or UGA). These codons do not code for any amino acids and signal the end of translation. A release factor binds to the stop codon, causing the ribosome to release the newly formed polypeptide chain. The ribosomal subunits disassemble, and the mRNA is released. Example Step 1: DNA Sequence (Template Strand) 3' - TAC GGA TCA TCG TTT GAA - 5’ Step 2: Complementary DNA Sequence (Coding Strand) 5' - ATG CCT AGT AGC AAA CTT - 3' Step 3: Transcription to mRNA During transcription, the template strand of DNA is used to synthesize a complementary mRNA sequence. Remember, RNA uses Uracil (U) instead of Thymine (T). DNA Template Strand: 3' - TAC GGA TCA TCG TTT GAA - 5' mRNA Transcript: 5' - AUG CCU AGU AGC AAA CUU - 3' Example Step 4: Translation to Amino Acids The mRNA sequence is read in codons (groups of three nucleotides) during translation. Each codon corresponds to an amino acid: 1.AUG - Methionine (Start Codon) 2.CCU - Proline 3.AGU - Serine 4.AGC - Serine 5.AAA - Lysine 6.CUU - Leucine Methionine - Proline - Serine - Serine - Lysine - Leucine