Bioenergetics and Thermodynamics Lecture 6 PDF
Document Details
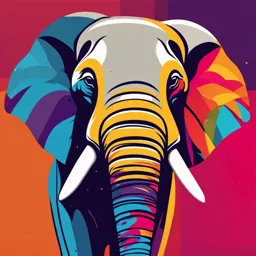
Uploaded by AmbitiousObsidian1851
Tags
Summary
This document provides a lecture on bioenergetics and thermodynamics, covering basic definitions and principles. The lecture touches on different types of energy systems and the laws of thermodynamics, relating to energy transformation and equilibrium. It also explores the concept of entropy.
Full Transcript
# Bioenergetics and Thermodynamics ## Learning Outcomes * Explain thermodynamics definition. * Understand different systems for energy transferring. * Explain first law of thermodynamics. * Explain second law of thermodynamics. ## Introduction * Bioenergetics is the quantitative study of the en...
# Bioenergetics and Thermodynamics ## Learning Outcomes * Explain thermodynamics definition. * Understand different systems for energy transferring. * Explain first law of thermodynamics. * Explain second law of thermodynamics. ## Introduction * Bioenergetics is the quantitative study of the energy transductions that occur in living cells as well as the nature and the function of the chemical processes underlying these transductions. * Biological energy transductions obey the same physical laws that govern all other natural processes. * Therefore, we first review the laws of thermodynamics and the quantitative relationships among free energy, enthalpy, and entropy. * Thermodynamics is the branch of physics concerned with heat and temperature and their relation to energy and work. Therefore, thermodynamics can be used to describe how systems respond to changes in their surroundings/environment. * Biochemical thermodynamics (Bioenergetics) is the field of biochemistry concerned with the transformation and use of energy by living cells. ## What is a system? It is the collection of matter that is undergoing a particular chemical or physical process; it may be an organism, a cell, or two reacting compounds (a matter within a defined region). * The biochemical reactions are associated with the liberation of energy as the reacting system moves from a higher to a lower energy level, and the energy liberated in the form of heat. * In non-biological systems, heat energy may be transformed into mechanical or electrical energy. * In biological systems (since it is isothermic) the heat energy cannot be used to derive the vital process including synthesis, active transport, muscular contraction, oxidative phosphorylation...etc. obtain energy by chemical linkage (or coupling) to oxidation reactions. ## Thermodynamic Systems Energy transfer is studied in three types of systems: * **Open systems**. Open systems can exchange both matter and energy with an outside system. They are portions of larger systems and in intimate contact with the larger system. Your body is an open system. * **Closed systems**. Closed systems exchange energy but not matter with an outside system. Though they are typically portions of larger systems, they are not in complete contact. The Earth is essentially a closed system; It obtains lots of energy from the Sun, but the exchange of matter with the outside is almost zero. * **Isolated systems**. Isolated systems can exchange neither energy nor matter with an outside system. While they may be portions of larger systems, they do not communicate with the outside in any way. The physical universe is an isolated system; a closed thermos bottle is essentially an isolated system (though its insulation is not perfect). ## Thermodynamics Principles Many quantitative observations made on the interconversion of various forms of energy have led scientists to the formulation of two fundamental laws of thermodynamics, the first and second. These laws help us understand: * The direction of a reaction, whether from left to right or vice versa, * The accomplishment of work, whether useful or not, and * Whether the energy for driving a reaction must be delivered from an external source. ## First Law of Thermodynamics * The first law of thermodynamics is the principle of the conservation of energy. It states that in any chemical or physical change, the total energy of the universe remains constant. This includes both the system and its surroundings, although the form of energy may change. * This means that energy cannot be created or destroyed. To date, there is no known exception to this law. Therefore, whenever energy is used to do work or it is converted from one form to another, the total amount of energy is unchanged. * The mathematical expression of the first law is: $$ \Delta{E} = E_B - E_A = Q - W $$ where, ΔΕ = change in internal energy, ΔΕ A = energy of a system at the start of a process, ∆Ев= energy of a system at the end of a process, Q = heat absorbed by the system, W = work done by the system. * A noteworthy point about this equation is that the change in energy of a system depends only on the initial and the final stages and not on the path of transformation. * The first law of thermodynamics cannot be used to predict whether a reaction can occur spontaneously, although ΔΕ is positive. In such cases, the system absorbs heat from its surroundings so that the sum of the energies of the system and its surroundings remains constant. It is evident that a function other than ΔΕ is required. ## Second Law of Thermodynamics The universe tends toward maximum disorder (the entropy of the universe always increases). * The entropy of a system increases when it becomes more disordered. Entropy becomes maximum in a system as it approaches equilibrium. When equilibrium is attained, no further change can occur spontaneously unless additional energy is supplied from outside the system. * The second law of thermodynamics, which can be stated in several forms, says that the universe always tends toward more and more disorder. In cell natural processes, the entropy of the universe increases. * The concept of entropy gives us a more quantitative way to describe the tendency for energy to flow in a particular direction. * The entropy change for a system is defined mathematically as the flow of energy divided by the temperature, or, $$ \Delta{S} \ge [Q/T] $$ where S is the change in entropy, Q is the heat flow into or out of a system, and T is the absolute temperature in degrees Kelvin (K). * In all spontaneous processes, a system in which it releases free energy and it moves to a lower, more thermodynamically stable energy state, the total entropy increases and the process is irreversible. * Examples of spontaneous processes (entropy increases): a smell diffusing in a room, ice melting in warm water, salt dissolving in water, and iron rusting. ## In Chemical Reactions * Solids generally have a low entropy because their particles are very organized. * Liquids have greater entropy than solid. * Gases have the highest entropy of all. The entropy of a system changes during any chemical process. * Therefore, the total entropy in the universe must be equal to the entropy of the system plus the surroundings. * Any change in the universal entropy must be due to a change in entropy of the system, surroundings, or both. I.e. $$ \Delta{S (universe)} = \Delta{S (system)} + \Delta{S (surroundings)} $$ The natural tendency of the universe is to progress toward maximum entropy; so, any chemical reaction with a positive entropy change is favorable and more likely to be spontaneous than a chemical reaction that decreases entropy. * For example, consider this chemical reaction: $$ CaCO_3(s) \rightarrow CaO(s) + CO_2(g) $$ Because a gas is produced, we can expect that this reaction has a positive entropy change. * Now consider this reaction: $$ NH_3(g) + HCl(g) \rightarrow NH_4Cl(s) $$ In this reaction, a solid is formed from two gasses. The system becomes more organized, so we can expect that this reaction has a negative entropy change.