Kinesiology Fundamentals of Motion Description PDF
Document Details
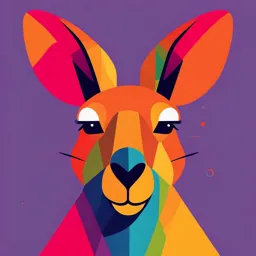
Uploaded by DeservingPoplar
University of Maryland
1971
David L. Kelley
Tags
Summary
This is a textbook on kinesiology, focusing on the fundamentals of motion description and electromotive characteristics of muscles. The book explores the electrical activity of muscles through electromyography, explaining the underlying concepts in human movement and is suitable for undergraduate studies.
Full Transcript
# KINESIOLOGY ## Fundamentals of Motion Description **David L. Kelley** **University of Maryland** **Prentice-Hall, Inc. Englewood Cliffs, New Jersey** **Copyright 1971 by Prentice-Hall, Inc. Englewood Cliffs, New Jersey.** **All rights reserved. No part of this book may be reproduced in any f...
# KINESIOLOGY ## Fundamentals of Motion Description **David L. Kelley** **University of Maryland** **Prentice-Hall, Inc. Englewood Cliffs, New Jersey** **Copyright 1971 by Prentice-Hall, Inc. Englewood Cliffs, New Jersey.** **All rights reserved. No part of this book may be reproduced in any form or by any means without permission in writing from the publisher.** **Printed in the United States of America** **Library of Congress Catalog Card Number: 79-144098** **Epigraph: From Thomas Mann, *The Magic Mountain*, trans. H. T. Lowe-Porter (© Copyright 1952 by Alfred A. Knopf, Inc.).** **Prentice-Hall International, Inc., London** **Prentice-Hall of Australia, Pty., Ltd., Sydney** **Prentice-Hall of Canada Ltd, Toronto** **Prentice-Hall of India Private Limited, New Delhi** **Prentice-Hall of Japan, Inc., Tokyo** ## 12 The Electromotive Characteristics of Muscle The contraction of muscle is preceded by the passage of neural impulses across the myoneural junctions of the muscles involved. When these impulses reach the muscle fiber membrane, a wave of electrical activity passes the length of the muscle fiber. This is known as the excitation wave. Since the neural impulses and the excitation wave are electrical in nature, they may be recorded by specialized equipment. In the case of the excita-tion wave exhibited in muscle, the recording apparatus is known as the electromyograph and the recording is the electromyogram. While the knowledge of this electrical phenomenon has been in existence for many years, it was considered little more than a curiosity until the electronic developments of recent times. The availability of equipment sensitive enough to monitor the minute electrical currents typifying the excitation process has resulted in the collection of a unified body of knowledge and the emergence of the field of electromyography. Much of the value of electromyography has been in the area of medical science, particularly as a diagnostic tool for neuromuscular pathology. For that purpose the work has been directed toward the study of the motor units, singularly or in small groups. While electromyography continues to be of great medical value, it has also attracted the interest of exercise physiologists and kinesiologists. These investigators have been more interested in the study of the actions of whole muscles or groups of muscles than in individual motor unit activity. The use of electromyo-graphy in the analysis of human motion has become of greater interest in recent years. While it is the purpose of this chapter to review the electrical characteristics of muscle and muscular contraction and explore its uses in motion analysis, it is, by no means, complete. Therefore, the interested reader is directed to the more detailed information afforded by the sources included at the end of the chapter. ## THE ELECTRICAL ACTIVITY OF MUSCULAR CONTRACTION Underlying the excitation wave phenomenon is the concept that the resting muscle fiber wall (sarcolemma) acts as a semipermeable membrane, with a high concentration of sodium ions on the outside and a high concentration of potassium ions on the inside of the membrane. This arrangement results in an electrical differential which is known as the membrane potential, with the outside of the membrane being charged positively and the inside charged negatively. When a nerve impulse travels along the axon to the motor unit and is transmitted by means of the myoneural junction to the muscle fiber, the permeability of the sarcolemma is changed. The change in membrane permeability results in sodium and potassium ions exchanging places. As the excitation wave passes along the muscle fiber, the membrane then reverts to its resting, polarized state, a situation which causes the sodium and potassium ions to return to their original positions. The electrical activity accompanying the exchange of ions is known as the action potential, and it is likely that it traverses the fiber's length before appreciable tension develops. In electromyography, it is these action potentials which are monitored and recorded. ## RECORDING OF MUSCLE ACTION POTENTIALS Electromyography is concerned with the measurement and analysis of muscle action potentials. Numerous techniques for recording and various methods of analyzing the data have been developed over the years. The person interested in using electromyography must determine exactly what his objectives are and then what instrumentation would best provide valid data. Whatever his choice, some type of electrodes must be attached to the subject to act as the first element between the bioelectric source and the recording device. The electrodes are then connected by wire or a telemetry device to a specialized piece of equipment (electromyograph) which receives and then records the action potentials as electromyograms. The electrode type used depends very much on the objective of the measurement. When the objective is to examine and measure the electrical activity of motor units individually or in small groups, then the choice would suitably be an electrode which is inserted directly into the muscle, either the needle or wire type. When measurements of the whole muscle or groups of muscles are to be made, surface electrodes may often be of value. Since surface electrodes monitor the electrical activity of the muscle fibers underlying them, information concerning specific frequencies, single motor unit activity, and action potential shape cannot be obtained but should be monitored by intramuscular electrodes. It has been reported that electrical activity measured from surface electrodes and intramuscular electrodes tends to be highly correlated with respect to quantitative features. The use of surface electrodes requires very careful preparation of the electrode site. The electrical energy must pass through the tissue located between the muscle and the electrode. These tissues offer a resistance to the flow of an alternating current known as impedance. The tissues lying below the skin have been shown to have relatively low impedance, while the skin itself has a high level of impedance. The preparation of the electrode site involves removing excessive hair from the surface, abrasion of the skin with a fine grade of sandpaper to remove some of the epidermal cells, and the application of an alcohol solution to the area to remove surface oils. When these steps have been completed, an electrolyte paste or jelly is rubbed into the prepared area. The electrolyte is then applied to the electrode, which is then secured to the prepared skin area. A measure of the impedance should then be taken. The maximal level of impedance considered acceptable depends in part on the objective of the study. Recordings of contractions of great magnitude will be influenced less by high impedance levels than will recordings of resting muscles. Levels ranging from 10,000 to 30,000 ohms (Ω) have been suggested. It is obvious that one should strive for low impedance conditions. The electrode configuration is usually one of two types: (1) unipolar and (2) bipolar. The former configuration consists of one electrode positioned over the muscle to be monitored and a second electrode positioned over an area which will be electrically inactive, possibly over an area where bone resides just under the skin. The bipolar configuration, on the other hand, consists of two "active" electrodes positioned close together over the muscle and a third, "indifferent" electrode placed over an inactive site. The electrodes used to monitor the action potentials sample only the electrical activity in the muscle mass in close proximity to the point of electrode application. While the unipolar recording is felt to be more easily analyzed than the bipolar regarding the waveform produced, when the activity of the whole muscle is being monitored, waveform is usually of little importance. A more important factor in the total electrical activity recorded is that of its tendency to spread from one muscle to another. The bipolar configuration samples only the area of the muscle lying under and between the two active electrodes. The size of the area sampled may be increased or decreased by altering the space between these electrodes. The bipolar configuration allows for more control over the possibility of electrical activity from adjacent muscles being monitored and recorded. On the other hand, the unipolar configuration monitors all of the electrical activity from the muscle mass around and under it, making it more susceptible to the electrical conditions of surrounding muscles. The electrical activity monitored by the electrodes can be recorded by several different instruments. The most common methods are photographing the wave produced on an oscilloscope and adapting the action potentials to drive a pen-writer which then produces an ink or heat tracing on moving paper. Whatever method is used to record the action potentials, the mode of operation must be determined by the investigator. A common mode of recording when one is interested in the activity of individual motor units, small groups of motor units, or the pattern of excitation is known as direct recording (Fig. 12-2a). In this mode, the electrical activity of the muscle fibers is recorded as it is monitored. From the baseline, the recording pen may move both positively (upward) and negatively (downward). This type of recording can be valuable when studying the very small magnitudes of activity produced during relaxation. When the amount of activity increases, however, the individual waveforms of motor units are no longer visible. If one is interested in the concurrent actions of several muscles during a movement, the direct recording mode can be used to help determine the relationships of these muscles to each other. The second mode of recording is that known as integration (Fig. 12-2b). The integrated recording is the result of electronic treatment of the electrical signals so that the frequency, duration, and amplitude of the current are averaged. The tracing thus produced is indicative of the amount of electrical activity produced during a particular activity or contraction. While the direct reading is more adaptable to subjective evaluations, the integrated recording is more useful in quantitative terms where statistical treatments are desired. Another method of recording the muscle action potentials is similar to the integrated method just discussed. However, after the integration procedure has been accomplished, the signal is treated by a voltage-to-frequency converter, the pulsed frequency output of which is proportional to the quantity of electrical energy monitored. The frequency output is then directed into an electronic counter which produces a digital figure representing the magnitude of the action potentials. These readings may be recorded over various time intervals and may be submitted to statistical treatment. ## ANALYSIS OF THE ELECTROMYOGRAM The recording of the muscle action potentials by any of the popular methods leaves the investigator faced with the problem of analyzing and interpreting the results. A review of the electromyographic literature indicates that there is no universally approved method of scoring and analysis. As would be expected, some investigators prefer to be rather subjective, while others lean toward a more objective approach. Regardless of the measurement approach taken, both qualitative and quantitative elements are available in the data for interpretation. Again, the choice of a measurement technique depends on the objectives of the investigation and it often boils down to a choice between methods of scoring. One subjective method of scoring electromyographic data is in common use. It depends on subjective judgments which are based upon careful training and experience. It is a method of visual inspection of the electromyogram which employs a system of classification that establishes categories of electrical activity by assigning a series of symbols (-, 0, +, +++) indicating relative magnitudes. Decisions regarding magnitudes involve amplitude and frequency primarily. An important value of this method is that it generally limits the investigator to the realm (a very productive realm to be sure) of relationships rather than strict quantification of numerical data. A second and more subjective method of analysis is performed by feeding the amplified action potentials into a loudspeaker. The result gives an audio impression of the quality and quantity of the excitation process which is quite dramatic. It has proved its value in clinical diagnostic practice as well as its use as a source of auditory feedback in studies involving muscle relaxation. Many different scoring methods have been devised in attempting to make electromyogram analysis more objective. All rely on some form of quantitative appraisal which may be subjected to statistical treatment for interpretive assistance. Some investigators have counted the frequency of the spikes in interference patterns. Studies of muscles involved in high levels of tension development have often used some form of amplitude measurement. The amplitude has been averaged over a predetermined period of time in some cases, while in others the highest amplitude at a given time during a movement has been used. Others have employed combinations of frequency and amplitude appraisal from interference patterns. And, of course, the method of integration described earlier as averaging frequency, duration, and amplitude is in common use. Attempts have been made to compare the results of spike counting with those of integration. For example, Bergström compared the number of spikes counted from a direct motor unit recording with the results of an integrated recording. He found a linear relationship up to a spike frequency of about 500/sec.3 He concluded that an estimation of the electrical activity of the whole muscle can be made by counting motor unit spikes. It should be pointed out that this study involved activity of small muscles of the hand and may not be entirely representative of the activity of larger muscles containing many more fibers under the control of fewer motor units per active fiber. One basic problem involved in using the frequency or amplitude is that there are notable variations in the electromyogram during the period of contraction. Another lies in the nature of the direct reading taken from a maximal muscular contraction. The spike potentials usually represent the combination of the excitation phases from many motor units firing at their independent rates. The value of spike count and amplitude measurement probably lies in the study of small muscle activity. The most common method of obtaining a measure utilizing both frequency and amplitude is by means of integration. This technique has been used by many investigators who have been interested in data which could be quantified with accuracy and submitted to statistical analysis. The typical procedure has been to measure the area under the curve drawn by the pen-writer, usually by means of a planimeter. Figure 12-3 illustrates an integrated electromyogram in which the area under the curve for a 1-sec duration has been shaded. The planimeter is made to trace the boldface line which acts as the perimeter of the shaded area. It then gives an indirect measure of the area, which is usually specified in emg units. Since the area measurement procedure remains the same, emg-unit values obtained from other electromyograms under similar conditions may be compared. In summary, the best method of scoring is probably dependent on the objective of the study in question. If one is interested in the functioning of motor units, individual muscles, or the patterns of the action in a group of muscles, then the more subjective techniques are probably best. These types of studies are described later under "Qualitative Analyses." On the other hand, if one is interested in the relationships of force and electrical activity or joint angle and electrical activity, then the more objective, integrative techniques are probably more desirable. These will be discussed under "Quantitative Analyses" in subsequent pages. ## USES OF ELECTROMYOGRAPHY IN THE ANALYSIS OF MUSCULAR ACTIVITY Electromyography (EMG) has been extremely important in the analysis of muscle action during movement. The analysis of the electrical activity during a skillful movement allows one to better understand how those muscles actually function. However, it is easy for the beginning student to assume that a particular movement occurs because of the action of the muscles which have been assigned that function in anatomy texts. The movement of elbow flexion, for example, is accompanied by action of the elbow flexors. To most, it should be obvious that forces other than muscular contraction can cause movement. Nevertheless, to some the assignment of elbow flexion to the biceps brachii indicates that whenever that movement occurs, the biceps brachii must be active. Actually, that muscle is often active during elbow extension. Because of space limitations, the Muscle Charts which accompany Chapters 14 through 20 do not include many actions such as the eccentric control of elbow extension by the elbow flexors. The mastery of these concomitant actions is left almost entirely to the student, whose inquiring nature will almost certainly impel him to accomplish the task. ## Quantitative Analyses The use of EMG to study muscle actions is not limited to determining when and if muscles act. When electromyograms are prepared for quantitative analysis, inferences may be drawn as to how and why muscles respond with varying magnitudes of activity. ## Emg-Force Relationships An assumption which seems to prevail in much of the literature is that there is a positive relationship between the force of muscular contraction and the electrical activity monitored from the contracting muscles. While this has been shown to be the case under many varied conditions, care should be taken in acceptance of this assumption under all conditions. The electrical activity monitored from active muscles is directly related to the number of muscle fibers being stimulated. Provided that the muscle fiber contracts when it is stimulated, then it would follow that a greater number of active muscle fibers would produce a greater amount of force. Research into the change seen in the frequency and the amplitude of the action potentials during increased work loads indicates that these two factors do not respond identically. Frequency tends to increase linearly with force of contraction until some limiting point is reached, where it then tends to stabilize. On the other hand, amplitude seems to continue to increase throughout an increasingly heavy action. Integrated electromyograms include both of these elements and therefore show electrical activity to continue to increase when the contractions are isometric in nature. Figure 12-4 graphs the typical emg activity and force production of two elbow flexors during 2 min of maximal, isometric contraction at a flexion angle of 115 deg. As the muscles lose their ability to exert applicable force over the sustained contraction period, emg activity follows the same trend, with that of the biceps brachii being more accelerated. While there is general agreement as to the relationship between emg activity and muscular force in isometric contractions, there is some disagreement regarding the relationship during isotonic contractions. Although the literature presents conflicting evidence, in studies where the rate of contractions and load intensity were controlled, the relationship was shown to be similar to that seen in isometric contractions. ## Emg-Muscle Length Relationships Muscles which function around a given joint can be seen to act more forcefully at one joint angle than another. The elbow flexors, for example, have been shown to produce their greatest amounts of force at an elbow angle approaching 120 deg. Physiologically, muscle fibers have been found to be most efficient during isometric contractions when operating at a length slightly longer than their resting length. It is evident, however, that muscle length is not the only factor influencing the force exerted by the limb in question. Variations in joint angles tend to influence the length of the muscles involved as well as their tension angles. The important factor would seem to be the composite, physiological-mechanical advantage resulting from a combination of these two factors, which are directly related to the joint angle. If the external force produced by a muscle were held constant while its physiological-mechanical advantage was varied, it would be seen that the number of active muscle fibers would also vary. More fibers would be required to perform the same task when the physiological-mechanical advantage was decreased, while less would be required when it was increased. As explained earlier, action potentials result from the excitation of muscle fibers. If a greater number of muscle fibers is stimulated or if the rate of stimulation is increased, a greater magnitude of electrical activity ensues. It follows then that a muscle operating from a favorable position would use fewer muscle fibers and therefore produce less electrical activity than under more unfavorable position conditions. Although the graphs in Fig. 12-5 do not represent activity under constant external force application conditions, they were obtained under continuing conditions of maximal isometric exertion. It can be seen that emg activity was greatest for the biceps brachii in its shortest position (45-deg joint position) and remained that way throughout almost all of the contraction period. At 115 deg, the muscle is at its intermediate length and exhibits intermediate emg activity. At its greatest length (170-deg joint angle), emg activity was lowest throughout. Since these contractions were all maximal as to effort, we must assume that the rate of stimulation was similar under the three joint angle conditions. Therefore, it would appear that the differences were due to the number of active fibers involved. This suggests that of the two factors involved in the physiological-mechanical advantage, muscle length is the more important contributor to favorable conditions for efficient muscular action, as was pointed out in Chapter 8. Numerous studies support the relationship given above concerning muscle length and electrical activity. Most of the data reported have been drawn from flexor muscles. A few authors who have examined extensor muscles have produced conflicting evidence. These studies show greater electrical activity in lengthened extensor muscles as opposed to their shortened states. Considering the conflicting nature of the data reported, it would appear advisable to consider the physiological-mechanical advantage of the muscles in question rather than simply their lengths. → Is it likely that a shortened flexor muscle is disadvantaged to a greater degree than a shortened extensor muscle? More work needs to be undertaken in this area. Worthy of careful attention is the approach taken by de Vries and others involving quantitative analysis. The technique is that involving voltage measurement which was briefly described on p. 165. New insights into muscle tonus, muscular relaxation, and emg-force relationships, particularly with very low potential levels, have been among the notable outcomes of that effort. The reader should begin by referring to the text identified in footnote 4. ## Emg-Muscular Fatigue Relationships One of the factors which is known to influence the electrical activity of muscle is muscular fatigue. As used here, fatigue refers to the condition which develops within the neuromuscular system which prevents the maintenance of initial levels of contractive force. The discussion of fatigue in general is beyond the scope of this text, but the effects that fatigue may have upon the electromyogram are important. Numerous investigators have used electromyography as a tool to study the problem of fatigue, with the results being somewhat at odds. Some results indicate that as fatigue develops, the recorded electrical activity increases. Others show that the decrease seen in the force exerted is accompanied by a similar decrease in the action potentials (refer again to Figs. 12-4 and 12-5). Under these maximal-effort, isometric conditions, it appears that those muscles which exhibit the greatest electrical activity early in the fatiguing bout show the most rapid reduction of that activity as fatigue sets in. The same sort of relationship seems to apply for the same muscle under varying length conditions (Fig. 12-5). If we compare different muscles or the same muscle under different conditions, fatigue acts as a great equalizer for electrical activity. A closer investigation of the fatigue studies involving EMG suggests that changes in the electromyogram owing to fatigue may be dependent on the type of conditions employed to develop the fatigue. When submaximal contractions were used to bring about fatigue, the electrical activity was seen to increase. However, when maximal efforts were used, the resulting pattern of activity was one of decrease. It should be evident that care must be taken in comparing emg-force relationships of muscles undergoing fatiguing activity with these same relationships of rested muscles. ## Concentric Versus Eccentric Contractions A muscle can develop tension in conjunction with shortening its length as well as increasing its length. Evidence from a number of different approaches has shown that greater tension can be produced by muscles when contracting eccentrically than when contracting concentrically. Assmussen has indicated that only about one third as many muscle fibers are required to perform a task eccentrically than are needed to perform the reversed task concentrically. In the latter, the contraction produces the movement, while in the former, the contraction controls the movement under extrinsic motivation. It is important to avoid any urge to refer to these two movement situations as constituting the same task. It must be assumed that the eccentric operation, because of its considerably lower active-fiber demands, would result in a lower electrical activity output even though the force exerted might be the same. Figure 12-6 illustrates direct and integrated emg patterns for such a concentric-eccentric contraction cycle under conditions which were controlled with respect to contraction durations and movement ranges. It is apparent that the concentric operation elicits the greater magnitudes of electrical activity. Thus it can be seen that in relating the electrical activity to the force produced, care must be taken to consider the nature of the contraction involved. ## Qualitative Analyses The analysis of muscular activity in qualitative terms is extremely important in the discussion of skilled movement. Evaluation of the electromyogram qualitatively allows one to look at the pattern of the excitation of various muscles. The amount of electrical activity is important as an indication of the degree of activity rather than as an indication of the force produced by the muscle. By monitoring the electrical activity of a group of muscles, one can determine when each muscle was active, when it was most and least active, whether or not the muscles were active in cyclical sequences, and many other factors which may be of interest regarding a specific problem under investigation. ## Testing Traditional and Theoretical Concepts Logically inferred muscle actions, which for years have awaited confirmation as the result of careful research work, have been substantiated in some cases and refuted in others on the basis of electromyographic evidence. An example of confirmation can be found in the shunt and spurt theory of MacConaill, which was introduced in Chapter 11.6 Mathematical analysis suggested that a muscle's position across its joint should indicate to some degree the conditions under which it will perform. Thus a shunt muscle should act in rapid movements to provide stabilizing support. The brachioradialis has been cited as an example of a shunt muscle. Muscles which act to produce the predominant rotatory forces because of their positions across the joint have been described as spurt muscles. At the elbow joint, the biceps brachii and brachialis fit this description. Electromyographic evidence shows the brachioradialis to be relatively inactive during slow elbow flexions and quite active during rapid flexions. The biceps brachii and brachialis tend to be active under most conditions of elbow flexion to offer their changing rotatory components to move the segment. An example of refuting evidence is found in the very old function applied to the brachioradialis, namely, supination of the forearm. This supposed function was so firmly set in the anatomical literature that the muscle was named the supinator longus. Electromyographic study has shown that this muscle is not a true supinator and is active in supination only when it occurs with the elbow extended, under resistance. Even at that, its supinating assistance is suspect. Enough examples exist, such as that just noted, to cause one to be cautious about assigning function to muscles without careful consideration of such factors as speed of contraction and resistance to the movement under question. ## Ballistic and Sustained Movements Electromyography has been employed by Hubbard in the study of muscular contractions to identify differences between the so-called ballistic and sustained types" (refer to Chapter 10 and its discussion of angular impulse and momentum). The ballistic contraction is said to produce a short impulse which sets the segment moving and continuing as a result of its momentum. A sustained contraction is one in which the muscle produces useful tension throughout the movement. The electrical activity of the biceps brachii and triceps brachii was monitored during rapid elbow flexions and extensions in the transverse plane while the shoulder was abducted, 90 deg. It was shown that the elbow flexors were responsible for accelerating the forearm through the first few degrees of flexion, after which the segment tended to move at a uniform speed for a time until it was compelled to decelerate. The uniform speed period was called the momentum phase. During this phase, biceps brachii shortening was said to "take up slack" within itself rather than applying motivating force to the bone as was indicated by reduced electrical activity. It was concluded that during the momentum phase, the muscle was contracting but was not actually causing the continued motion. It was simply keeping up with the rapidly moving segment to which it was attached.. In a sustained movement, the electromyogram would indicate more uniform activity of the agonists throughout the action. It seems likely that sustained contractions occur in slow, refined activities where a momentum phase is never clearly evident. The literature tends to support the ballistic theory as the basis for rapid or violent movements but gives no clear answer for the action during slow movements. Consequently, care must be taken not to assume that monitored electrical activity from a muscle during a movement means that it is undergoing a sustained contraction. It may simply be keeping in step with the movement. ## Emg Patterns of Muscle Groups It was stated previously that the pattern of electrical activity of muscles during a specific movement is of interest to the kinesiologist because it gives him information about the way individual muscles function within a group. It is one thing to say that a muscle is merely involved in a particular activity, but quite another to specify precisely its correct function. Therefore, the kinesiologist finds not only general activities useful in electromyographic investigations but sports skills in particular because they reflect movement behavior which runs the gamut of abilities from the halting, beginning attempts of the novice to the beauty and precision of the professional athlete. ## REFERENCES Adrian, E. D. "Interpretation of the Electromyogram." Lancet 5311 (1925): 1229, 5312 (1925): 1283. Adrian, E. D., and Bronk, D. W. "The Discharge of Impulses in Motor Nerve Fibers." Journal of Physiology 67 (1929): 119. Allen, C. E. L. "Muscle action Potentials Used in the Study of Dynamic Anatomy." British Journal of Physical Medicine 11 (1948): 66. Assmussen, E. "Muscular Performance." In Muscle as a Tissue, edited by K. Rodahl and S. M. Horvath. New York: McGraw-Hill Book Company, 1960. Basmajian, J. V. "Control and Training of Individual Motor Units." Science 141 (1963): 440. Muscles Alive: Their Function Revealed by Electromyography. 2d ed. Baltimore: The Williams & Wilkins Company, 1967. ""Spurt' and 'Shunt' Muscles: an Electromyographic Confirmation." Journal of Anatomy 93 (1959): 551. Battye, C. K., and Joseph, J. "An Investigation of Telemetering of the Activity of Some Muscles in Walking." Medical and Biological Engineering 4 (1966): 125. Bergstrom, R. M. "The Relation Between the Number of Impulses and the Integrated Electric Activity in Electromyograms." Acta Physiologica Scandinavica 45 Suppl. (1959): 97. Bierman, W., and Yashmon, L. J. "Electromyography in Kinesiologic Evaluations." Archives of Physical Medicine 29 (1948): 206. Bigland, B., and Lippold, O. C. J. "The Relation Between Force, Velocity, and Integrated Electrical Activity in Human Muscles." Journal of Physiology 123 (1945a): 214. Broer, Marion R., and Houtz, Sara Jane. Patterns of Muscular Activity in Selected Sport Skills: An Electromyographic Study. Springfield, Ill.: Charles C. Thomas, Publisher, 1967. Close, J. Robert. Motor Function in the Lower Extremity. Springfield, Ill.: Charles C. Thomas, Publisher, 1964. Close, J. R.; Nickel, E. D.; and Todd, F. N. "Motor-Unit Action-Potential Counts. Their Significance in Isometric and Isotonic Contractions." Journal of Bone and Joint Surgery 42-A (1960): 1207. Csapo, A. "Studies on Excitation-Contraction Coupling." Annals of the New York Academy of Sciences 81 (1959): 453. Davis, John F. "Manual of Surface Electromyography." WADC Technical Report 59-184. Wright-Patterson Air Force Base, Ohio: Wright Air Development Center, December 1959. Denslow, J. S., and Graham-Service, D. "The Spread of Muscle Action Potentials from Active to Inactive Areas." Federation Proceedings 7 (1948): 27. deVries, Herbert A. Physiology of Exercise. Dubuque, Iowa: Wm. C. Brown Company, Publishers, 1966. "Quantitative Electromyographic Investigation of the Spasm Theory of Muscle Pain." American Journal of Physical Medicine 45 (1966): 119. Eccles, J. C., and Sherrington, C. S. "Numbers and Contraction Values of Individual Motor-Units Examined in Some Muscles of the Limb." Proceedings of the Royal Society of Medicine 106 (1950): 326. Edwards, R. G., and Lippold, O. C. J. "The Relation Between Force and Integrated Electrical Activity in Fatigued Muscle." Journal of Physiology 132 (1956): 677. Flint, M. M., and Gudgell, J. "Electromyographic Study of Abdominal Muscular Activity During Exercise." Research Quarterly 36 (1965): 29. Floyd, W. F., and Silver, P. H. S. "The Function of the Erectores Spinae Muscles in Certain Movements and Postures in Man." Journal of Physiology 129 (1955): 184. Gray, E. C., and Basmajian, J. V. "Electromyography and Cinematography of Leg and Foot ('Normal' and Flat) During Walking." Anatomical Record 161 (1968): 1. Hermann, G. W. "An Electromyographic Study of Selected Muscles Involved in the Shot Put." Research Quarterly 33 (1962): 1. Houtz, S. J. "Influence of Gravitational Forces on Function of Lower Extremity Muscles." Journal of Applied Physiology 9 (1964): 999. Houtz, S. J., and Fischer, F. J. "An Analysis of Muscle Action and Joint Excursion During Exercise on a Stationary Bicycle." Journal of Bone and Joint Surgery 41-A (1959): 123. Hubbard, A. W. "Homokinetics." In Science and Medicine of Exercise and Sports, edited by W. R. Johnson. New York: Harper & Row, Publishers, 1960. Inman, V. T.; Ralston, H. J.; Saunders, J. B. deC. M.; Feinstein, B.; and Wood, E. W. "Relation of Human Electromyogram to Muscular Tension." Electroencephalography and Clinical Neurophysiology 4 (1952): 187. Joseph, J. Man's Posture; Electromyographic Studies. Springfield, Ill.: Charles C. Thomas, Publisher, 1960. Kamon, E. "Electromyography of Static and Dynamic Postures of the Body Supported on the Arms." Journal of Applied Physiology 21 (1966); 1611. Kaplan, E. B. trans. Duchenne's Physiology of Motion. Philadelphia: W. B. Saunders Company, 1959. Kennedy, J. L., and Travis, R. C. "Prediction of Speed of Performance by Muscle Action Potentials." Science 105 (1947): 410. Kitzman, E. W. "Baseball: Electromyographic Study of Batting Swing." Research Quarterly 35 (1964): 166. Knowlton, G. C.; Bennett, R. L.; and McClure, R. "Electromyography of Fatigue." Archives of Physical Medicine 32 (1951): 648. Licht, Sidney, ed. Electrodiagnosis and Electromyography. 2d ed. New Haven, Conn.: Elizabeth Licht, Publisher, 1961. Lipetz, S., and Gutin, B. "An Electromyographic Study of Four Abdominal Exercises." Medicine and Science in Sports 2 (1970): 35. Lippold, O. C. J. "The Relation Between Integrated Action Potentials in a Human Muscle and its Isometric Tension." Journal of Physiology 117 (1952): 492. Loofbourrow, G. N. "Neuromuscular Integration." In Science and Medicine of Exercise and Sports, edited by W. R. Johnson. New York: Harper & Row, Publishers, 1960. MacConaill, M. A. "The Movements of Bones and Joints: II. Function of the Musculature." Journal of Bone and Joint Surgery 31-B (1949): 100. "Some Anatomical Factors Affecting the Stabilizing Functions of Muscles." Irish Journal of Medical Science 6 (1946): 160. and Basmajian, J. V. Muscles and Movement: A Basis for Human Kinesiology. Baltimore: The Williams & Wilkins Company, 1969. McFarland, G. B.; Krusen, V. L.; and Weatherby, H. T. "Kinesiology of Selected Muscles Acting on the Wrist: Electromyographic Study." Archives of Physical Medicine and Rehabilitation 43 (1962): 165. Merton, P. V. "Problems of Muscular Fatigue." British Medical Bulletin 12 (1956): 219. Miwa, N.; Tanaka, T.; and Matoba, M. "Electromyography in Kinesiologic Evaluations. Subjects on Two Joint Muscle and the Relation Between the Muscular Tension and Electromyogram." Journal of the Japanese Orthopaedic Association 36 (1963): 1025. Norris, Forbes M., Jr. The EMG: A Guide and Atlas for Practical Electromyography. New York: Grune & Stratton, 1963. O'Connell, A. L., and Gardner, E. B. "The Use of Electromyography in Kinesiological Research." Research Quarterly 34 (1963): 166. Perry, J. "The Mechanics of Walking: A Clinical Interpretation." Physical Therapy 47 (1967): 778. Ralston, H. J. "Uses and Limitations of Electromyography in the Quantitative Study of Skeletal Muscle Function." American Journal of Orthodontics 47 (1961): 521. Rodahl, Kaare, and Horvath, Steven M., eds. Muscle as a Tissue. New York: McGraw-Hill Book Company, 1962. Scheving, L. E., and Pauly, J. E. "An Electromyographic Study of Some Muscles Acting on the Upper Extremity of Man." Anatomical Record 135 (1959): 239. Slater-Hammel, A. T. "Action Current Study of Contraction