IVESSA Control of gene expression 040324.ppt
Document Details
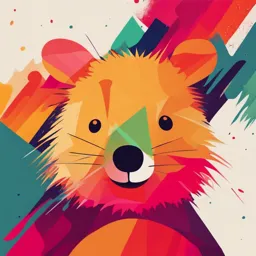
Uploaded by UnquestionableKremlin
Rutgers University
Full Transcript
Control of Gene Expression Andreas Ivessa, Ph.D. Department of Cell Biology and Molecular Medicine [email protected] April 3, 2024 Figures from “Molecular Biology of the Cell” (5th edition) Objectives: To understand that different cell types contain the same DNA but express different protei...
Control of Gene Expression Andreas Ivessa, Ph.D. Department of Cell Biology and Molecular Medicine [email protected] April 3, 2024 Figures from “Molecular Biology of the Cell” (5th edition) Objectives: To understand that different cell types contain the same DNA but express different proteins To know at which steps gene expression is controlled. To understand how DNA-binding proteins bind to DNA and what they recognize. To know how DNA-binding proteins are studied. To know how genes are turned on/off in prokaryotic cells. To understand how transcription is controlled in eukaryotic cells. To know what the mediator protein complex does. To know what transcriptional synergy means. To know how proteins assemble at the promoter site to activate transcription. To know that domains of transcription factors can be separated in their function. To understand how the activity of gene regulatory proteins in eukaryotic cells can be altered To understand how chromatin is opened or closed (condensed). To understand what histone-modifying enzymes do. To understand the action of eukaryotic gene repressor proteins. To know how active and inactive chromatin regions are separated. To understand how active and inactive chromatin regions are inherited. To understand how imprinting works. To understand how gene expression is controlled at the post-transcriptional level. To know what attenuation does. To understand how expression of genes is regulated on the level of splicing. To know what RNA editing means. To understand how translation can be controlled. OUTLINE: Introduction Overview – Gene Expression DNA binding proteins (Transcription factors) Study of DNA binding proteins Prokaryotic Gene Expression Eukaryotic Gene Expression Chromatin structure – activation and repression Separation of active and inactive chromatin Inheritance of active and inactive chromatin Post-transcriptional control Control of translation Cell differentiation depends on changes in gene expression rather than on changes in the nucleotide sequence of the cell’s genome Figure 7-1 Same nuclear genome, but different protein patterns 2nd dimension Differences in the proteins expressed by two human tissues (Protein 2-dimensional gel-electrophoresis: Proteins separated by charge in the first dimension and by molecular weight in the second dimension) 1st dimension Figure 7-4 Evidence that a differentiated cell contains all the genetic instructions necessary to direct the formation of a complete organism Differentiated Chromosomal cell DNA John Gurdon (Nature 1958) Non-differentiated cells lacking functional chromosomal DNA Figure 7-2a Nobel Prize for Medicine 2012 (together with Shinya Yamanaka) Six steps at which eukaryotic gene expression can be controlled NUCLEUS DNA chromosomes Figure 7-5 mRNA CYTOSOL mRNA protein OUTLINE: Introduction Overview – Gene Expression DNA binding proteins (Transcription factors) Study of DNA binding proteins Prokaryotic Gene Expression Eukaryotic Gene Expression Chromatin structure – activation and repression Separation of active and inactive chromatin Inheritance of active and inactive chromatin Post-transcriptional control Control of translation Transcription Transcription factors to start transcription RNA polymerase Promoter and gene regulatory sequences messenger RNA ( mRNA ) Open reading frame RNA polymerase DNA-binding proteins such as transcription factors bind to the surface of DNA without opening the DNA double-helix. Figure 7-6 DNA binding proteins (including transcription factors) bind usually to short DNA sequences. These short DNA sequences are fundamental components of genetic switches. Some examples of transcription factors: Table 7-1 The helix-turn-helix motif is one of the simplest and most common DNA-binding motifs. TURN alphaHELIX Figure 7-10 alphaHELIX alphaHELIX The helix-turn-helix motif is one of the simplest and most common DNA-binding motifs. TURN alphaHELIX alphaHELIX stabilizes the recognition helix major groove Important role in recognizing specific sequences in DNA Figure 7-10 Some examples of helix-turn-helix DNA-binding proteins: Proteins bind DNA as a dimer in which the two copies of the recognition helix (red cylinder) are separated by exactly one turn of the DNA helix (3.4 nm). The other helix of the helix-turn-helix motif (blue color) stabilizes the recognition helix. The lambda repressor and Cro proteins control bacteriophage lambda gene expression. The tryptophan repressor and catabolite activator protein (CAP) control the expression of sets of E. coli (bacterium) genes. Figure 7-11 The helix-loop-helix motif mediates dimerization and DNA binding LOOP HELIX HELIX Oct1 protein Figure 7-22 Helix-Loop-Helix (HLH) Truncated form (green) Full-length form (blue) NO DNA binding, but dimerization Figure 7-24 The leucine zipper motif mediates both DNA binding and dimerization: Two alpha helical DNA binding domains (bottom) dimerize through their alpha helical leucine zipper region (top) to form an inverted Y-shaped structure. Each arm of the Y is formed by a single alpha helix, one from each monomer, that mediates binding to a specific DNA sequence in the major groove of DNA. Example: Gcn4 protein: transcription factor regulating amino acid biosynthesis. Figure 7-19 Dimerization region is rich in leucine Hetero-dimerization of leucine zipper proteins can alter their DNA-binding specificity Homo-dimer Same sequence Figure 7-20 Homo-dimer Same sequence Hetero-dimer Different sequence Beta-sheets can also recognize DNA: Beta-sheets major groove The bacterial methionine repressor protein Figure 7-17 OUTLINE: Introduction Overview – Gene Expression DNA binding proteins (Transcription factors) Study of DNA binding proteins Prokaryotic Gene Expression Eukaryotic Gene Expression Chromatin structure – activation and repression Separation of active and inactive chromatin Inheritance of active and inactive chromatin Post-transcriptional control Control of translation Study of DNA-binding proteins: A gel mobility shift assay readily detects sequence-specific DNA-binding proteins: DNA is negatively charged and migrates to the positive electrode. * * * * * * * * * * * * * * poly-acrylamide gel Figure 7-27a DNA is charged negatively on the surface Study of DNA-binding proteins: A gel mobility shift assay readily detects sequence-specific DNA-binding proteins: DNA is negatively charged and migrates to the positive electrode. Free DNA migrates fast through the poly-acryl-amide gel. * * * * * * * * * * * * * * DNA covered with proteins will migrate slowly. Proteins, such as e.g. nuclear protein extracts, are mixed with labeled DNA molecules in the assay. Figure 7-27a poly-acrylamide gel Study of DNA-binding proteins: DNA footprinting: radioactive label Figure 7-29a Study of DNA-binding proteins: DNA footprinting: 1. In a pre-experiment it will be determined how much nuclease/chemical needs to be added to the DNA fragment so that a ladder (as shown below) will be created (thus: short, medium and long-sized fragments). If too much nuclease/chemical is added, only short DNA fragments are created, if too little is added the DNA fragments are too long. 2. In the actual experiment proteins are added which bind to DNA (DNA footprint) and protect the DNA from DNA digestion by the nuclease/chemical. Figure 7-29b Protected by protein attached to DNA Study of DNA-binding proteins: DNA affinity chromatography: Figure 7-28 1 Study of DNA-binding proteins: Chromatin immuno precipitation: Determination whether a protein associates with DNA at a specific location on the chromosome. Protein-complex bound to chromosomal DNA in the cell DNA Promoter Open Reading Frame Add cross-linking agent (e.g., formaldehyde) to immobilize protein-DNA interactions in the living cell Fragmentation of DNA by sonication (Proteins remain attached by the cross-link) 2 Study of DNA-binding proteins: Chromatin immuno precipitation: Determination whether a protein associates with DNA at a specific location on the chromosome. un-specific specific antibody Immuno-precipitation of protein (attached to DNA) Fragment A Fragment B Amplification of DNA fragments by polymerase-chain-reaction (PCR) Fragment A Low Enrichment (Background) PCR-amplified DNA fragments are analyzed by agarose gel electrophoresis Large enrichment (because antibody precipitated a specific protein attached to DNA) Fragment B OUTLINE: Introduction Overview – Gene Expression DNA binding proteins (Transcription factors) Study of DNA binding proteins Prokaryotic Gene Expression Eukaryotic Gene Expression Chromatin structure – activation and repression Separation of active and inactive chromatin Inheritance of active and inactive chromatin Post-transcriptional control Control of translation Transcription Transcription factors to start transcription RNA polymerase Promoter and gene regulatory sequences messenger RNA ( mRNA ) Open reading frame RNA polymerase PROKARYOTIC gene expression Polycistronic messenger RNAs: One mRNA encodes multiple proteins The clustered genes in E. coli that code for enzymes that synthesize the amino acid tryptophan: Bind specific proteins that regulate the expression of genes Figure 7-34 Switching the genes on and off which are needed for the synthesis of the amino acid tryptophan: The end-product tryptophan binds to the repressor. The repressor protein binds then to DNA and prevents transcription. Figure 7-35 Summary of the mechanisms by which specific gene regulatory proteins control gene transcription in prokaryotes: e.g. substrate e.g. end-product e.g. end-product Figure 7-37 e.g. substrate Dual control of the Lac operon: The lac operon codes for proteins required to transport the sugar lactose into the cell and break it down. Glucose Galactose Lactose Catabolite Activating Protein (CAP) Figure 7-39 The gene control region for a typical EUKARYOTIC gene consists of a promoter plus regulatory sequences: The mediator protein complex helps to establish interactions between the components Figure 7-44 Transcriptional synergy: TATA sequence on DNA: Binds general transcription factor TFIID which is needed for the recruitment of RNA polymerase II to the promoter 1 activator 1 activator 2 activators Figure 7-48 Eukaryotic gene regulatory proteins often assemble into complexes on DNA: Figure 7-51 The integration of multiple inputs at a promoter: DNA looping can contribute to gene activation at a distance. Figure 7-58 The activation and DNA-binding domains of a gene activator protein can be separated: NO binding! Figure 7-45 Alteration of the activity of gene regulatory proteins in eukaryotic cells: Regulatory protein is synthesized only when needed Phosphorylation Figure 7-59 Phosphorylation A single gene regulatory protein can coordinate the expression of several different genes: Figure 7-74 OUTLINE: Introduction Overview – Gene Expression DNA binding proteins (Transcription factors) Study of DNA binding proteins Prokaryotic Gene Expression Eukaryotic Gene Expression Chromatin structure – activation and repression Separation of active and inactive chromatin Inheritance of active and inactive chromatin Post-transcriptional control Control of translation Eukaryotic activator proteins can alter local features in chromatin structure to stimulate transcription initiation: DNA is wrapped by histones (H1, H2A, H2B, H3, H4) in nucleosomes forming chromatin. Figure 7-46 (e.g. acetylation, methylation) An order of events leading to transcription initiation of a specific gene: By remodeling the chromatin and modifying histones, chromatin will open. Transcription factors can bind to the promoter region and activate the gene. Figure 7-49 Action of eukaryotic gene repressor proteins: 1. Binds general transcription factor TFIID which is needed for the recruitment of RNA polymerase II to the promoter Figure 7-50a Action of eukaryotic gene repressor proteins: 2. Figure 7-50b Action of eukaryotic gene repressor proteins: 3. Figure 7-50c Action of eukaryotic gene repressor proteins: 4. Figure 7-50d Action of eukaryotic gene repressor proteins: Removes the stimulus for transcription 5. Closed chromatin Stimulates transcription HAT Figure 7-50e Open chromatin HAT: Histone acetyl transferase HDAC: Histone deacetylase K: lysine (positively charged amino acid) Acetylation of specific lysines in histones causes the affected nucleosomes to appear rather neutral. DNA is open and accessible. Action of eukaryotic gene repressor proteins: 6. Figure 7-50f Schematic diagram summarizing the properties of insulators and barrier sequences: In-active DNA (ON or OFF) Figure 7-62 Active DNA Constitutive in-active DNA Inheritance of methylated DNA Formation of 5-methylcytosine occurs by methylation of a cytosine base in the DNA double helix. How DNA methylation patterns are faithfully inherited. Figure 7-80 Mechanisms that can produce a form of epigenetic inheritance in an organism The ability of a daughter cell to retain a memory of the gene expression patterns that were present in the parent cell is an example of epigenetic inheritance : OFF Gene OFF Cell division Gene OFF Gene Nucleosomes Gene Cell division Gene Gene Figure 7-86a Mechanisms that can produce a form of epigenetic inheritance in an organism Figure 7-86a Schematic diagram showing how a positive feedback loop can create cell memory Figure 7-68 Mechanisms that can produce a form of epigenetic inheritance in an organism Figure 7-86b Mechanisms that can produce a form of epigenetic inheritance in an organism Figure 7-86c Imprinting in mammals: Methylation NO methylation Figure 7-82 Methylation X-chromosome inactivation: Mammals: Female: 2 X chromosomes (One X chromosome is silenced by chromosome condensation.) Male: 1 X (active) and 1 Y chromosome Figure 7-90 OUTLINE: Introduction Overview – Gene Expression DNA binding proteins (Transcription factors) Study of DNA binding proteins Prokaryotic Gene Expression Eukaryotic Gene Expression Chromatin structure – activation and repression Separation of active and inactive chromatin Inheritance of active and inactive chromatin Post-transcriptional control Control of translation Post-transcriptional controls of gene expression: NUCLEUS – TRANSCRIPTION CYTOPLASM – TRANSLATION Figure 7-92 Attenuation: In this example, the riboswitch controls expression of the purine biosynthetic genes. When guanine levels in cells are low, an elongating RNA polymerase transcribes the purine biosynthetic genes. If guanine is abundant, it binds the riboswitch, causing it to undergo a conformational change that forces the RNA polymerase to terminate transcription. Figure 7-93a Alternative RNA splicing: In each case a single type of RNA transcript is spliced in two alternative ways to produce two distinct messenger RNAs (1 and 2). The dark blue boxes mark exon sequences that are retained in both mRNAs. The light blue boxes mark possible exon sequences that are included in only one of the mRNAs. The boxes are joined by red lines to indicate where intron sequences (yellow) are removed. Figure 7-94 RNA editing: Specific nucleotides are inserted into the mRNA sequence or existing nucleotides are changed by deamination (e.g. adenine to inosine, cytosine to uracil). Results: alteration in protein sequence. In humans: Estimated over 1000 genes are affected. Figure 7-100 Mechanisms for the localization of messenger RNAs. Figure 7-104 Mechanisms of translation control: Figure 7-106 Micro RNA processing and mechanism of action: Micro RNA (miRNA): Short non-coding RNAs. Humans express more than 400 different miRNAs, and these appear to regulate at least one-third of all human protein-encoding genes. Once made, miRNAs base-pair with specific mRNAs and regulate their stability and their translation. The miRNAs undergo a special type of processing, after which the miRNA is assembled with a set of proteins to form an RNA-induced silencing complex (RISC). Figure 7-112 Sample Question: Gene expression is controlled on the level of A. transcription and RNA processing B. RNA transport, RNA localization, and RNA degradation C. translation D. protein activity E. all of the above