Isomerism in Carbohydrates PDF
Document Details
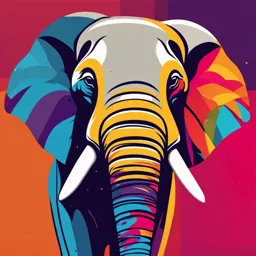
Uploaded by ProblemFreeRuby6628
Unilorin
Tags
Summary
This document explains the concept of isomerism in carbohydrates, focusing on structural differences in monosaccharides. It uses Fischer projections and details how stereoisomers, such as D- and L-isomers, arise due to asymmetric centers. The document also touches on concepts like aldoses, epimers and cyclic forms.
Full Transcript
Structure and Isomerism in Carbohydrates Isomers are compounds that have the same structural formula but different spatial configuration. As a result of the asymmetric carbon atoms contained in monosaccharides, it is important to correctly position the spatial arrangement of H and OH groups when wri...
Structure and Isomerism in Carbohydrates Isomers are compounds that have the same structural formula but different spatial configuration. As a result of the asymmetric carbon atoms contained in monosaccharides, it is important to correctly position the spatial arrangement of H and OH groups when writing molecular formula. An asymmetric carbon atom, also known as a chiral carbon is a carbon atom to which 4 different functional groups are attached. Molecules with asymmetric or chiral carbon are capable of existing in optically active isomeric forms. All monosaccharides with the exception of dihydroxyacetone contain one or more asymmetric (chiral) carbon atoms and thus occur in optically active isomeric forms. The convention for presenting linear monosaccharides that allows obvious visualization of their chiral centre is Fischer projection. Using the Fischer projection formula, the stereochemistry of the monosaccharide molecule can be reported as D or L by looking at the position of the H and OH groups on the chiral carbon at the bottom that is farthest away from the aldehyde or ketone functional groups as shown on figure 1. Figure 1: D and L isomers of glyceraldehyde Conventionally, one of these two forms is designated the D isomer, the other the L isomer as already stated. It should be noted that most sugars naturally occur in the D form. The number of possible stereoisomers for a given monosaccharide depends on the number of asymmetric carbon atoms. This can be calculated using the formula 2n Where n, is the number of asymmetric carbon atoms. Using the 2 n formula, the simplest aldose triose sugar, glyceraldehyde, contains one chiral centre, which is the middle carbon atom and therefore has two different optical isomers or enantiomers as shown on figure 1. Glyceraldehyde = 21 = 2 Aldotetroses = 22 = 4 stereoisomers including D –Erythrose, D- Threose and their L enantiomers Aldopentoses = 23 = 8 including D- ribose, D- arabinose, D-xylose, D-lyxose and their L enantiomers Aldohexoses = 24 = 16 including D- allose, D-altrose, D- glucose, D-mannose, D- gulose, D- idose, D-galactose, D-talose and their L enantiomers Figure 2: D- isomers of aldose monosaccharides. (The chiral carbon on this glucose molecule are coloured pink) Figure 3: D and L isomers of glucose Epimers: are molecules that differ only in the configuration around one carbon atom. Examples include D-glucose and D-mannose, which differ only in stereochemistry at C-2, as well as D-glucose and D – galactose which differ at C-4. Figure 3: Epimers of glucose Formation of cyclic monosaccharide structures: In 1883, Emil Fisher proposed the open chain projection formula (used above) and hemiacetal ring structure of glucose, hence, the name Fischer projection formula. Although the straight chain Fischer projection was sufficiently simplified to illustrate stereochemistry in monosaccharides, it was later observed by Sir Haworth in 1925 that glucose and more generally aldotrioses and most pentose sugar do not exist as rectangles in biological systems, but rather as a cyclic structure referred to as a pyranose ring shown in figure 4. Figure 4: Pyranose ring The cyclic structures form as a result of covalent interaction between the carbonyl group (that is the aldehyde or ketone functional group) and alcohols (that is the oxygen atom of a hydroxyl group) on carbon 5 and 4 for hexose and pentose sugars respectively. The reaction is given in figure 5. Aldohexoses also exist in cyclic forms having five membered rings. This is because they resemble the five membered ring compound furan, are called furanoses. However, the six-membered aldopyranose ring is much more stable than the aldofuranose ring and predominates in aldohexose solutions. Note: Only aldoses having five or more carbon atoms can form pyranose rings. Figure 5: formation of hemiacetals and hemiketals Harworth projections are commonly used to show the stereochemistry of cyclic structures or ring forms of monosaccharides. One practical application of the Haworth projection is to show anomeric conformation of sugars. For example, in figure 5, a sugar molecule may either exist as α or β if the OH group on carbon 1 is down or up respectively. Figure 5: formation of a cyclic monosaccharide structure showing α and β anomers of glucose. Anomers: are isomeric forms of monosaccharides that differ only in their configuration about the hemiacetal or hemiketal carbon atom. Therefore, the hemiacetal carbon atom at carbon 1 is called the anomeric carbon. In an aqueous solution, the α and β anomers of D-glucose interconvert by a process called mutarotation. This occurrence eventually results in the formation of identical equilibrium mixtures. solution, Typically, β-D-glucopyranose occurs predominantly and accounts for about 63%, α- D-glucopyranose 36% while glucofuranose form account for 1% of molecules in an aqueous solution. Note: Ketohexoses also occur in α and β anomeric forms. In this case, the hydroxyl group at carbon 5 or 6 (for pentose or hexose respectively) reacts with the keto group at carbon 2 to form a furanose (or pyranose) ring containing a hemiketal linkage as already shown in figure 5. D-Fructose readily forms the furanose ring and the more common anomer of this sugar in combined forms or in derivatives is -D-fructofuranose. Figure 6: Haworth projections of hexose pyranose and furanose