IoT Sem Q&A PDF
Document Details
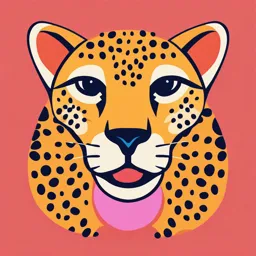
Uploaded by CourageousKrypton7509
Tags
Summary
This document is a question and answer document on the topic of Internet of Things (IoT), covering definitions, characteristics, components, and various applications. It also discusses the physical design of IoT systems and different types of sensors used. The document appears to be study material.
Full Transcript
IOT SEM Q&A Answers are generated using GPTs from OpenAI, Gemini & Mistral and also gathered information from various other platforms. Refer Digital Notes/PPTs or other sources, if you feel this answers doesn’t match well with the questions. Join for more pdfs - https://chat.whatsa...
IOT SEM Q&A Answers are generated using GPTs from OpenAI, Gemini & Mistral and also gathered information from various other platforms. Refer Digital Notes/PPTs or other sources, if you feel this answers doesn’t match well with the questions. Join for more pdfs - https://chat.whatsapp.com/GSdHVI5AgoaIe92jLO5nCX UNIT – I 1) Define IOT. Explain Characteristics and components of IOT. A: Internet of Things (IoT) The Internet of Things (IoT) refers to a network of interconnected physical devices, embedded systems, and mechanical and digital machines that gather, process, and share data through the internet. These devices are equipped with sensors, actuators, and connectivity technologies to interact with their environment and communicate with other devices. >Characteristics of IoT Interconnectivity: IoT devices are connected to the internet, allowing them to communicate and exchange data with other devices. Real-time Data: IoT devices generate and transmit data in real-time, providing up-to-date information about the physical world. Remote Monitoring and Control: IoT devices can be monitored and controlled remotely, enabling efficient management and operation. Automation: IoT devices can automate tasks and processes, improving efficiency and reducing human intervention. Scalability: IoT systems can be scaled to accommodate a large number of devices and data volumes. Integration: IoT can be integrated with other technologies, such as artificial intelligence, machine learning, and big data analytics, to enhance its capabilities. >Components of IoT Devices: These are the physical objects that are connected to the internet. They can be anything from sensors and actuators to appliances and vehicles. Sensors: These devices collect data from the physical world, such as temperature, humidity, pressure, motion, and light. Actuators: These devices perform actions based on the data collected by sensors, such as controlling lights, opening doors, or adjusting temperature. Connectivity: This refers to the technology that allows devices to connect to the internet. Common connectivity options include Wi-Fi, Bluetooth, cellular networks, and LoRaWAN. Network Infrastructure: This includes the routers, switches, and other hardware that make up the network connecting IoT devices. Cloud Platform: This is a cloud-based platform that stores, processes, and analyzes data from IoT devices. Applications: These are software programs that use IoT data to provide valuable insights or automate tasks. 2) Discuss various application areas of IoT. A: Applications of the Internet of Things (IoT) The Internet of Things (IoT) has revolutionized numerous industries and aspects of daily life. Here are some key application areas: >Smart Homes Home Automation: Control lights, thermostats, appliances, and security systems remotely. Energy Management: Optimize energy consumption and reduce costs. Entertainment: Control smart TVs, speakers, and other entertainment systems. >Healthcare Remote Patient Monitoring: Monitor patients' vital signs and health conditions from afar. Wearable Devices: Track fitness, sleep, and other health metrics. Medical Devices: Improve the accuracy and efficiency of medical procedures. >Industrial IoT (IIoT) Predictive Maintenance: Use sensor data to predict equipment failures and prevent downtime. Supply Chain Management: Track products and optimize logistics. Quality Control: Monitor production processes and ensure product quality. >Agriculture Precision Agriculture: Optimize farming practices based on real-time data about soil, weather, and crop conditions. Livestock Monitoring: Track animal health and behavior. Irrigation Management: Efficiently manage water resources. >Retail Smart Retail: Enhance customer experience through personalized recommendations and in-store analytics. Inventory Management: Optimize stock levels and prevent stockouts. Supply Chain Visibility: Track products from manufacturing to delivery. >Environmental Monitoring Air Quality Monitoring: Track air pollution levels and identify sources of contamination. Water Quality Monitoring: Monitor water quality and detect pollutants. Climate Change Monitoring: Collect data on climate patterns and trends. >Other Applications Smart Cities: Improve urban infrastructure and services through IoT-enabled solutions. Smart Grids: Optimize energy distribution and consumption. Security and Surveillance: Enhance security through surveillance cameras, access control systems, and other IoT devices. 3) Explain in detail about Physical design of IOT with neat suitable diagram. A: Figure 1-- C:\Users\VARSHITH HOWDEKAR\Desktop\2111CS020621\Zeta\4-1\Massssss.jpg The physical design of IoT systems involves selecting and configuring appropriate hardware components to meet the specific requirements of the application. The image you provided offers a comprehensive overview of the key components and interfaces involved. By carefully considering factors such as power efficiency, environmental conditions, scalability, security, and cost, engineers can design IoT devices that are reliable, efficient, and meet the needs of diverse applications. Connectivity: USB Host: This interface enables direct connection to various peripherals, such as sensors, actuators, or storage devices. It provides flexibility and ease of integration. RJ45/Ethernet: This wired network connection ensures reliable and high-bandwidth communication. It is suitable for applications requiring robust and consistent connectivity. Processor: The CPU serves as the brain of the IoT device, processing data, executing algorithms, and controlling the overall operation. Its capabilities determine the device's performance and computational power. Audio/Video Interfaces: These interfaces are essential for applications involving multimedia content, such as smart speakers, video surveillance systems, or virtual reality devices. They enable seamless interaction with audio and visual information. I/O Interfaces: The various I/O interfaces allow the device to interact with the physical world. They are crucial for connecting sensors, actuators, and other external components. UART: Simple serial communication for basic data exchange. SPI: Synchronous serial communication for high-speed data transfer. I2C: Synchronous serial communication for low-speed, multi-master applications. CAN: Robust and reliable communication protocol for real-time applications, especially in automotive and industrial settings. Memory Interfaces: NAND/NOR: Non-volatile memory for storing data persistently, even when the device is powered off. DDR: Dynamic random access memory for temporary data storage. The choice of DDR generation (DDR1, DDR2, DDR3) affects performance and power consumption. Graphics: The GPU is responsible for rendering graphics and displaying visual information. Its capabilities are essential for applications requiring rich user interfaces or advanced visualization. Storage Interfaces: These interfaces allow the device to connect to external storage devices, such as SD cards or MMCs. They provide additional storage capacity for data or media files. Physical Design Considerations: Power Efficiency: IoT devices often operate on battery power, so optimizing power consumption is crucial. Selecting low-power components and implementing energy- saving techniques are essential. Environmental Factors: The device's operating environment (temperature, humidity, vibration) must be considered to ensure its durability and reliability. Scalability: The design should accommodate future growth and expansion, allowing for easy addition of new components or features. Security: Protecting against unauthorized access and data breaches is paramount. Physical security measures, such as tamper-resistant enclosures and secure boot processes, can help mitigate risks. Cost: Balancing performance, features, and cost is essential. Careful selection of components and manufacturing processes can help optimize the overall cost. 4) Define sensor and mention different types of sensors available in the market. A: A sensor is a device that detects and responds to changes in the environment. It converts physical or chemical stimuli into signals that can be interpreted by humans or machines. Sensors are used to measure parameters such as temperature, pressure, motion, light, humidity, and various other physical properties, depending on their application. Different Types of Sensors Available in the Market: Temperature Sensors: Thermocouples: Measure temperature by generating a voltage when two different metals are heated. RTDs (Resistance Temperature Detectors): Measure temperature by correlating the resistance of a conductor with temperature. Thermistors: A type of resistor that changes resistance with temperature. Infrared Sensors: Measure temperature from the emitted infrared radiation. Pressure Sensors: Piezoelectric Sensors: Detect changes in pressure based on the electrical charge generated by stress on a material. Strain Gauge Sensors: Measure the deformation of an object due to applied pressure. Capacitive Pressure Sensors: Use changes in capacitance caused by pressure changes to determine pressure. Proximity Sensors: Inductive Sensors: Detect metallic objects by generating a magnetic field. Capacitive Sensors: Detect changes in capacitance when objects approach. Ultrasonic Sensors: Use sound waves to detect the presence or distance of an object. Optical Sensors: Detect objects through light reflection or interruption. Light Sensors: Photodiodes: Convert light into electrical current. Phototransistors: Amplify the current generated by light exposure. LDR (Light Dependent Resistor): Resistance varies with the intensity of light. Photoresistors: Measure light intensity based on resistance changes. Motion Sensors: PIR (Passive Infrared) Sensors: Detect infrared radiation emitted by humans or animals. Ultrasonic Sensors: Use sound waves to detect motion or measure distance. Radar Sensors: Measure the velocity and position of objects. Humidity Sensors: Capacitive Humidity Sensors: Measure humidity by changes in capacitance. Resistive Humidity Sensors: Measure changes in resistance with humidity levels. Thermal Conductivity Sensors: Measure humidity based on thermal conductivity of the air. Gas Sensors: Electrochemical Sensors: Measure the concentration of gases by a chemical reaction with the gas. Metal Oxide Sensors: Detect gases based on changes in resistance when exposed to a gas. Infrared Sensors: Detect gases by measuring the absorption of infrared light. Force and Load Sensors: Load Cells: Measure weight or force through strain gauges. Piezoelectric Force Sensors: Use the piezoelectric effect to measure force or pressure. Acceleration Sensors: Accelerometers: Measure acceleration or vibration using changes in capacitance or piezoelectric effects. Gyroscope Sensors: Measure angular velocity to determine orientation or rotational motion. pH Sensors: Ion-Selective Electrodes (ISE): Measure the pH level based on the electrical potential generated by ions in solution. 5) Discuss IoT levels and deployment templates. A: Levels and Deployment Templates IoT systems can be categorized into different levels based on their complexity and functionality. Understanding these levels helps in designing and implementing effective IoT solutions. IoT Levels Device Level: This is the most basic level, where individual IoT devices are connected to the internet. These devices typically consist of sensors, actuators, and microcontrollers. Gateway Level: At this level, multiple devices are connected to a gateway, which acts as a bridge between the devices and the network. Gateways aggregate data from the devices, perform basic processing, and provide security. Network Level: This level involves the network infrastructure that connects the devices and gateways. It can be a local area network (LAN), a wide area network (WAN), or a cellular network. Application Level: This is the highest level, where data collected from the devices is processed, analyzed, and used to provide valuable insights or trigger actions. Applications can be web-based, mobile-based, or embedded in other systems. IoT Deployment Templates IoT deployment templates are pre-defined architectures or models that can be used as a starting point for building IoT solutions. They provide a structured approach to designing and implementing IoT systems. >Common IoT Deployment Templates: Centralized Architecture: In this template, all data is sent to a central server for processing and analysis. This is suitable for simple IoT applications with a limited number of devices. Decentralized Architecture: In this template, data is processed and analyzed at the edge devices or gateways, reducing latency and improving scalability. This is suitable for large- scale IoT deployments with geographically distributed devices. Hybrid Architecture: This is a combination of centralized and decentralized architectures, where some data is processed at the edge and some is sent to a central server. This provides a balance between performance and scalability. Fog Computing: This template involves deploying computing resources closer to the edge devices, enabling real-time processing and analysis of data. This is suitable for applications that require low latency and local decision-making. Cloud-Based IoT: In this template, IoT data is stored and processed in the cloud. This provides scalability, flexibility, and access to advanced analytics tools. However, it may introduce latency and security concerns. 6) Explain in detail about Logical design of IOT with a neat suitable diagram. A: It is the actual design of the IoT system. It illustrates the assembling and configuration of the components i.e. computers, sensors, and actuators. The logical design of IoT is composed of: IoT functional blocks IoT communications models IoT communication APIs 1. IoT functional blocks The functional blocks of IoT systems provide sensing, identification, actuation, management, and communication capabilities to the IoT ecosystem. The devices of the functional blocks handle the communication between the server and the host. This enables monitoring of control functions, managing the data transfer, securing the IoT system using authentication, and providing an interface for controlling and monitoring various functions. It is the most crucial part of the logical and physical designs of IoT. The Functional blocks of IoT are: Device: Devices provide sensing, actuation, monitoring, and control functions to the IoT ecosystem. Communication: Manages communication for the IoT system. Services : Services perform the functions of device monitoring, device control, data publishing, and device discovery. Management : gives functions to govern the IoT system.\ Security : provides security to the IoT system. It performs authentication, authorization, message and content integrity, and data security functions. Application : An interface where the users can control and monitor various aspects of the IoT system. It allows the users to view the system status and analyze the processed data. 2. IoT Communication Models\ The communication models of IoT are used for communicating between the system and the server. The types of IoT communication models are : Request-Response Model In this communication model, the client sends requests to the server and the server responds to their requests. After receiving a request, the server decides how to respond by fetching the data, retrieving resource representation, preparing the response, and then sending the response to the client. The request-response protocol between a client and a server is HTTP. Publisher-Subscriber Model This model is made up of three entities: Publishers, Brokers, and Consumers. Publishers- it is the source of data that sends the data to the topic. Consumers- they subscribe to the topics. Brokers- they accept data from publishers and send it to the consumers. Push-Pull Model This model is made up of data publishers, data consumers, and data queues. Publishers- they publish the message and push it into the queue. Consumers- they present on the other side and they pull the data out of the queue. Queues- it helps in decoupling the messages between the producer and consumer. Exclusive Pair It is a bi-directional model that includes full-duplex communication between client and server. The client sends a request and the server keeps the record of all the connections. In this model, only WebSocket-based communication API is based. 3. IoT communication API There are two types of communication APIs – REST-based communication APIs REST stands for Representational State Transfer. It is a set of architectural protocols by which you can design web services and web APIs. These web services and APIs focus on a system’s resources and how resource states are addressed and transferred. It has a request-response communication model. Its architectural constraints are components, connectors, and data elements. All of them are included within a distributed hypermedia system. Web Socket-Based Communication APIs Web Socket API is the bi-directional, full-duplex communication model between clients and servers. It does not require a new connection to set up for each message between clients and servers. After the connection is set the messages can be sent and received continuously without any interruption. It is best for IoT Applications with low latency or high throughput requirements Figure 2- C:\Users\VARSHITH HOWDEKAR\Desktop\2111CS020621\Zeta\4-1\Bujjithalli.jpg 7) Define actuator and mention different types of actuators available in the market. A: An actuator is a device that converts an input signal (often electrical, hydraulic, or pneumatic) into physical motion or mechanical action. It is a key component in control systems that perform a specific task, such as opening a valve, moving a robotic arm, or adjusting the position of a mechanical component. Actuators are widely used in systems requiring automated movement or control, translating energy into motion. Different Types of Actuators Available in the Market: Electric Actuators: -DC Motor Actuators: These actuators use direct current (DC) motors to create rotational movement. They are commonly used for small-scale, precise motion control. -AC Motor Actuators: These actuators use alternating current (AC) motors and are typically used in larger systems requiring high power or continuous operation. -Stepper Motors: Stepper motors move in discrete steps and provide precise control over angular position. They are commonly used in applications requiring precise, repeatable motion. -Servo Motors: These motors provide high torque and precise position control, often used in robotics, CNC machines, and automation systems. -Linear Electric Actuators: These actuators convert electrical energy into linear motion (back- and-forth or push-pull movement). They are widely used in applications like lifts, robotic arms, and positioning systems. Pneumatic Actuators: -Linear Pneumatic Actuators: Use compressed air to produce linear motion. Common in industrial automation, they are often used for tasks like opening and closing valves or lifting objects. -Rotary Pneumatic Actuators: Use compressed air to generate rotational motion. These actuators are often employed in machinery for turning or rotating components. -Pneumatic Cylinders: Typically used for applications that require linear force, such as in assembly lines, material handling, and packaging. Hydraulic Actuators: -Hydraulic Cylinders: These actuators use pressurized hydraulic fluid to produce linear motion. They are known for providing high force and are often used in heavy-duty machinery, construction equipment, and automotive applications. -Hydraulic Motors: These actuators use hydraulic fluid pressure to produce rotational motion. Commonly found in applications that require high torque, such as industrial machines and lifting equipment. Thermal Actuators: -Shape Memory Alloys (SMA): Materials that change shape in response to temperature changes. When heated, SMAs can contract or expand, making them useful for applications where compact, precise motion is required (e.g., actuators in medical devices or aerospace systems). -Bimetallic Actuators: These use the differential expansion of two metals to create a bending action when the temperature changes, often used in thermostats and circuit breakers. Magnetic Actuators: -Electromagnetic Actuators: These actuators use electromagnetic forces to generate motion. They are used in various devices such as solenoids, relays, and in electromagnetic valves for controlling the flow of fluids or gases. -Voice Coil Actuators: These actuators use the magnetic force generated between a coil and a magnet to produce precise linear motion, often used in high-precision applications such as disk drives or autofocus systems in cameras. Piezoelectric Actuators: -These actuators use piezoelectric materials that change shape when an electric field is applied. - --They provide very precise motion control and are used in applications requiring extremely fine adjustments, such as in optics, microscopy, and precision manufacturing. Mechanical Actuators: -Gear-Based Actuators: Use gears to transmit rotational motion from a motor to the desired mechanism. They are commonly found in robotics, mechanical systems, and machines. -Screw Jack Actuators: These actuators use screw threads to convert rotational motion into linear motion, typically used for lifting or positioning heavy loads. Vacuum Actuators: -These actuators use vacuum pressure to generate motion, typically used in automotive systems (e.g., controlling valves and doors) and other applications requiring low-force control systems. 8) Explain the various communication models used in IOT. A: In the Internet of Things (IoT), communication between devices, sensors, and actuators is essential for data exchange and system functioning. Several communication models define how information is transmitted and received in IoT systems. These models are often designed based on factors like power consumption, distance, speed, reliability, and scalability. Below are the most commonly used communication models in IoT: 1. Device-to-Device Communication (D2D): In D2D communication, IoT devices communicate directly with each other without needing an intermediary like a gateway or server. This is ideal for scenarios where devices need to exchange data quickly and in real-time. Example: A smart thermostat communicating with a smart light bulb to adjust lighting based on the room's temperature. Advantages: Low latency, faster communication. Disadvantages: Limited range, not scalable for large networks. 2. Device-to-Gateway Communication (D2G): In this model, IoT devices communicate with a local gateway that acts as an intermediary between devices and the cloud. The gateway often processes or aggregates data before transmitting it to the cloud or a remote server. Example: A smart home system where sensors (like motion detectors or temperature sensors) communicate with a hub, which then sends the data to the cloud for analysis. Advantages: Reduces cloud traffic, local data processing, and provides a central point for management. Disadvantages: The gateway can become a bottleneck, and the range is still limited to the gateway's proximity. 3. Device-to-Cloud Communication (D2C): In this model, IoT devices send data directly to the cloud via a gateway or directly over the internet. The cloud serves as the central hub for data storage, processing, and analysis. Example: A wearable fitness tracker sending data (like heart rate or steps) directly to the cloud for processing and long-term storage. Advantages: Scalable, allows for centralized management and analytics. Disadvantages: Dependent on internet connectivity and cloud servers, can introduce latency, higher power consumption for continuous communication. 4. Cloud-to-Device Communication (C2D): In this model, cloud servers send commands, instructions, or updates to IoT devices. It is commonly used in IoT applications where the devices need to receive remote instructions or configurations. Example: A smart thermostat receiving an update from a cloud server to adjust its settings or apply new energy-saving algorithms. Advantages: Centralized control, remote management of IoT devices. Disadvantages: Dependence on cloud infrastructure, potential delays in command execution due to network latency. 5. Machine-to-Machine Communication (M2M): M2M communication involves direct communication between machines (IoT devices) without human intervention. It is typically used for industrial applications, where devices exchange data to automate processes. Example: Two machines on a production line communicating with each other to ensure that parts are delivered to the correct location. Advantages: Autonomous operations, efficient data exchange. Disadvantages: Complexity in setup and integration, possible network congestion in large networks. 6. Cloud-to-Cloud Communication (C2C): This model involves communication between multiple cloud systems, where data or information is exchanged between different cloud platforms or services. It's used in cases where IoT devices are integrated with services hosted on different cloud infrastructures. Example: A smart agriculture platform communicating with a weather service cloud to access weather forecasts and optimize irrigation schedules. Advantages: Facilitates inter-cloud data exchange and service integration. Disadvantages: Security and privacy concerns, complex integration. 9) Discuss the general block diagram of an IOT device. A: Refer Any other sources for answer 10) Explain a case study “Smart Home Automation” using IOT. A: Introduction to Smart Home Automation A Smart Home uses IoT (Internet of Things) technology to control and automate various home appliances, devices, and systems like lighting, heating, cooling, security, and entertainment. The goal of smart home automation is to increase convenience, energy efficiency, security, and comfort while reducing manual effort in managing household tasks. In this case study, we explore how an IoT-based smart home automation system works, focusing on key components such as sensors, controllers, communication networks, and user interfaces, and highlighting the benefits and challenges. The objective of implementing smart home automation using IoT is to provide a fully integrated system that allows users to control and monitor home devices remotely via smartphones, tablets, or voice assistants. The main goals include: Energy Efficiency: Automatically adjusting lighting, heating, and cooling to save energy. Convenience: Allowing remote control of devices and providing real-time monitoring. Security: Enhancing home security by automating door locks, surveillance cameras, and alarm systems. Comfort: Personalizing the home environment (e.g., adjusting thermostat settings and lighting based on user preferences). Working of a Smart Home Automation System Sensing and Data Collection: The system begins by collecting data from sensors embedded in the environment. For example, temperature sensors constantly monitor the home's indoor temperature, and motion sensors detect any movement within rooms. Processing and Decision Making: The data from the sensors is transmitted to the microcontroller or processor (such as an Arduino or Raspberry Pi). Based on predefined rules or algorithms, the controller processes the data and decides whether to trigger actions. For example, if the temperature rises above a threshold, the system may activate the air conditioning system. If motion is detected in a room and no one is home, lights might be turned off automatically to conserve energy. Communication: The microcontroller sends the data to the cloud platform via Wi-Fi or another communication protocol. The cloud platform stores and processes this data and can also provide real-time updates to the homeowner via the mobile app or web dashboard. User Interaction:The user interacts with the system through the app or voice assistant. They can check the status of devices, receive alerts (such as when doors are left open or when a gas leak is detected), or manually control devices like lights or thermostats. Automation: Based on the data received and the rules defined, the system can automatically adjust home conditions without requiring user intervention. For instance: Energy Management: The system may adjust the thermostat based on the user’s preferences orschedule (e.g., reducing heating when no one is home). Security Management: If motion is detected when the system is armed, the alarm goes off, and a notification is sent to the user’s mobile device. Lighting Control: Based on time of day or detected presence, the lights can be automatically turned on/off or adjusted in brightness. Example Scenario: Situation: A family leaves for work and school in the morning. The motion sensors detect no movement in the house, so the system automatically turns off lights and reduces heating to save energy. The door/window sensors check if the house is secure (e.g., windows CHILL AVU MOWA are closed and doors are locked). The thermostat adjusts itself to an energy-saving mode, reducing heating or cooling while the family is away. In the evening, when the family is about to return, the thermostat and lights are automatically adjusted to ensure the house is comfortable when they arrive. If any unusual activity is detected (such as an intruder), the security system sends an immediate alert to the family’s mobile app. Benefits of Smart Home Automation Using IoT: Energy Efficiency: Smart homes optimize energy use, reducing costs by adjusting heating, cooling, and lighting based on occupancy and environmental conditions. Convenience: Users can remotely monitor and control home systems from anywhere in the world using mobile apps or voice assistants, reducing the need for manual intervention. Enhanced Security: IoT-based smart home systems enhance security by offering real-time monitoring through cameras, motion detectors, and smart locks. Alerts can be sent directly to the user’s phone in case of suspicious activity. Improved Comfort: Homes are automatically adjusted for the comfort of the occupants, such as adjusting lighting and temperature to preferred settings. Automation and Personalization: Systems learn the preferences of the occupants, allowing devices to work autonomously (e.g., turning on lights or adjusting the thermostat when people are near). UNIT – II 11) Explain the steps to interface any actuator to Arduino board? A: Interfacing an actuator to an Arduino board involves a series of steps to connect the actuator (e.g., motor, relay, or servo) and control it through Arduino’s digital or analog outputs. Below are the general steps to interface an actuator to an Arduino: 1. Choose the Actuator: First, identify the type of actuator you want to interface with the Arduino. Common actuators include: DC Motors: For rotary motion. Servo Motors: For precise angle control. Step Motors: For precise rotary motion with specific steps. Relays: To control high-voltage or high-current devices. Solenoids: For linear motion. Each actuator will have different wiring and control requirements. 2. Understand Actuator Control Requirements: Before interfacing an actuator, understand how it is controlled: DC Motors: Require a motor driver or transistor for controlling speed and direction. Servo Motors: Controlled via PWM signals (typically through one GPIO pin). Step Motors: Require a driver like the A4988 or DRV8825 to control steps. Relays: Activated by a HIGH or LOW signal (usually requires a transistor for switching). Solenoids: Usually require a transistor to switch the current on and off. 3. Gather Required Components For a successful interface, gather the necessary components: Arduino board (e.g., Arduino Uno, Mega, or Nano) Actuator (DC motor, servo, relay, etc.) Driver/Controller Circuit (for motors or high-power actuators) Power supply (suitable for the actuator, typically a separate supply for motors) Transistors (e.g., NPN transistor for switching) Flyback Diode (for inductive actuators like motors or solenoids) Resistors (for current-limiting) Wires and Breadboard for prototyping 4. Connect the Actuator to the Arduino For a DC Motor (using an H-Bridge motor driver): Step 1: Connect the motor driver to the DC motor. Connect the motor terminals to the output pins of the motor driver. Step 2: Connect the Arduino to the motor driver. Motor driver input pins (e.g., IN1, IN2 for direction control) should be connected to the Arduino’s digital pins (e.g., D3, D4). Step 3: Connect the motor driver’s enable pin (if applicable) to a PWM-capable pin (e.g., D5) to control the speed. Step 4: Connect the power supply to the motor driver to provide the necessary voltage and current for the motor. Step 5: GND connections: Connect the Arduino GND, motor driver GND, and power supply GND to a common ground. Figure 3-C:\Users\VARSHITH HOWDEKAR\Desktop\2111CS020621\Zeta\4-1\Pushpa.jpg For a Servo Motor: Step 1: Connect the servo control wire to a PWM-capable Arduino pin (e.g., D9). Step 2: Connect the servo’s power and ground wires to the 5V and GND pins on the Arduino (or an external 5V supply for larger servos). Step 3: Common Ground: Ensure all grounds (Arduino, servo, and external power supply) are connected. 5. Write the Arduino Code Once the hardware is connected, write the Arduino code to control the actuator. For a servo motor : #include Servo myServo; // Create a servo object void setup() { myServo.attach(9); // Attach the servo to pin 9 } void loop() { myServo.write(0); // Move servo to 0 degrees delay(1000); // Wait for 1 second myServo.write(90); // Move servo to 90 degrees delay(1000); // Wait for 1 second myServo.write(180); // Move servo to 180 degrees delay(1000); // Wait for 1 second } 6. Power the System Ensure the power supply matches the voltage and current requirements of the actuator: For low-power actuators (like small servos), the Arduino’s 5V pin may suffice. For high-power actuators (like motors or relays), use a separate power supply (e.g., 12V for motors), and ensure that the ground of the actuator's power supply is connected to the Arduino ground. 7. Test the System Upload the code to the Arduino and test the actuator’s functionality. Monitor the output to ensure proper operation: Check if the actuator responds to the Arduino’s control signals. Ensure the actuator is operating within its specified limits (e.g., motor speed, servo angles). By following these steps, you can successfully interface and control actuators with an Arduino board. The steps involve selecting the correct actuator, connecting it with the appropriate driver circuits, writing the control code, and ensuring the power supply meets the actuator’s requirements. With proper wiring and code, the Arduino can control actuators for a wide range of applications, from robotics to home automation systems. 12) Explain the functions of digital I/O, analog I/O & advanced I/O in Arduino programming, with an example. A: In Arduino programming, Digital I/O, Analog I/O, and Advanced I/O are the three main types of input/output operations used to interact with various devices and sensors. Here's a breakdown of their functions with examples: 1. Digital I/O Function: Digital I/O pins can either read or write binary values: HIGH (1) or LOW (0). These are used for simple on/off or true/false signals. Use Case: Controlling LEDs, reading button states, etc. Example: Blinking an LED const int ledPin = 13; // Pin connected to LED void setup() { pinMode(ledPin, OUTPUT); // Set pin as output } void loop() { digitalWrite(ledPin, HIGH); // Turn LED on delay(1000); // Wait for 1 second digitalWrite(ledPin, LOW); // Turn LED off delay(1000); // Wait for 1 second } 2. Analog I/O Function: Analog Input: Reads varying voltage levels (0-5V by default) and converts them to a range of values (0-1023 for 10-bit ADC). Analog Output: Generates a PWM signal that approximates analog voltages using analogWrite(). Use Case: Reading sensors (e.g., temperature or light sensors) and controlling devices (e.g., dimming LEDs, motor speed control). Example: Reading a potentiometer and dimming an LED const int potPin = A0; // Potentiometer connected to analog pin A0 const int ledPin = 9; // LED connected to PWM pin 9 void setup() { pinMode(ledPin, OUTPUT); } void loop() { int sensorValue = analogRead(potPin); // Read analog input (0-1023) int outputValue = map(sensorValue, 0, 1023, 0, 255); // Map to PWM range analogWrite(ledPin, outputValue); // Dim the LED } 3. Advanced I/O Function: Used for specialized purposes like communication protocols (SPI, I2C, UART), interrupts, and advanced timing. Use Case: Communicating with external modules like displays, sensors, or wireless modules. Example: Using I2C to interface with an LCD #include #include LiquidCrystal_I2C lcd(0x27, 16, 2); // I2C address 0x27, 16x2 LCD void setup() { lcd.init(); // Initialize LCD lcd.backlight(); // Turn on the backlight lcd.setCursor(0, 0); lcd.print("Hello, World!"); } void loop() { // Nothing to do here } Summary Digital I/O: Simple on/off operations (e.g., LEDs, buttons). Analog I/O: Reading sensor values and outputting PWM signals (e.g., dimming, motor speed). Advanced I/O: Specialized tasks like communication protocols (e.g., I2C, SPI) or handling complex devices. Each type serves a unique role in enabling Arduino to interface with the physical world effectively. 13) Explain the steps to interface a digital sensor to Arduino board with an example? A: Interfacing a digital sensor with an Arduino board involves several steps to ensure proper connection, programming, and functioning. Here’s a step-by-step guide: 1. Understand the Sensor Research the sensor's datasheet to understand: Pin configuration (e.g., power, ground, signal). Operating voltage (e.g., 3.3V or 5V). Communication protocol, if applicable (e.g., digital signal, I2C, SPI). 2. Gather the Required Components Arduino board (e.g., Uno, Nano, Mega). Digital sensor (e.g., IR sensor, ultrasonic sensor). Jumper wires. Breadboard (optional). Resistors, if needed. 3. Connect the Sensor to the Arduino Power the sensor: Connect the sensor's VCC pin to the Arduino's 5V or 3.3V pin (as per the sensor's requirement). Connect the sensor's GND pin to the Arduino's GND pin. Connect the signal pin: Connect the sensor's signal output to a digital input pin on the Arduino (e.g., pin 2). Additional connections: Some sensors may require pull-up/pull-down resistors or additional connections for proper functioning (e.g., ultrasonic sensors need a trigger and echo pin). Figure 4-C:\Users\VARSHITH HOWDEKAR\Desktop\2111CS020621\Zeta\4-1\Nani.jpg 4. Write the Arduino Code Initialize the sensor pin in the setup() function. Read the sensor's digital signal in the loop() function using digitalRead(). 5. Upload the Code to Arduino Open the Arduino IDE. Connect the Arduino to your computer via USB. Write or load the program. Select the appropriate board and port from the Tools menu. Click the Upload button. 6. Test the Setup Open the Serial Monitor (if needed) to observe the sensor output or test the sensor's functionality based on its intended purpose. 7. Debug (if necessary) Check wiring, code, or sensor placement if the sensor does not work as expected. Example: Interfacing a Digital IR Proximity Sensor Connections: VCC: Arduino 5V. GND: Arduino GND. OUT: Arduino digital pin 2. Code: const int sensorPin = 2; // Sensor output pin connected to digital pin 2 const int ledPin = 13; // Built-in LED for feedback void setup() { pinMode(sensorPin, INPUT); // Set sensor pin as input pinMode(ledPin, OUTPUT); // Set LED pin as output Serial.begin(9600); // Start Serial communication } void loop() { int sensorState = digitalRead(sensorPin); // Read sensor output Serial.println(sensorState); // Print state to Serial Monitor if (sensorState == HIGH) { // If object detected digitalWrite(ledPin, HIGH); // Turn LED on } else { digitalWrite(ledPin, LOW); // Turn LED off } delay(100); // Small delay for stability } 8. Observe Results The LED will turn on when the sensor detects an object. Serial Monitor will display the sensor state (e.g., 1 for detected, 0 for not detected). By following these steps, you can effectively interface and test any digital sensor with an Arduino board. 14) Explain about the Arduino programming control structures with an Example A: Arduino Programming Control Structures Conditional Statements: Make decisions. if: Executes if a condition is true. if-else: Executes one block if true, another if false. switch-case: Handles multiple conditions. Example: if (digitalRead(2) == HIGH) { digitalWrite(13, HIGH); } else { digitalWrite(13, LOW); } Loops: Repeat actions. for: Repeats a fixed number of times. while: Repeats as long as the condition is true. do-while: Executes at least once before checking. Example: for (int i = 0; i < 5; i++) { digitalWrite(13, HIGH); delay(500); digitalWrite(13, LOW); delay(500); } Control Transfer: break: Exits loops or switch cases. continue: Skips the current iteration. return: Exits a function. Example: for (int i = 0; i < 10; i++) { if (i == 5) break; Serial.println(i); } Control structures make programs logical and dynamic. 15) Write a program to implement push button controlled Led on Arduino Uno Board. A: const int buttonPin = 2; // Pin connected to the button const int ledPin = 13; // Pin connected to the LED int buttonState = 0; // Variable to store the button state void setup() { pinMode(buttonPin, INPUT); // Set the button pin as an input pinMode(ledPin, OUTPUT); // Set the LED pin as an output } void loop() { buttonState = digitalRead(buttonPin); // Read the button state if (buttonState == HIGH) { digitalWrite(ledPin, HIGH); // Turn on the LED } else { digitalWrite(ledPin, LOW); // Turn off the LED } } 16) Explain about the comparison operators with an example. A: Comparison Operators in Arduino Comparison operators are used to compare two values or expressions and return a Boolean result (true or false). These are commonly used in conditional statements and loops. Figure 5-C:\Users\VARSHITH HOWDEKAR\Desktop\2111CS020621\Zeta\4-1\GPT.jpg Example: Using Comparison Operators This example turns on an LED when a potentiometer's value is greater than a threshold. const int potPin = A0; // Potentiometer connected to analog pin A0 const int ledPin = 13; // LED connected to pin 13 const int threshold = 512; // Threshold value (half of 1023) void setup() { pinMode(ledPin, OUTPUT); Serial.begin(9600); } void loop() { int sensorValue = analogRead(potPin); // Read potentiometer value Serial.println(sensorValue); // Print the value if (sensorValue > threshold) { // Compare with threshold digitalWrite(ledPin, HIGH); // Turn LED on } else { digitalWrite(ledPin, LOW); // Turn LED off } delay(100); // Small delay for stability } Summary Purpose: Compare values and make decisions. Result: Returns true or false. Common Use: Conditional statements like if, while. 17) Discuss the steps involved in the process of interfacing a Bluetooth module to Arduino Uno. A: Interfacing a Bluetooth module (e.g., HC-05, HC-06) with an Arduino Uno involves the following steps: 1. Understand the Bluetooth Module Check the datasheet of the Bluetooth module to identify: Pins: VCC, GND, TX, RX. Default baud rate (commonly 9600). Communication protocol: UART (Serial Communication). 2. Gather Required Components CHILL AVU MOWA Arduino Uno. Bluetooth module (e.g., HC-05). Jumper wires. Resistors (if needed, to protect the module's RX pin from 5V logic). Optional: Breadboard. 3. Connect the Bluetooth Module Power the module: Connect the VCC pin of the module to the 5V pin of the Arduino. Connect the GND pin of the module to the GND pin of the Arduino. Connect the UART pins: Connect the TX pin of the Bluetooth module to the RX pin (pin 0) of the Arduino. Connect the RX pin of the Bluetooth module to the TX pin (pin 1) of the Arduino. Important: Use a voltage divider (two resistors) to reduce the Arduino's 5V signal to 3.3V for the module's RX pin if required. Figure 6-C:\Users\VARSHITH HOWDEKAR\Desktop\2111CS020621\Zeta\4-1\ZETA.jpg 4. Write and Upload Arduino Code Ensure the module is in communication mode (not AT mode). Load a simple program to send and receive data. Example Code: void setup() { Serial.begin(9600); // Initialize Serial communication at 9600 baud } void loop() { if (Serial.available()) { // Check if data is received char data = Serial.read(); // Read the received data Serial.println(data); // Echo the data back for confirmation } } Important: Disconnect the module's TX and RX pins while uploading the code to avoid interference with Serial communication. 5. Pair the Bluetooth Module Power the Arduino and Bluetooth module. Search for the module on a Bluetooth-enabled device (e.g., phone or laptop). Pair using the default password (e.g., 1234 or 0000). 6. Test the Setup Use a Bluetooth terminal app (e.g., "Serial Bluetooth Terminal") to connect to the module. Send and receive data to/from the Arduino via the Bluetooth connection. 7. Debugging Ensure baud rates match between the Arduino and Bluetooth module. Check wiring and power connections. Verify the module is in communication mode (not AT command mode). Summary Power the module. Connect TX-RX pins between the module and Arduino. Write, upload, and test the communication code. Pair and test using a Bluetooth-enabled device. 18) Write a program to interface a Humidity sensor to Arduino Uno Board with a neat circuit diagram. A: Program to Interface a Humidity Sensor to Arduino Uno This example demonstrates interfacing a DHT11/DHT22 humidity sensor with an Arduino Uno. These sensors provide temperature and humidity readings. Components: Arduino Uno. DHT11/DHT22 Sensor. Jumper Wires. Breadboard (optional). Connections: DHT11 Pinout: VCC: Connect to 5V on Arduino. GND: Connect to GND on Arduino. Data: Connect to a digital pin (e.g., pin 2). Program You need the DHT library to interface the sensor. Install it via Arduino IDE: Open Tools > Manage Libraries. Search for "DHT sensor library" by Adafruit and install it. Figure 7-C:\Users\VARSHITH HOWDEKAR\Desktop\2111CS020621\Zeta\4-1\Hey.jpg Code: #include // Define the pin and type of sensor #define DHTPIN 2 // Data pin connected to digital pin 2 #define DHTTYPE DHT11 // Change to DHT22 if using DHT22 DHT dht(DHTPIN, DHTTYPE); void setup() { Serial.begin(9600); // Start Serial communication dht.begin(); // Initialize the DHT sensor Serial.println("DHT11 Humidity and Temperature Sensor"); } void loop() { // Reading humidity and temperature float humidity = dht.readHumidity(); float temperature = dht.readTemperature(); // Check if readings failed if (isnan(humidity) || isnan(temperature)) { Serial.println("Failed to read from DHT sensor!"); return; } // Print values to Serial Monitor Serial.print("Humidity: "); Serial.print(humidity); Serial.print(" %\t"); Serial.print("Temperature: "); Serial.print(temperature); Serial.println(" °C"); delay(2000); // Wait 2 seconds between readings } 19) Write a program to interface an LED to Arduino board and it should be ON for 1Sec and OFF for 2Sec continuously, draw a neat circuit diagram. A: Program to Interface an LED to Arduino This program demonstrates how to interface an LED with an Arduino board. The LED will turn ON for 1 second and OFF for 2 seconds in a continuous loop. Components: Arduino Uno. LED. 220Ω Resistor. Jumper Wires. Breadboard (optional). Connections: LED Anode (longer leg) → Digital pin 13 via a 220Ω resistor. LED Cathode (shorter leg) → Arduino GND. Figure 8-C:\Users\VARSHITH HOWDEKAR\Desktop\2111CS020621\Zeta\4-1\Howdy.jpg Program const int ledPin = 13; // Pin connected to LED void setup() { pinMode(ledPin, OUTPUT); // Set LED pin as output } void loop() { digitalWrite(ledPin, HIGH); // Turn LED ON delay(1000); // Wait for 1 second digitalWrite(ledPin, LOW); // Turn LED OFF delay(2000); // Wait for 2 seconds } 20) Write a program to interface a Humidity sensor and its values will be displayed on LCD, with a neat circuit diagram A: Interfacing a Humidity Sensor with LCD to Display Readings This example demonstrates interfacing a DHT11/DHT22 humidity sensor and a 16x2 LCD with an Arduino Uno. The humidity and temperature readings will be displayed on the LCD. Circuit Diagram Description Components: Arduino Uno. DHT11/DHT22 Sensor. 16x2 LCD. 10kΩ Potentiometer (for LCD contrast control). Jumper Wires. Breadboard. Figure 9-C:\Users\VARSHITH HOWDEKAR\Desktop\2111CS020621\Zeta\4-1\Hope.jpg Connections: DHT11 Sensor: VCC → Arduino 5V. GND → Arduino GND. DATA → Arduino digital pin 2. 16x2 LCD: VSS → Arduino GND. VDD → Arduino 5V. VO → Middle pin of the potentiometer. RS → Arduino digital pin 7. RW → Arduino GND. E → Arduino digital pin 8. D4, D5, D6, D7 → Arduino digital pins 9, 10, 11, 12 respectively. A → Arduino 5V. K → Arduino GND. Program Before using the program, install the following libraries via Arduino IDE: DHT sensor library (by Adafruit). LiquidCrystal library (comes pre-installed with Arduino IDE). Code: #include #include // Define DHT sensor #define DHTPIN 2 // Data pin connected to pin 2 #define DHTTYPE DHT11 // Change to DHT22 if using DHT22 DHT dht(DHTPIN, DHTTYPE); // Initialize the LCD (RS, E, D4, D5, D6, D7) LiquidCrystal lcd(7, 8, 9, 10, 11, 12); void setup() { dht.begin(); // Initialize the DHT sensor lcd.begin(16, 2); // Initialize the LCD (16x2) lcd.print("Initializing"); // Display startup message delay(2000); lcd.clear(); } void loop() { // Read humidity and temperature float humidity = dht.readHumidity(); float temperature = dht.readTemperature(); // Check if readings are valid if (isnan(humidity) || isnan(temperature)) { lcd.clear(); lcd.print("Sensor Error"); delay(2000); return; } // Display readings on LCD lcd.clear(); lcd.setCursor(0, 0); // Set cursor to top left lcd.print("Humidity: "); lcd.print(humidity); lcd.print(" %"); lcd.setCursor(0, 1); // Set cursor to bottom left lcd.print("Temp: "); lcd.print(temperature); lcd.print(" C"); delay(2000); // Update every 2 seconds } UNIT-III 21) Explain RFID and NFC protocols in detail and mention its applications? A: RFID and NFC: A Comparative Overview RFID (Radio Frequency Identification) and NFC (Near-Field Communication) are wireless technologies used for contactless identification and data transfer. While they share similarities, they have distinct characteristics and applications. RFID (Radio Frequency Identification) RFID uses electromagnetic fields to identify and track tags attached to objects. The tag contains a microchip and an antenna, which receives and transmits data to an RFID reader. >Types of RFID: Passive RFID: Tags derive their power from the reader's electromagnetic field. They have a longer read range but are less secure. Active RFID: Tags have their own power source, allowing for longer read ranges and more complex data storage. >Applications of RFID: Supply Chain Management: Tracking products from manufacturing to delivery. Access Control: Controlling access to buildings, facilities, or restricted areas. Inventory Management: Monitoring stock levels and preventing theft. Animal Identification: Tracking livestock and pets. Electronic Tolling: Collecting tolls automatically. Payment Systems: Contactless payment cards. NFC (Near-Field Communication) NFC is a subset of RFID technology that operates at a shorter distance (typically a few centimeters) and requires both the tag and reader to be in close proximity. NFC is designed for simple, secure, and contactless data exchange. >Applications of NFC: Mobile Payments: Contactless payments using smartphones. Data Sharing: Sharing data between devices (e.g., exchanging contact information). Access Control: Controlling access to doors or devices. Ticketing: Electronic tickets for events or transportation. Loyalty Cards: Storing loyalty points and rewards. Feature RFID NFC Read Range Longer (up to several meters) Shorter (a few centimeters) Power Source Passive or Active Always Passive Data Primarily for identification and Exchange tracking For data exchange and payments Generally more secure due to shorter range and Security Varies encryption 22) Explain Bluetooth protocol in detail and mention its applications? A: Bluetooth Bluetooth is a wireless technology that lets devices like phones, tablets, and headphones connect to each other and share information without needing cables. Bluetooth simply follows the principle of transmitting and receiving data using radio waves. It can be paired with the other device which has also Bluetooth but it should be within the estimated communication range to connect. When two devices start to share data, they form a network called piconet which can further accommodate more than five devices. Bluetooth Protocol: Bluetooth is a wireless communication technology designed for short-range data exchange between devices. It operates in the 2.4 GHz ISM band and supports point-to-point and point-to-multipoint communication. The protocol is standardized under the IEEE 802.15.1 specification Key Features of Bluetooth Protocol: Range: Typically 10 meters (33 feet), extendable with higher power. Data Rate: Varies by version: Bluetooth 4.0: Up to 25 Mbps. Bluetooth 5.0 and 5.1: Up to 50 Mbps. Power Consumption: Low-power design, suitable for battery-operated devices. Security: Includes encryption, authentication, and key management.Types of Bluetooth Various types of Bluetooth are available in the market nowadays. Let us look at them. In-Car Headset: One can make calls from the car speaker system without the use of mobile phones. Stereo Headset: To listen to music in car or in music players at home. Webcam: One can link the camera with the help of Bluetooth with their laptop or phone. Bluetooth-Equipped Printer: The printer can be used when connected via Bluetooth with mobile phone or laptop. Bluetooth Global Positioning System (GPS): To use Global Positioning System (GPS) in cars, one can connect their phone with car system via Bluetooth to fetch the directions of the address. Applications of Bluetooth It can be used in wireless headsets, wireless PANs, and LANs. It can connect a digital camera wireless to a mobile phone. It can transfer data in terms of videos, songs, photographs, or files from one cell phone to another cell phone or computer. It is used in the sectors of Medical healthcare, sports and fitness, Military. Advantages It is a low-cost and easy-to-use device. It can also penetrate through walls. It creates an Ad-hoc connection immediately without any wires. It is used for voice and data transfer. Disadvantages It can be hacked and hence, less secure. It has a slow data transfer rate of 3 Mbps. Bluetooth communication does not support routing. 23) Differentiate CoAP and MQTT A: CoAP (Constrained Application Protocol) and MQTT (Message Queuing Telemetry Transport) are two popular protocols used in IoT applications. While both are designed for resource-constrained devices, they differ in their approach to communication. Feature CoAP MQTT Transport Layer UDP TCP Communication Model Request-Response Publish-Subscribe Security DTLS TLS Resource Discovery Built-in Not Built-in Reliability Lower, but can be enhanced with retransmissions Higher, with QoS levels Latency Lower Higher Bandwidth Efficiency Higher Lower 24) Explain Zigbee protocol in detail and mention the advantages over IEEE 802.15.4 ? A: Zigbee Protocol Zigbee is a wireless communication protocol specifically designed for low-power, low-data-rate wireless networks. It is built on top of the IEEE 802.15.4 physical layer (PHY) and media access control (MAC) layer. This means that Zigbee devices can communicate with other 802.15.4 devices, but Zigbee adds additional features and functionality to create a robust and flexible network. Key Features of Zigbee Low Power Consumption: Zigbee devices are designed to operate on battery power for extended periods, making them ideal for IoT applications. Mesh Networking: Zigbee devices can form self-organizing mesh networks, which provide increased reliability and range. Security: Zigbee offers various security features, including encryption and authentication, to protect sensitive data. Interoperability: Zigbee devices from different manufacturers can interoperate, ensuring flexibility and compatibility. Low Data Rate: Zigbee is suitable for applications that require low data rates, such as sensor networks and home automation. Advantages of Zigbee over IEEE 802.15.4 While IEEE 802.15.4 provides the foundation for Zigbee, Zigbee offers several advantages: Network Layer: Zigbee adds a network layer on top of 802.15.4, enabling features like routing, addressing, and security. Application Layer: Zigbee provides an application layer for specific use cases, such as home automation and industrial control. Self-Healing Networks: Zigbee networks can automatically reconfigure themselves in case of device failures or network changes, ensuring reliability. Security: Zigbee offers stronger security features than 802.15.4, protecting sensitive data and preventing unauthorized access. Interoperability: Zigbee devices from different manufacturers can interoperate, making it easier to create heterogeneous IoT networks. Applications of Zigbee Home Automation: Controlling lights, thermostats, and other home appliances. Industrial Automation: Monitoring and controlling industrial processes. Healthcare: Remote patient monitoring and medical device connectivity. Smart Cities: Infrastructure monitoring and traffic management. Agricultural Technology: Precision agriculture and crop monitoring. By leveraging the advantages of Zigbee, IoT devices can create efficient, reliable, and secure wireless networks, enabling a wide range of innovative applications. 25) Describe the features of 6LowPAN and mention routing considerations in it? A: 6LoWPAN (IPv6 over Low-Power Wireless Personal Area Networks) 6LoWPAN is a specification that enables IPv6 to run over low-power wireless networks, specifically IEEE 802.15.4 networks. This allows devices with limited resources, like those in IoT applications, to connect to the internet. Key Features of 6LoWPAN Header Compression: 6LoWPAN compresses IPv6 headers to reduce packet size, making them suitable for transmission over low-bandwidth links. Fragmentation and Reassembly: Large IPv6 packets are fragmented into smaller packets that can be transmitted over the 802.15.4 network. These fragments are reassembled at the destination. Neighbor Discovery: 6LoWPAN uses Neighbor Discovery Protocol (NDP) to discover and communicate with other nodes in the network. Security: 6LoWPAN supports various security mechanisms, including encryption and authentication, to protect data transmission. Energy Efficiency: 6LoWPAN devices are designed to be energy-efficient, with features like low-power modes and optimized communication protocols. Routing Considerations in 6LoWPAN Routing in 6LoWPAN is a critical aspect, as it determines how packets are forwarded from source to destination. Due to the constraints of low-power and low-bandwidth devices, routing protocols must be carefully designed to minimize overhead and energy consumption. Here are some key routing considerations in 6LoWPAN: Route Discovery: Proactive Routing: Routes are pre-computed and maintained, which can be efficient for static networks. Reactive Routing: Routes are discovered on-demand, which can be more energy-efficient for dynamic networks. Hybrid Routing: A combination of proactive and reactive routing can be used to balance efficiency and flexibility. Route Maintenance: Routing protocols must be able to detect and repair link failures to ensure network connectivity. Periodic route updates can be used to maintain route information and avoid stale routes. Energy Efficiency: Routing protocols should minimize the number of routing messages to reduce energy consumption. Sleep scheduling can be used to further reduce energy consumption by allowing nodes to enter low-power modes. Scalability: Routing protocols should be able to scale to large networks with many nodes. Hierarchical routing can be used to reduce the routing overhead in large networks. Security: Routing protocols must be secure to prevent attacks like routing loops and black hole attacks. Authentication and encryption can be used to protect routing messages. By carefully considering these factors, 6LoWPAN can enable efficient and reliable communication in low-power, low-bandwidth IoT networks 26) Explain in detail about Message Queue Telemetry Transport Protocol. A: Message Queue Telemetry Transport (MQTT) MQTT is a lightweight, publish-subscribe messaging protocol designed for constrained devices and low-bandwidth networks. It's particularly well-suited for Internet of Things (IoT) applications where devices need to communicate with each other and with cloud servers, often over unreliable networks. Key Features of MQTT Publish-Subscribe Model: Publisher: A device or application that sends messages to a specific topic. Subscriber: A device or application that receives messages from a specific topic. Broker: A central server that facilitates the communication between publishers and subscribers. Lightweight and Efficient: Designed for resource-constrained devices. Minimal overhead and low bandwidth usage. Reliable Message Delivery: Offers different Quality of Service (QoS) levels to ensure reliable message delivery: QoS 0: At most once delivery. QoS 1: At least once delivery. QoS 2: Exactly once delivery. Security: Supports various security mechanisms, including TLS/SSL for encrypted communication. Flexibility: Can be used in various IoT applications, from simple sensor networks to complex industrial systems. How MQTT Works: Connection: A device connects to an MQTT broker. Subscription: A device subscribes to specific topics of interest. Publication: A device publishes messages to specific topics. Message Delivery: The broker delivers messages to all subscribed devices. Use Cases of MQTT CHILL AVU MOWA Home Automation: Controlling smart devices like lights, thermostats, and security systems. Industrial IoT: Monitoring and controlling industrial processes, such as machine health and production line efficiency. Wearable Devices: Collecting and transmitting health data, such as heart rate and step count. Telematics: Tracking vehicle location and performance. Environmental Monitoring: Collecting data from sensors to monitor air quality, water quality, and weather conditions. Advantages of MQTT Low Bandwidth: Ideal for devices with limited bandwidth. Low Latency: Fast message delivery. Scalability: Can handle a large number of devices. Reliability: Ensures reliable message delivery. Flexibility: Supports various use cases and network topologies. Disadvantages of MQTT Complexity: Requires careful configuration and management of brokers and clients. Security Risks: If not properly configured, can be vulnerable to security attacks Figure 10-C:\Users\VARSHITH HOWDEKAR\Desktop\2111CS020621\Zeta\4-1\Rampage.jpg 27) Discuss in detail about XMPP protocol with a neat suitable diagram. A: Figure 11C:\Users\VARSHITH HOWDEKAR\Desktop\2111CS020621\Zeta\4-1\Turtles.jpg XMPP: A Versatile Communication Protocol XMPP (Extensible Messaging and Presence Protocol), formerly known as Jabber, is an open-source communication protocol for instant messaging, presence information, and other related services. It operates on a client-server architecture, allowing for real-time communication between different devices and applications. Key Features of XMPP Client-Server Architecture: XMPP operates on a decentralized network of servers. Clients connect to a server, which then routes messages to other clients, regardless of their location. XML-Based: XMPP uses XML to structure and exchange messages. This flexibility allows for the creation of custom extensions and protocols. Presence Information: XMPP provides real-time information about a user's availability status (online, away, busy, etc.). Message Delivery: XMPP supports various message types, including text, file transfers, and voice/video calls. Security: XMPP offers strong security mechanisms, including encryption and authentication, to protect user privacy and data integrity. Extensibility: XMPP's modular design allows for the development of custom extensions to add new features and functionalities. How XMPP Works: Client Connection: A client (e.g., a messaging app) connects to an XMPP server. Authentication: The client authenticates itself to the server using a username and password or other security mechanisms. Resource Binding: The client binds to a resource (e.g., a device or browser tab) to identify itself uniquely. Presence Information: The client sends presence information to the server, indicating its availability status. Message Exchange: Clients can send messages to other clients or to specific groups. Message Routing: The server routes messages to the appropriate recipients, regardless of their location or connection status. XMPP Use Cases XMPP has a wide range of applications, including: Instant Messaging: Real-time text-based communication between individuals or groups. Presence Information: Sharing real-time availability status. Voice and Video Calls: VoIP and video conferencing. File Transfer: Sharing files between users. Collaborative Tools: Real-time document editing and shared whiteboards. IoT Applications: Connecting devices and enabling remote control and monitoring. Advantages of XMPP Flexibility: XMPP's extensibility allows for customization and integration with other protocols. Security: Strong security features protect user privacy and data integrity. Scalability: XMPP can handle a large number of users and devices. Open Source: XMPP is open-source, enabling community-driven development and innovation. 28) Compare Zigbee and Zwave protocols and mention its applications. A: Zigbee and Z-Wave are two popular wireless communication protocols used in home automation and IoT devices. They both offer low-power, wireless connectivity for various smart devices, but they have distinct characteristics and applications. Feature Zigbee Z-Wave Frequency Band 2.4 GHz Sub-1 GHz 100 meters (328 Range feet) 50 meters (164 feet) Data Rate 250 kbps 100 kbps Network Topology Mesh networking Mesh networking Power Low power Low power Consumption consumption consumption Security AES-128 encryption AES-128 encryption Generally more Cost Generally cheaper expensive Applications Both Zigbee and Z-Wave are widely used in home automation, but they have specific strengths and weaknesses that make them suitable for different applications: Zigbee: ->Home Automation: Smart lighting control Smart thermostats Smart locks Smart sensors (temperature, humidity, motion) Industrial Applications: Wireless sensor networks Industrial automation Building automation Z-Wave: ->Home Automation: Smart home security systems Smart door locks Smart home appliances Smart irrigation systems ->Commercial Applications: Hotel room control Office building automation 29) Differentiate HTTP and MQTT protocols used in IOT. A: HTTP (Hypertext Transfer Protocol) and MQTT (Message Queue Telemetry Transport) are two popular protocols used in IoT applications. While both serve the purpose of data communication, they differ significantly in their approach and suitability for IoT scenarios. HTTP Communication Model: Request-Response Data Transfer: Text-based Connection: Stateless Security: SSL/TLS for HTTPS IoT Suitability: Well-suited for infrequent, large data transfers. Ideal for web-based interfaces and human-to-machine interactions. Less efficient for frequent, small data transfers. MQTT Communication Model: Publish-Subscribe Data Transfer: Binary Connection: Stateful Security: TLS IoT Suitability: Optimized for frequent, small data transfers. Ideal for machine-to-machine communication and sensor data. Efficient in low-bandwidth and unreliable network conditions. Feature HTTP MQTT Communication Model Request-Response Publish-Subscribe Data Transfer Text-based Binary Connection Stateless Stateful Security SSL/TLS TLS IoT Suitability Infrequent, large data transfers Frequent, small data transfers 30) Discuss in detail about two protocols used in low power applications. A: Two Protocols for Low-Power Applications In the realm of IoT and low-power applications, two protocols have emerged as popular choices: Zigbee and Bluetooth Low Energy (BLE). Let's delve into their characteristics and applications. Zigbee Zigbee is a wireless communication protocol designed for low-power, low-data-rate networks. It's particularly well-suited for home automation, industrial control, and sensor networks. Key Features: Mesh Networking: Zigbee devices can form self-organizing mesh networks, providing increased range and reliability. Low Power Consumption: Zigbee devices are designed to operate on battery power for extended periods. Security: Zigbee offers various security features, including encryption and authentication. Interoperability: Zigbee devices from different manufacturers can interoperate. Applications: Home automation: Controlling lights, thermostats, and other home appliances. Industrial automation: Monitoring and controlling industrial processes. Healthcare: Remote patient monitoring and medical device connectivity. Smart cities: Infrastructure monitoring and traffic management. Bluetooth Low Energy (BLE) BLE is a wireless technology designed for low-power consumption and short-range communication. It's commonly used in wearable devices, mobile accessories, and IoT devices. Key Features: Low Power Consumption: BLE devices can operate on battery power for extended periods. Short-Range Communication: BLE is suitable for short-range communication, typically within 100 meters. Fast Connection Establishment: BLE devices can establish connections quickly. Security: BLE offers various security features, including encryption and authentication. Applications: Wearable devices: Fitness trackers, smartwatches, and health monitors. Mobile accessories: Wireless headphones, keyboards, and mice. IoT devices: Smart locks, smart bulbs, and other IoT gadgets. UNIT – 1V 31) Define Machine-to- Machine and explain the working of M2M Gateway unit? A: Machine-to-Machine (M2M) Communication M2M refers to the direct communication between devices without human intervention. These devices exchange data and perform actions based on predefined rules or triggers. This technology enables the automation of various processes and systems, leading to increased efficiency and productivity. Working of an M2M Gateway An M2M gateway acts as a bridge between various devices and networks. It facilitates communication between these devices, ensuring seamless data exchange. Here's a breakdown of its functions: Device Connectivity: Wireless Protocols: The gateway supports various wireless protocols like Wi-Fi, Bluetooth, Zigbee, and cellular networks to connect with diverse devices. Wired Connections: It can also connect to devices through wired interfaces like Ethernet or RS-232. Data Aggregation and Processing: Data Collection: The gateway gathers data from multiple devices, including sensors, actuators, and other IoT devices. Data Processing: It processes the raw data, filters it, and applies necessary transformations. Data Formatting: The gateway formats the data into a suitable format for transmission over the network. Network Connectivity: Network Selection: It selects the appropriate network (cellular, Wi-Fi, Ethernet) based on the device's requirements and network availability. Network Protocols: The gateway uses protocols like MQTT, HTTP, or CoAP to communicate with the network. Security: It ensures secure data transmission by implementing encryption and authentication mechanisms. Cloud Integration: Data Transmission: The gateway sends the processed data to cloud platforms or servers. Cloud Services: It leverages cloud services like data storage, analytics, and machine learning to extract valuable insights from the data. Remote Management: Device Monitoring: The gateway allows remote monitoring of connected devices. Firmware Updates: It can update the firmware of connected devices remotely. Configuration Changes: Remote configuration changes can be made to the gateway and connected devices. By effectively performing these functions, M2M gateways enable a wide range of applications, including: Smart Homes: Controlling lights, thermostats, and security systems. Industrial Automation: Monitoring and controlling industrial processes. Remote Monitoring: Tracking assets and environmental conditions. Smart Cities: Managing traffic, energy consumption, and waste management. Healthcare: Remote patient monitoring and telemedicine. 32) Differentiate M2M and IOT, explain the communication in IOT and M2M? A: While M2M and IoT are often used interchangeably, they represent distinct concepts, albeit closely related. Let's delve into the differences and their communication mechanisms. M2M (Machine-to-Machine) Definition: M2M refers to the direct communication between devices without human intervention. Focus: Primarily on data exchange between devices for specific tasks. Communication: Typically point-to-point or simple network topologies. Applications: Industrial automation, remote monitoring, telemetry, and supply chain management. IoT (Internet of Things) Definition: IoT involves the interconnection of devices embedded with sensors, actuators, and software, enabling them to collect and exchange data over the internet. Focus: Broader scope, encompassing device connectivity, data analytics, and intelligent decision-making. Communication: Often involves cloud-based platforms for data storage, processing, and analysis. Applications: Smart homes, smart cities, wearables, healthcare, and agriculture. Communication in M2M and IoT M2M Communication: Point-to-Point: Direct communication between two devices, often using proprietary protocols or industry standards like Modbus or Zigbee. Simple Networks: Limited networks with a few devices, using protocols like Z-Wave or Wi-Fi. IoT Communication: Cloud-Based: Devices connect to the cloud via various protocols (Wi-Fi, cellular, LPWAN) to store, process, and analyze data. Internet Protocols: HTTP, MQTT, CoAP, and other internet protocols are commonly used. Edge Computing: Data processing and analysis can occur at the edge of the network, closer to the devices, reducing latency and bandwidth requirements. 33) Discuss Software Defined Networking and Network Function Virtualization? A: Software Defined Networking (SDN) SDN is a network architecture that separates the control plane from the data plane. Traditionally, network devices like routers and switches made routing decisions independently. In SDN, a centralized controller takes over these decisions, making the network more programmable and flexible. Key Components of SDN: Control Plane: A centralized controller that manages the network's overall behavior. Data Plane: Network devices (switches and routers) that handle the actual data forwarding. Southbound Interface: The interface between the controller and the data plane devices. Northbound Interface: The interface between the controller and network applications or management tools. Benefits of SDN: Programmability: SDN allows network administrators to programmatically configure and control the network. Flexibility: Network changes can be made quickly and easily without requiring physical hardware changes. Scalability: SDN can scale to support large and complex networks. Security: SDN can improve network security by implementing centralized security policies. Cost-Effectiveness: SDN can reduce operational costs by automating network management tasks. Network Function Virtualization (NFV): NFV is a technology that enables network functions, such as routers, firewalls, and load balancers, to be virtualized and deployed on standard IT hardware. This allows for greater flexibility, scalability, and cost-effectiveness. Key Components of NFV: Virtual Network Functions (VNFs): Software implementations of network functions. Virtualization Infrastructure: The underlying hardware and software platform that hosts VNFs. Management and Orchestration (MANO): Software that manages the lifecycle of VNFs, including deployment, configuration, and scaling. Benefits of NFV: Flexibility: NFV allows network operators to deploy and scale network functions quickly and easily. Cost-Effectiveness: NFV can reduce capital and operational costs by consolidating hardware and software resources. Agility: NFV enables rapid deployment of new services and features. Innovation: NFV fosters innovation by enabling the development of new network services and applications. 34) Explain the need the IOT System Management and mention the Characteristics of SNMP? A: The Need for IoT System Management As IoT systems become increasingly complex, with a vast number of interconnected devices, effective management becomes crucial. Without proper management, IoT deployments can face several challenges: Scalability: Managing a large number of devices can be overwhelming. Security: Protecting devices and data from cyber threats is essential. Reliability: Ensuring continuous operation and minimizing downtime is critical. Performance: Optimizing device performance and network efficiency is vital. Cost-Effectiveness: Efficient management can reduce operational costs. IoT System Management Key Features To address these challenges, IoT system management solutions offer several key features: Device Provisioning: Automating the process of adding new devices to the network. Configuration Management: Remotely configuring devices and updating settings. Firmware Updates: Distributing and deploying firmware updates to devices. Security Management: Implementing security policies, monitoring for threats, and responding to incidents. Monitoring and Alerting: Tracking device health, performance, and usage, and generating alerts for anomalies. Data Collection and Analysis: Gathering and analyzing data from devices to gain insights and optimize operations. Remote Control: Controlling devices remotely, such as restarting or resetting them. SNMP: A Protocol for Network Management Simple Network Management Protocol (SNMP) is a widely used protocol for managing network devices. It's particularly well-suited for IoT systems due to its simplicity and scalability. Characteristics of SNMP: Agent-Based Architecture: SNMP operates on a client-server model. Network devices, called agents, collect information and respond to queries from a network management station (NMS). Management Information Base (MIB): A hierarchical structure that defines the objects and their attributes that can be managed. Management Operations: SNMP defines five basic management operations: Get: Retrieves information from a device. Set: Modifies the value of an object on a device. GetNext: Retrieves the next object in a sequence. GetBulk: Retrieves multiple objects in a single request. Trap: Sends unsolicited notifications from a device to the NMS. Scalability: SNMP can manage large networks with thousands of devices. Flexibility: SNMP can be customized to meet the specific needs of different network environments. Security: SNMP supports various security mechanisms, including authentication and encryption. By leveraging SNMP, IoT system managers can efficiently monitor and control devices, ensuring optimal performance and security. 35) Describe IOT Systems Management with NETCONF? A: IoT System Management with NETCONF NETCONF (Network Configuration Protocol) is a powerful protocol designed for managing network devices. It provides a structured and secure way to remotely configure and monitor devices, making it well-suited for IoT system management. How NETCONF Works in IoT System Management: YANG Modeling: Data Modeling: YANG (Yang Modeling Language) is used to define the configuration and operational data of network devices. It provides a structured way to represent the device's state and the operations that can be performed on it. Data Model Creation: A YANG model is created to define the specific data elements and operations for IoT devices. This model serves as a blueprint for NETCONF interactions. NETCONF Client-Server Communication: NETCONF Client: A management application or tool that initiates NETCONF sessions with devices. NETCONF Server: The IoT device itself, which listens for NETCONF requests and responds accordingly. Secure Communication: NETCONF typically uses SSH for secure communication, ensuring data privacy and integrity. NETCONF Operations: Remote Configuration: The NETCONF client sends configuration commands to the device, such as setting up network parameters, enabling/disabling features, or updating firmware. Remote Monitoring: The client can retrieve real-time operational data from the device, such as sensor readings, device status, and error logs. Notification Mechanism: The device can send notifications to the client about events like alarms, faults, or changes in configuration. Benefits of Using NETCONF for IoT System Management: Standardized Approach: NETCONF provides a standardized way to manage network devices, simplifying integration and interoperability. Secure Communication: NETCONF leverages SSH for secure communication, protecting sensitive data. Flexible Configuration: YANG models allow for flexible and granular configuration of devices. Efficient Management: NETCONF enables automated configuration and monitoring, reducing manual effort. Scalability: NETCONF can manage a large number of devices efficiently. 36) Explain SDN in detail with a suitable block diagram. A: Software defined networking (SDN) is an approach to network management that enables dynamic, programmatically efficient network configuration to improve network performance and monitoring. It is a new way of managing computer networks that makes them easier and more flexible to control. In traditional networks, the hardware (like routers and switches) decides how data moves through the network, but SDN changes this by moving the decision-making to a central software system. This is done by separating the control plane (which decides where traffic is sent) from the data plane (which moves packets to the selected destination). In this article, we will discuss Software-Defined Networking in detail, including its workings, different models, and architecture. What is a Data Plane? All the activities involving and resulting from data packets sent by the end-user belong to this plane. Data Plane includes: Forwarding of packets. Segmentation and reassembly of data. Replication of packets for multicasting. What is a Control Plane? All activities necessary to perform data plane activities but do not CHILL AVU MOWA involve end-user data packets belong to this plane. In other words, this is the brain of the network. The activities of the control plane include: Making routing tables. Setting packet handling policies. Figure 12-C:\Users\VARSHITH HOWDEKAR\Desktop\2111CS020621\Zeta\4-1\Moto.jpg In Software-Defined Networking (SDN), the software that controls the network is separated from the hardware. SDN moves the part that decides where to send data (control plane) to software, while the part that actually forwards the data (data plane) stays in the hardware. This setup allows network administrators to manage and control the entire network using a single, unified interface. Instead of configuring each device individually, they can program and adjust the network from one central place. This makes managing the network much easier and more efficient. In a network, physical or virtual devices move data from one place to another. Sometimes, virtual switches, which can be part of either software or hardware, take over the jobs of physical switches. These virtual switches combine multiple functions into one smart switch. They check the data packets and their destinations to make sure everything is correct, then move the packets to where they need to go. 37) Discuss YANG model in NETCONF with necessary diagram. A: YANG (Yet Another Next Generation) is a data modeling language specifically designed for network configuration and state data. It provides a structured and human-readable way to define the configuration and operational state of network devices. NETCONF (Network Configuration Protocol) is a network management protocol that leverages YANG models to efficiently configure and manage network devices. It enables secure and programmatic control over network devices, making it a powerful tool for network automation and orchestration. The Synergy of YANG and NETCONF YANG and NETCONF work together to provide a robust and flexible framework for network management. Here's how: YANG Models Define Data Structures: YANG models define the hierarchical structure of network configuration and state data. They specify data types, constraints, and relationships between different data elements. NETCONF Uses YANG Models for Communication: NETCONF leverages YANG models to structure and format configuration and operational data. NETCONF operations, such as get, edit-config, and rpc, use YANG data structures to request and modify device configurations. For example, a NETCONF client can use a YANG model to construct an edit-config operation to modify the hostname and ip-address of a device. Figure 13-C:\Users\VARSHITH HOWDEKAR\Desktop\2111CS020621\Zeta\4-1\BE^.jpg 38) Explain in detail about session based network management protocol with suitable diagram. A: Session-Based Network Management Protocol (SNMPv3) SNMPv3 is the latest version of the Simple Network Management Protocol, designed to address the security vulnerabilities and scalability limitations of its predecessors (SNMPv1 and SNMPv2). It introduces a session-based approach to network management, enhancing security, authentication, and privacy. Figure 14-C:\Users\VARSHITH HOWDEKAR\Desktop\2111CS020621\Zeta\4-1\M2.jpg Key Features of SNMPv3: Security Model: User-Based Security Model (USM): Provides user-based authentication and privacy. View-Based Access Control Model (VACM): Controls access to specific MIB objects based on user roles and privileges. Community-Based Security Model (CSM): Provides a less secure, backward-compatible security model. Session-Based Communication: Establishes secure sessions between the NMS and managed devices. Encrypts and authenticates messages exchanged during the session. Enhanced Security Features: Supports strong cryptographic algorithms like AES and SHA. Provides message integrity and confidentiality. Protects against unauthorized access and data tampering. 39) Explain Simple Network Management Protocol in detail with a suitable block diagram. A: Simple Network Management Protocol (SNMP) is an Internet Standard protocol used for managing and monitoring network-connected devices in IP networks. SNMP is an application layer protocol that uses UDP port number 161/162.SNMP is used to monitor the network, detect network faults, and sometimes even to configure remote devices. Components of SNMP There are mainly three main components of SNMP SNMP Manager: It is a centralized system used to monitor the network. It is also known as a Network Management Station (NMS). A router that runs the SNMP server program is called an agent, while a host that runs the SNMP client program is called a manager. SNMP agent: It is a software management software module installed on a managed device. The manager accesses the values stored in the database, whereas the agent maintains the information in the database. To ascertain if the router is congested or not, for instance, a manager can examine the relevant variables that a router stores, such as the quantity of packets received and transmitted. Management Information Base: MIB consists of information on resources that are to be managed. This information is organized hierarchically. It consists of objects instances which are essentially variables. A MIB, or collection of all the objects under management by the manager, is unique to each agent. System, interface, address translation, IP, udp, and egp , icmp, tcp are the eight categories that make up MIB. The mib object is home to these groups. Figure 15-C:\Users\VARSHITH HOWDEKAR\Desktop\2111CS020621\Zeta\4-1\Coffee.jpg Characteristics of SNMP SNMP is used to monitor network It detects any network faults Can also be used to configure remote devices.