Inheritance Grade 12 GED Biology PDF
Document Details
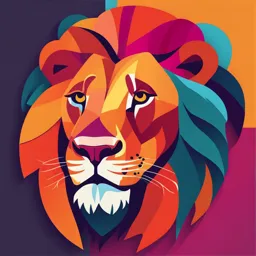
Uploaded by PrestigiousCotangent6986
Al Hikma Private School
Ms. Fathima
Tags
Related
- Cell Reproduction Grade 12 PDF
- Biology PPT Notes Ch 11 Meiosis & Sexual Reproduction PDF
- Lecture Slides: Genetics - Chapter 2 - Chromosomes and Cellular Reproduction - PDF
- Genetics Chapter 2 PDF - Study Guide
- Introduction to Genetics and Cellular Reproduction PDF
- Chapter 2: Introduction to Genetics and Cellular Reproduction PDF
Summary
This document covers inheritance concepts in biology, focusing on the structure of chromosomes, genes, alleles, haploid and diploid cells, and reduction division during meiosis. It provides definitions and explanations of these terms in the context of sexual reproduction, particularly highlighting the roles of gametes and zygotes.
Full Transcript
Inheritance Grade 12 GED Biology Ms. Fathima Reminder- The structure and features of chromosomes: The number of chromosomes per species is fixed. The shape of a chromosome is characteristic; they are of fixed length and with a narrow region, called the centromere, somewhere al...
Inheritance Grade 12 GED Biology Ms. Fathima Reminder- The structure and features of chromosomes: The number of chromosomes per species is fixed. The shape of a chromosome is characteristic; they are of fixed length and with a narrow region, called the centromere, somewhere along their length. The centromere is always in the same position on any given chromosome, and this position and the length of the chromosome is how it can be identified in photomicrographs. Chromosomes hold the hereditary factors, genes. A particular gene always occurs on the same chromosome in the same position. The position of a gene is called a locus. Each gene has two or more forms, called alleles. Gametes 2.1.1. Explain the meanings of and the terms gene, haploid (n) and reproduction diploid (2n). In most of your body cells there are two complete sets of chromosomes in the nucleus. One set Two sets L.O: Explain the meanings of the terms gene, haploid (n) and diploid (2n). One complete set of chromosomes contains one complete set of genes. Therefore, one complete set of instructions for making all the proteins that the organism needs. In humans, there are 23 chromosomes in one complete set. Examples of haploid cells are sex cells, also known as gametes A cell that has ONE complete set of chromosomes is said to be haploid. L.O: Explain the meanings of the terms gene, haploid (n) and diploid (2n). During sexual reproduction, each gamete contributes one set of chromosomes to form the zygote. You, for example, began your life when a set of chromosomes from your father's sperm was joined with a set of chromosomes from your mother's egg cell, as the nuclei of these two gametes fused. It is therefore important that gametes have only one set of chromosomes. A cell that has two complete sets of chromosomes is said to be diploid. L.O: Explain the meanings of the terms gene, haploid (n) and diploid (2n). There are therefore 46 chromosomes in a diploid cell. L.O: Explain the meanings of the terms gene, haploid (n) and diploid (2n). Haploid and diploid cells Diploid cells contain two sets of chromosomes. Most cells in organisms are diploid This is represented as 2n, where n = the number of chromosomes in one set of chromosomes. Haploid cells contain only one set of chromosomes. This is represented as n. Not all cells are diploid. As we shall see, gametes have only one set of chromosomes. In humans, therefore, normal body cells are diploid (2n), with 46 chromosomes, and gametes are haploid (n), with 23 chromosomes. L.O: Explain the meanings of the terms gene, haploid (n) and diploid (2n). L.O: Explain the meanings of the terms gene, haploid (n) and diploid (2n). Gametes 2.1.2. Explain what is meant by and homologous pairs of reproduction chromosomes. The picture shows these two sets of chromosomes, taken from a human cell. Their individual photographs have been moved around so that the chromosomes are arranged in their matching pairs. Each chromosome has a number. The chromosomes with the same number contain the same genes in the same positions. These are said to be homologous chromosomes. L.O: Explain what is meant by homologous pairs of chromosomes. Chromosomes have a characteristic shape They have a fixed length The position of the centromere is in a particular location L.O: Explain what is meant by homologous pairs of chromosomes. Homologous chromosomes Carry the same genes in the same positions (locus) Are the same shape They have the same length The position of the centromere are in the same place along the chromosome L.O: Explain what is meant by homologous pairs of chromosomes. L.O: Explain what is meant by homologous pairs of chromosomes. L.O: Explain what is meant by homologous pairs of chromosomes. L.O: Explain what is meant by homologous pairs of chromosomes. Gametes 2.1.3. Explain the need for a and reduction division during meiosis in reproduction the production of gametes. Meiosis is a type of nuclear (nucleus) division that produces haploid cells from a diploid cell. It is used in the production of gametes in animals and plants. L.O: Explain the need for a reduction division during meiosis in the production of gametes. Meiosis is type of nuclear division that results in daughter nuclei each containing half the number of chromosomes of the parent cell. L.O: Explain the need for a reduction division during meiosis in the production of gametes. In sexual reproduction, two sex cells (called gametes) fuse; this is fertilisation. The resulting cell is called the zygote, and it will grow and develop into a new individual. Because two nuclei fuse when sexual reproduction occurs, the chromosome number is doubled at that time. For example, human gametes (each egg cell and sperm) have just 23 chromosomes each. When the male and female gametes fuse, the full component of 46 chromosomes is restored. L.O: Explain the need for a reduction division during meiosis in the production of gametes. The doubling of the number chromosome each time sexual reproduction occurs (every generation) can be avoided. It is avoided by a nuclear division that halves the chromosome number. This reductive (reduces) division is called meiosis. The sex cells formed following meiosis contain a single set of chromosomes, a condition known as haploid. When haploid gametes fuse the resulting cell has the diploid condition again. L.O: Explain the need for a reduction division during meiosis in the production of gametes. Homologous chromosomes are a pair of chromosomes in a diploid cell that have the same structure as each other, with the same genes (but not necessarily the same alleles of those genes) at the same loci, and that pair together to form a bivalent during the first division of meiosis. A gene is a length of DNA that codes for a particular protein or polypeptide. An allele is a particular variety of a gene. A locus is the position at which a particular gene is found on a particular chromosome; the same gene is always found at the same locus. A zygote is a diploid cell resulting from the fusion of 2 haploid gametes. A diploid cell is one that possesses two complete sets of chromosomes; the abbreviation for diploid is 2n. A haploid cell is one that possesses one complete set of chromosomes; the abbreviation for haploid is n. 2.1.4. Describe the behaviour of Gametes chromosomes in plant and animal and cells during meiosis and the reproduction associated behaviour of the nuclear envelope, the cell surface membrane, and the spindle Meiosis » A few cells in the human body – some of those in the testes and ovaries – are able to divide by a type of division called meiosis. » Meiosis involves two divisions (not one as in mitosis), so four daughter cells are formed. » Meiosis produces four new cells with: – only half the number of chromosomes as the parent cell – different combinations of alleles from each other and from the parent cell » In animals and flowering plants, meiosis produces gametes. » In a human body, cells are diploid (2n), containing two complete sets of chromosomes. » Meiosis produces gametes that are haploid (n), containing one complete set of chromosomes. » This is why meiosis is known as reduction division and is necessary so that the cell formed at fertilisation (the zygote) is diploid. L.O: Describe the behaviour of chromosomes in plant and animal cells during meiosis. Meiosis Before meiosis begins, DNA replication takes place exactly as it does before mitosis DNA replication takes place during interphase, so that there are two identical copies of each DNA molecule in the nucleus. The phase within interphase in which it occurs is called S phase. Each original chromosome is made up of one DNA molecule, so after replication is complete each chromosome is made of two identical DNA molecules. L.O: Describe the behaviour of chromosomes in plant and animal cells during meiosis. Meiosis L.O: Describe the behaviour of chromosomes in plant and animal cells during meiosis. Meiosis L.O: Describe the behaviour of chromosomes in plant and animal cells during meiosis. Meiosis We count chromosomes by the number of centromeres present. So when the 46 chromosomes duplicate during interphase and the amount of DNA in the cell doubles there are still only 46 chromosomes present because there are still only 46 centromeres present. However, there are now 92 chromatids, which are strands of replicated chromosomes. L.O: Describe the behaviour of chromosomes in plant and animal cells during meiosis. Meiosis P Prophase Preparing M Metaphase Middle A Anaphase Away T Telophase Two L.O: Describe the behaviour of chromosomes in plant and animal cells during meiosis. Meiosis L.O: Describe the behaviour of chromosomes in plant and animal cells during meiosis. Meiosis L.O: Describe the behaviour of chromosomes in plant and animal cells during meiosis. Meiosis L.O: Describe the behaviour of chromosomes in plant and animal cells during meiosis. Meiosis L.O: Describe the behaviour of chromosomes in plant and animal cells during meiosis. Meiosis L.O: Describe the behaviour of chromosomes in plant and animal cells during meiosis. Meiosis L.O: Describe the behaviour of chromosomes in plant and animal cells during meiosis. Meiosis L.O: Describe the behaviour of chromosomes in plant and animal cells during meiosis. Meiosis L.O: Describe the behaviour of chromosomes in plant and animal cells during meiosis. Meiosis L.O: Describe the behaviour of chromosomes in plant and animal cells during meiosis. Meiosis L.O: Describe the behaviour of chromosomes in plant and animal cells during meiosis. Meiosis L.O: Describe the behaviour of chromosomes in plant and animal cells during meiosis. Meiosis L.O: Describe the behaviour of chromosomes in plant and animal cells during meiosis. Meiosis L.O: Describe the behaviour of chromosomes in plant and animal cells during meiosis. Meiosis L.O: Describe the behaviour of chromosomes in plant and animal cells during meiosis. Meiosis L.O: Describe the behaviour of chromosomes in plant and animal cells during meiosis. Meiosis Prophase I DNA condenses and becomes visible as chromosomes DNA replication has already occurred so each chromosome consists of two sister chromatids joined together by a centromere The chromosomes are arranged side by side in homologous pairs A pair of homologous chromosomes is called a bivalent As the homologous chromosomes are very close together the crossing over of non-sister chromatids may occur. The point at which the crossing over occurs is called the chiasma (chiasmata; plural) In this stage centrioles migrate to opposite poles and the spindle is formed The nuclear envelope breaks down and the nucleolus disintegrates L.O: Describe the behaviour of chromosomes in plant and animal cells during meiosis. Meiosis Metaphase I The bivalents line up along the equator of the spindle, with the spindle fibres attached to the centromeres Anaphase I The homologous pairs of chromosomes are separated as microtubules pull whole chromosomes to opposite ends of the spindle The centromeres do not divide L.O: Describe the behaviour of chromosomes in plant and animal cells during meiosis. Meiosis Telophase I The chromosomes arrive at opposite poles Spindle fibres start to break down Nuclear envelopes form around the two groups of chromosomes and nucleoli reform Some plant cells go straight into meiosis II without reformation of the nucleus in telophase I L.O: Describe the behaviour of chromosomes in plant and animal cells during meiosis. Meiosis Cytokinesis This is when the division of the cytoplasm occurs Cell organelles also get distributed between the two developing cells The end product of cytokinesis in meiosis I: two haploid cells These cells are haploid as they contain half the number of centromeres L.O: Describe the behaviour of chromosomes in plant and animal cells during meiosis. Meiosis Cytokinesis In animal cells: The cell surface membrane pinches inwards creating a cleavage furrow in the middle of the cell which contracts, dividing the cytoplasm in half L.O: Describe the behaviour of chromosomes in plant and animal cells during meiosis. Meiosis Cytokinesis In plant cells: Vesicles from the Golgi apparatus gather along the equator of the spindle (the cell plate). The vesicles merge with each other to form the new cell surface membrane. Layers of cellulose are laid down to form the primary and secondary walls of the cell L.O: Describe the behaviour of chromosomes in plant and animal cells during meiosis. Meiosis Prophase II The nuclear envelope breaks down and chromosomes condense A spindle forms at a right angle to the old one Metaphase II Chromosomes line up in a single file along the equator of the spindle L.O: Describe the behaviour of chromosomes in plant and animal cells during meiosis. Meiosis Anaphase II Centromeres divide and individual chromatids are pulled to opposite poles This creates four groups of chromosomes that have half the number of chromosomes compared to the original parent cell L.O: Describe the behaviour of chromosomes in plant and animal cells during meiosis. Meiosis Telophase II Nuclear membranes form around each group of chromosomes Cytokinesis Cytoplasm divides as new cell surface membranes are formed creating four haploid cells The cells still contain the same number of centromeres as they did at the start of meiosis I but they now only have half the number of chromosomes (previously chromatids) L.O: Describe the behaviour of chromosomes in plant and animal cells during meiosis. Meiosis L.O: Describe the behaviour of chromosomes in plant and animal cells during meiosis. Meiosis L.O: Describe the behaviour of chromosomes in plant and animal cells during meiosis. Meiosis L.O: Describe the behaviour of chromosomes in plant and animal cells during meiosis. Meiosis DNA Replication L.O: Describe the behaviour of chromosomes in plant and animal cells during meiosis. Meiosis L.O: Describe the behaviour of chromosomes in plant and animal cells during meiosis. Meiosis L.O: Describe the behaviour of chromosomes in plant and animal cells during meiosis. Meiosis L.O: Describe the behaviour of chromosomes in plant and animal cells during meiosis. Meiosis L.O: Describe the behaviour of chromosomes in plant and animal cells during meiosis. Meiosis L.O: Describe the behaviour of chromosomes in plant and animal cells during meiosis. Meiosis L.O: Describe the behaviour of chromosomes in plant and animal cells during meiosis. Meiosis L.O: Describe the behaviour of chromosomes in plant and animal cells during meiosis. Meiosis L.O: Describe the behaviour of chromosomes in plant and animal cells during meiosis. Meiosis L.O: Describe the behaviour of chromosomes in plant and animal cells during meiosis. Meiosis L.O: Describe the behaviour of chromosomes in plant and animal cells during meiosis. Gametes 2.1.5. Interpret photomicrographs and and diagrams of cells in different reproduction stages of meiosis and identify the main stages of meiosis. L.O: Interpret photomicrographs and diagrams of cells in different stages of meiosis and identify the main stages L.O: Interpret photomicrographs and diagrams of cells in different stages of meiosis and identify the main stages L.O: Interpret photomicrographs and diagrams of cells in different stages of meiosis and identify the main stages L.O: Interpret photomicrographs and diagrams of cells in different stages of meiosis and identify the main stages L.O: Interpret photomicrographs and diagrams of cells in different stages of meiosis and identify the main stages L.O: Interpret photomicrographs and diagrams of cells in different stages of meiosis and identify the main stages L.O: Interpret photomicrographs and diagrams of cells in different stages of meiosis and identify the main stages L.O: Interpret photomicrographs and diagrams of cells in different stages of meiosis and identify the main stages L.O: Interpret photomicrographs and diagrams of cells in different stages of meiosis and identify the main stages L.O: Interpret photomicrographs and diagrams of cells in different stages of meiosis and identify the main stages L.O: Interpret photomicrographs and diagrams of cells in different stages of meiosis and identify the main stages L.O: Interpret photomicrographs and diagrams of cells in different stages of meiosis and identify the main stages Keeping count of chromosomes Mitosis Before interphase After interphase (DNA Replication) During mitosis (* anaphase) After Mitosis Chromosomes 46 46 46 46 Chromatids 46 92 92 46 Meiosis Before interphase After interphase (DNA Replication) After meiosis I After meiosis II Chromosomes 46 46 23 23 Chromatids 46 92 46 23 2.2.1. Explain that crossing over and Production random orientation (independent of assortment) of pairs of homologous genetic chromosomes and sister chromatids during meiosis variation produces genetically different gametes, with reference to alleles, linkage, and loci. Genetics Meiosis and genetic variation There are differences in the genetic information carried by different gametes. This variation arises in meiosis and is highly significant for the organism. The reason why the four haploid cells produced by meiosis differ genetically from each other is because of two important features of meiosis. The crossing over of segments of individual maternal and paternal homologous chromosomes. The independent assortment of maternal and paternal homologous chromosomes. Crossing Over Each chromosome in a homologous pair carries genes for the same characteristics at the same locus. The alleles of the genes on the two chromosomes may be the same or different. During prophase of meiosis I, as the two homologous chromosomes lie side by side, their chromatids form links called chiasmata (singular: chiasma) with each other. When they move apart, a piece of chromatid from one chromosome may swap places with a piece from the other chromosome. This is called crossing over. It results in each chromosome having different combinations of alleles than it did before L.O: Explain that crossing over and random orientation (independent assortment) of pairs of homologous chromosomes and sister chromatids during meiosis produces genetically different gametes Crossing Over Each chromosome in a homologous pair carries genes for the same characteristics at the same locus. L.O: Explain that crossing over and random orientation (independent assortment) of pairs of homologous chromosomes and sister chromatids during meiosis produces genetically different gametes Crossing Over L.O: Explain that crossing over and random orientation (independent assortment) of pairs of homologous chromosomes and sister chromatids during meiosis produces genetically different gametes  The gene for antenna length has two alleles. Allele E gives long antennae allele e gives short ones (a condition called Crossing Over aristopedia).  The gene for eye colour also has two alleles, A which gives red eyes a which gives brown eyes In some of the cells undergoing But in some of the cells, crossing meiosis, crossing over between over switches the positions of the these two gene loci does not alleles. Now the alleles on one happen. The alleles stay on their chromosome are E and a, and on own chromosome. the other they are e and A. This means that there are four different kinds of gamete: alleles E and A, coding for long alleles E and a, coding for long antennae and red eyes antennae and brown eyes alleles e and a, coding for short alleles e and A, coding for short antennae and brown eyes antennae and red eyes L.O: Explain that crossing over and random orientation (independent assortment) of pairs of homologous chromosomes and sister chromatids during meiosis produces genetically different gametes Crossing Over L.O: Explain that crossing over and random orientation (independent assortment) of pairs of homologous chromosomes and sister chromatids during meiosis produces genetically different gametes Crossing Over L.O: Explain that crossing over and random orientation (independent assortment) of pairs of homologous chromosomes and sister chromatids during meiosis produces genetically different gametes Crossing Over L.O: Explain that crossing over and random orientation (independent assortment) of pairs of homologous chromosomes and sister chromatids during meiosis produces genetically different gametes Crossing Over L.O: Explain that crossing over and random orientation (independent assortment) of pairs of homologous chromosomes and sister chromatids during meiosis produces genetically different gametes Independent Assortment The production of different combinations of alleles in daughter cells, as a result of the random alignment of bivalents on the equator of the spindle during metaphase I of meiosis During the first division of meiosis, the pairs of homologous chromosomes line up on the equator before being pulled to opposite ends of the cell. Each pair behaves independently from every other pair, so there are many different combinations that can end up together. L.O: Explain that crossing over and random orientation (independent assortment) of pairs of homologous chromosomes and sister chromatids during meiosis produces genetically different gametes Independent Assortment Each new group, therefore, contains a different mixture of maternal and paternal chromosomes. Similarly, at meiosis II, the direction in which each sister chromatid from a pair is pulled is completely random. Figure 16.9 shows the different combinations you can get with just two pairs of chromosomes. In a human there are 23 pairs, so there is a huge number of different possibilities. L.O: Explain that crossing over and random orientation (independent assortment) of pairs of homologous chromosomes and sister chromatids during meiosis produces genetically different gametes Independent Assortment L.O: Explain that crossing over and random orientation (independent assortment) of pairs of homologous chromosomes and sister chromatids during meiosis produces genetically different gametes Independent Assortment L.O: Explain that crossing over and random orientation (independent assortment) of pairs of homologous chromosomes and sister chromatids during meiosis produces genetically different gametes Independent Assortment L.O: Explain that crossing over and random orientation (independent assortment) of pairs of homologous chromosomes and sister chromatids during meiosis produces genetically different gametes Independent Assortment L.O: Explain that crossing over and random orientation (independent assortment) of pairs of homologous chromosomes and sister chromatids during meiosis produces genetically different gametes Genetic variation arising from meiosis 1. Genetic variation increases the chances of survival of the species in a changing environment. 2. Variation provides the basis for natural selection, a process where, over time, individuals with heritable traits more suitable for the environment are more likely to survive and reproduce, passing on their favourable genes to their offspring. 3. In a changing environment, a larger gene pool (due to genetic variation) is more likely to contain genes that express traits more suitable for the new environment. The species have a higher chance of becoming adapted instead of becoming extinct. L.O: Explain that crossing over and random orientation (independent assortment) of pairs of homologous chromosomes and sister chromatids during meiosis produces genetically different gametes 4. Genetic variation arises through 3 processes: (a) Independent assortment of chromosomes during metaphase I of meiosis. Independent assortment results in gametes with a random mixture of maternal and paternal chromosomes. (b) Crossing-over between homologous chromosomes during prophase I of meiosis. Crossing-over results in genetic recombination, producing chromosomes that have a mixture of maternal and paternal DNA. (c) Random fertilisation of gametes. Each gamete has a unique set of 23 chromosomes due to independent assortment and crossing-over in meiosis. Any one male gamete representing one out of the many different possible gene combinations, can fertilise an ovum, also representing one out of the many different possible gene combinations. This will produce variation due to the many different combinations of genes from the male and female gamete. L.O: Explain that crossing over and random orientation (independent assortment) of pairs of homologous chromosomes and sister chromatids during meiosis produces genetically different gametes Production 2.2.2. Explain that the random of fusion of gametes at fertilization genetic produces genetically different variation individuals. Genetic variation arising from random fertilisation. The two sources of genetic variation you have looked at so far – crossing over and independent assortment – both result in different combinations of alleles in gametes. If you assume that any male gamete can fuse with any female gamete, each with these potentially large amounts of variation between them, you can see that the new individuals produced as a result of sexual reproduction have almost no chance of being genetically identical. They will inevitably have different combinations of alleles. L.O: Explain that the random fusion of gametes at fertilization produces genetically different individuals. L.O: Explain that crossing over and random orientation (independent assortment) of pairs of homologous chromosomes and sister chromatids during meiosis produces genetically different gametes 2.3.1. Explain the meaning of the term's dominant, recessive, Genetics codominant, phenotype, genotype, homozygous and heterozygous. Key Words in Genetics Genes & alleles The DNA contained within chromosomes is essential for cell survival Every chromosome consists of a long DNA molecule which codes for several different proteins A length of DNA that codes for a single polypeptide or protein is called a gene The position of a gene on a chromosome is its locus (plural: loci) Each gene can exist in two or more different forms called alleles Different alleles of a gene have slightly different nucleotide sequences but they still occupy the same position (locus) on the chromosome Example of alleles One of the genes for coat colour in horses is Agouti This gene for coat colour is found on the same position on the same chromosome for all horses Hypothetically there are two different forms (alleles) of that gene found in horses: A and a Each allele can produce a different coat colour: Allele A → black coat Allele a → chestnut coat Genotype & phenotype The chromosomes of eukaryotic cells occur in homologous pairs (there are two copies of each chromosome) As a result cells have two copies of every gene As there are two copies of a gene present in an individual that means there can be different allele combinations within an individual The genotype of an organism refers to the alleles of a gene possessed by that individual. The different alleles can be represented by letters Genotype & phenotype When the two allele copies are identical in an individual they are said to be homozygous When the two allele copies are different in an individual they are said to be heterozygous The genotype of an individual affects their phenotype A phenotype is the observable characteristics of an organism Dominant and Recessive Not all alleles affect the phenotype in the same way Some alleles are dominant: they are always expressed in the phenotype This means they are expressed in both heterozygous and homozygous individuals Dominant genes are usually represented by a capital letter. E.g. A Others are recessive: they are only expressed in the phenotype if no dominant allele is present This means that it is only expressed when present in a homozygous individual Recessive genes are usually represented by a small letter. E.g. a Example of genotype & phenotype Every horse has two copies of the coat colour gene in all of their cells A horse that has two black coat alleles A has the genotype AA and is homozygous dominant. The phenotype of this horse would be a black coat In contrast a horse that has one black coat allele A and one chestnut coat allele a would have the genotype Aa and is heterozygous. The phenotype of this horse would be a black coat A horse that has two chestnut coat alleles a has the genotype aa and is homozygous recessive. The phenotype of this horse would be a chestnut coat. Example of dominance If for horses the allele A for a black coat is dominant and the allele a for a chestnut coat is recessive the following genotypes and phenotypes occur: Genotype AA → black coat Genotype Aa → black coat Genotype aa → chestnut coat In the diagram, the parent pea plants (P generation) are either: homozygous tall homozygous dwarf heterozygous tall Codominance Sometimes both alleles can be expressed in the phenotype at the same time This is known as codominance Even when an individual is heterozygous they will express both alleles in their phenotype When writing the genotype for codominance the gene is symbolised as the capital letter and the alleles are represented by different superscript letters, for example IA Example of codominance A good example of codominance can be seen in human blood types The gene for blood types is represented in the genotype by I and the three alleles for human blood types are represented by A, B and O Allele A results in blood type A (IAIA or IAIO) and allele B results in blood type B (IBIB or IBIO) If both allele A and allele B are present in a heterozygous individual they will have blood type AB (IAIB) Blood type O (IOIO) is recessive to both group A and group B alleles Genotype Phenotype A A II A B II A O II B B II B O II O O I I A O B O II x II Another example of codominance is the case of the haemoglobin -polypeptide gene, where: the phenotype of a person who has the genotype HbA HbA is unaffected by sickle cell disorder, the phenotype of a person who has the genotype HbA HbS is the less severe sickle cell trait and the phenotype of a person who has the genotype HbS HbS is the more severe sickle cell anaemia. HbA HbS x HbA HbS Snapdragons, or antirrhinums, are plants with colourful flowers. The allele for white petals, CW, is codominant with the allele for red petals, CR. Heterozygous plants produce pink flowers. A pink snapdragon was pollinated with a white snapdragon. Give the predicted phenotype and genotype ratios for the next generation. Parents are CRCW and CWCW; offspring genotypes: CRCW and CWCW in a 1:1 ratio; offspring phenotypes: pink and white in a 1:1 ratio. Inheritance 2.4.1. Explain the meaning of the and terms test cross, F1, F2 and sex genetic diagrams linkage. F1 and F2 When a homozygous dominant individual is crossed with a homozygous recessive individual the offspring are called the F1 generation All of the F1 generation are heterozygous If two individuals from the F1 generation are then crossed, the offspring they produce are called the F2 generation Test crosses A test cross can be used to try and deduce the genotype of an unknown individual that is expressing a dominant phenotype The individual in question is crossed with an individual that is expressing the recessive phenotype The resulting phenotypes of the offspring provide sufficient information to suggest the genotype of the unknown individual If there are any offspring expressing the recessive phenotype then the unknown individual must have a heterozygous genotype A carrier for a disease has the allele for a trait but doesn’t usually show symptoms. They will be heterozygous (e.g. Aa or Hh) and can pass the allele for the disorder to their offspring. If the offspring is homozygous-recessive (aa or hh) then the offspring will be infected. Sex linkage: There are two sex chromosomes: X and Y Women have two copies of the X chromosome (XX) whereas men have one X chromosome and one shorter Y chromosome (XY) Some genes are found on a region of a sex chromosome that is not present on the other sex chromosome As the inheritance of these genes is dependent on the sex of the individual they are called sex-linked genes Most often sex-linked genes are found on the longer X chromsome Haemophilia is well known example of a sex-linked disease Sex-linked genes are represented in the genotype by writing the alleles as superscript next to the sex chromosome. For example a particular gene that is found only on the X chromosome has two alleles G and g. The genotype of a heterozygous female would be written as XGXg. A males genotype would be written as XGY Haemophilia is a disease that affects the blood’s ability to clot. Haemophilia can lead to slow and persistent internal bleeding, particularly in joints, and can be lethal if left untreated. Because many individuals with this disease do not survive until reproductive age, it is relatively rare. The overwhelming majority of its sufferers today are male; only a small percentage are female. This is in part due to the fact that many haemophiliac females die from extreme blood loss when menstruation begins. Haemophilia can be caused by a number of things, but one is a recessive allele on the X chromosome which codes for a faulty protein involved in the clotting process. When a carrier female and a healthy male have offspring, 25% will be haemophilic males, as predicted by the diagram below. While males can inherit haemophilia from their mothers, they cannot inherit it from their fathers. 25% of the offspring will also be carriers XᴴXʰ. The following diagram shows a cross between a non-carrier male and a carrier female. This gene is said to be sex-linked. Because it is found only on the X chromosome, its inheritance is affected by the sex of an individual. For example, the genetic diagram shows the possible offspring born to a heterozygous woman and a man with normal blood clotting. Neither parent had haemophilia, yet there is a one in four chance that they will have a boy child with haemophilia. The haemophilia allele comes from the mother. She is a symptomless carrier for haemophilia. Some of the genes for red-green colour blindness are sex-linked and found on the X chromosome. Let the allele B represent healthy vision and b represent colour blindness. The capital (B in this case) always denotes the dominant allele and lower case (b) denotes the recessive allele. An investigation of the inheritance of a single contrasting characteristic is known as a monohybrid cross. It had been noticed that the garden pea plant was either tall or dwarf. How this contrasting characteristic is controlled is made clear by analysing the monohybrid cross. From the monohybrid cross we see that: within an organism are genes (called ‘factors’ when first discovered) controlling characteristics such as ‘tall’ and ‘dwarf’ there are two alleles of the gene in each cell one allele has come from each parent each allele has an equal chance of being passed on to an offspring the allele for tall is an alternative form of the allele for dwarf the allele for tall is dominant over the allele for dwarf. We call this the Law of Segregation / Mendels Law of Segregation During meiosis, pairs of alleles separate so that each cell has one allele of a pair. Allele pairs separate independently during the formation of gametes. The dihybrid cross Another early investigation by Mendel into the inheritance of variation involved two pairs of contrasting characters, again using the garden pea plant. This is now referred to as a dihybrid cross. For example, pure-breeding pea plants from round seeds with yellow cotyledons (seed leaves) were crossed with pure-breeding plants from wrinkled seeds with green cotyledons (P generation). All the progeny (F1 generation) had round seeds with yellow cotyledons. When plants grown from these seeds were allowed to self-fertilise the following season, the resulting seeds (F2 generation) – of which there were more than 500 to be classified and counted – were of four phenotypes and were present in the ratio shown It was noticed that two new combinations (recombinations), not represented in the parents, appeared in the progeny: either ‘round’ or ‘wrinkled’ seeds can occur with either ‘green’ or ‘yellow’ cotyledons. Thus the two pairs of factors were inherited independently. That means either one of a pair of contrasting characters could be passed to the next generation. This tells us that a heterozygous plant must produce four types of gametes in equal numbers. From the dihybrid cross we can conclude a Law of Independent Assortment: During meiosis the separation of one pair of alleles is independent of the separation of another pair of alleles. The dihybrid test cross In the case of dihybrid inheritance, too, whilst homozygous recessive genotypes such as wrinkled green peas (rryy) can be recognised in the phenotype, homozygous round yellow peas (RRYY) and heterozygous round yellow peas (RrYy) look exactly the same. They can only be distinguished by the progeny they produce, as illustrated in a dihybrid test cross. Unlike the monohybrid test cross where the outcome is a ratio of 1 : 1, in the dihybrid test cross the outcome is a ratio of 1 : 1 : 1 : 1. The dihybrid cross for linked genes The dihybrid test cross the outcome is a ratio of 1 : 1 : 1 : 1 and the observed ratio here is quite different. In sheep, the allele for black wool (B) is dominant over the allele for white wool (b). Similarly, the allele for horns (H) is dominant over the allele for being hornless (h). Pure breeding horned sheep with black wool were crossed with pure breeding hornless sheep with white wool. (a) State the genotype and the phenotype of the F1 individuals produced as a result of this cross. (b) Two F1 offspring were mated together. Calculate the expected ratio of phenotypes in the F2 generation. In cats, the allele for grey fur (G) is dominant over the allele for beige fur (g). The allele for a solid coat (S) is dominant over the allele for a striped coat (s). A pure breeding solid, beige cat is crossed with a pure breeding striped, grey cat. (a) State the genotype and the phenotype of the F1 individuals produced as a result of this cross. (b) Calculate the phenotypes resulting from a cross between a pure breeding solid, beige cat and an F1 offspring. 2.4.2. Interpret and construct genetic diagrams, including Punnett Inheritance squares, to explain and predict the and results of monohybrid crosses and genetic diagrams dihybrid crosses that involve dominance, codominance, multiple alleles, and sex linkage. Monohybrid inheritance Monohybrid inheritance is the inheritance of a single gene. For example, albinism (the lack of melanin in the skin) is caused by a recessive allele, a. Imagine that a man with the genotype Aa and a woman with the genotype AA have children. Gametes are produced In their testes and ovaries. In the man, half of his sperm will contain the A allele and half will contain the a allele. All of the woman’s eggs will contain the A allele. » We can predict the likely genotypes of any children that they have using a genetic diagram. Genetic diagrams should always be set out as in Figure 16.4. We can therefore predict that there is an equal chance of any child born to them having the genotype AA or Aa. There is no chance they will have a child with albinism. Figure 16.5 shows what would happen if both parents had the genotype Aa. We can therefore predict that, each time they have a child, there is a 1 in 4 chance that it will have the genotype aa and have albinism. Dihybrid inheritance This involves the inheritance of two genes. For example, a breed of dog may have genes for hair colour and leg length. Allele A is dominant and gives brown hair. Allele a is recessive and gives black hair. Allele L is dominant and gives long legs. Allele l is recessive and gives short legs. As before, always begin by writing down all the possible genotypes and phenotypes. A dihybrid cross A genetic diagram showing a dihybrid cross is set out exactly as for a monohybrid cross. The only difference is that there will be more different types of gamete. Each gamete will contain just one allele of each gene. The genetic diagram in Figure 16.8 shows the offspring we would expect from a cross between two dogs that are both heterozygous for both genes. We would therefore expect offspring in the ratio 9 brown hair, long legs : 3 brown hair, short legs : 3 black hair, long legs : 1 black hair, short legs. Notice: Each gamete contains one allele of each gene (one of either A or a, and one of either L or l). The ratio 9:3:3:1 is typical of a dihybrid cross between two heterozygotes, where the alleles of both genes show dominance (as opposed to codominance). Multiple alleles Many genes have more than two alleles. For example, the gene that determines the antigen on red blood cells, and therefore your blood group, has three alleles – IA, IB and Io. IA and IB are codominant. They are both dominant to Io, which is recessive. Genetic diagrams involving multiple alleles are constructed in the same way as before. For example, you could be asked to use a genetic diagram to show how parents with blood groups A and B could have a child with blood group O (Figure 16.6). When solving a problem like this: » Begin by working out and then writing down a table of genotypes and phenotypes, which you can easily refer back to as you work through the problem. » Consider whether you can tell the genotype of any of the individuals in the problem from their phenotype. Here, we know that the child with blood group O must have the genotype IoIo. » Work back from there to determine the genotypes of the parents. In this case, each of them must have had an Io allele to give to this child. » Always show a complete genetic diagram. Do not take short cuts, even if you can see the answer straight away, as there will be marks for showing each of the steps in the diagram. Construct a genetic diagram to predict the genotypes and phenotypes of the children of two people with blood group AB. Sex linkage A sex-linked gene is one that is present on the X chromosome but not on the Y chromosome. The X chromosome is longer than the Y chromosome. Most of the genes on the X chromosome are not present on the Y chromosome. These are called sex-linked genes. – For example, a gene that determines the production of red-receptive and green receptive pigments in the retina of the eye is found on the X chromosome. – A woman has two copies of this gene, because she has two X chromosomes. A man has only one copy, because he has only one X chromosome. – There is a recessive allele of this gene that results in red-green colour-blindness. – If the normal allele is A, and the recessive abnormal allele is a, then the possible genotypes and phenotypes a person may have are as shown in Table 16.5. You are asked to predict the chance of a woman who is a carrier for colour blindness (that is, heterozygous) and a man with normal vision having a colour-blind child There is therefore a 1 in 4 chance that a child born to this couple will be a colour- blind boy. There is a 1 in 2 chance of any boy that is born being colourblind. Explain why a man who is colour-blind cannot pass this condition to his son. The allele for colour blindness is carried on the X chromosome. A man passes only his Y chromosome to his son. 2.4.3. Interpret and construct Inheritance genetic diagrams, including Punnett and squares, to explain and predict the genetic diagrams results of monohybrid crosses and dihybrid crosses that involve autosomal linkage and epistasis Linkage There are two types of linkage in genetics: sex linkage and autosomal linkage Autosomal linkage: This occurs on the autosomes (any chromosome that isn’t a sex chromosome) Two or more genes on the same chromosome do not assort independently during meiosis These genes are linked and they stay together in the original parental combination If the loci of the 2 linked genes are not that If the loci of the 2 linked are extremely close on the chromosome, they result in a close on the chromosome, they result in a higher % of recombinants. very small % of recombinants. The fruit fly, Drosophila, normally has a striped body and antennae with a feathery arista. The gene for body colour and the gene for antennal shape are close together on the same chromosome and so are linked. A black body with no stripes results from a recessive allele called ‘ebony’. A recessive allele for antennal shape, called ‘aristopedia’, gives an antenna looking rather like a Drosophila leg, with two claws on the end. The alleles of these two genes that affect body colour and antenna shape are: A fly homozygous for striped body and normal antennae was crossed with a fly homozygous for ebony body and aristopedia antennae. All the offspring had striped bodies and normal antennae. You can use a genetic diagram to show this. To help keep track of linked alleles in a genetic diagram, it is best to bracket each linkage group. So, where you would write EEAA for the genotype if there was no linkage, here you write (EA)(EA). The genotypes of the plants that were crossed to produce the heterozygous plants were YYPP and yypp. Construct two genetic diagrams to show the expected offspring ratios from crossing two of the heterozygous plants if: a the genes are linked b the genes are not linked The genotypes of the plants that were crossed to produce the heterozygous plants were YYPP and yypp. Construct two genetic diagrams to show the expected offspring ratios from crossing two of the heterozygous plants if: a the genes are linked b the genes are not linked Epistasis Sometimes, two genes at different loci interact to produce a phenotype. This interaction of different genes is called epistasis. For example, in mice, two genes affect fur colour: Gene for distribution of melanin in hairs: A produces banding (agouti), a produces a uniform distribution. Gene for presence of melanin in hairs: B produces melanin, b does not produce melanin. Clearly, gene A/a can only have an effect if the pigment melanin is present. If there is no melanin, fur colour is white 1 Construct a genetic diagram to predict the ratios of phenotypes resulting from a cross between two mice with the genotypes AaBB and AaBb. 2 How could you use a test cross to find out the genotype of a white mouse? 2 A white mouse could have the genotype AAbb, Aabb or aabb. We could cross this mouse with a pure-breeding black mouse, with the genotype aaBB. If the unknown mouse has the genotype AAbb, then all the offspring will be agouti (AaBb). If the unknown mouse has the genotype Aabb, then half the offspring will be agouti (AaBb) and half will be black (aaBb). If the unknown mouse has the genotype aabb, then all the offspring will be black (aaBb). We would expect offspring in the ratio 3 agouti : 1 black. When recessive alleles at one locus mask the expression of both (dominant and recessive) alleles at another locus, this is called recessive epistasis. Two genes are involved in coat colour determination in Labrador retrievers. Genes BB and Bb result in a black coat, gene bb produces a golden coat. The chocolate coat colour of Labrador retrievers is a result of homozygosity for a gene that is epistatic to the gene for coat colour. The epistatic gene has two alleles, the dominant allele (E) enables the coat colour allele to be expressed. If the recessive allele (e) is homozygous, coat colour is not expressed on the body but the nose is black if allele B is present or brown if allele b is homozygous. Pigment production (B) and subsequent incorporation (E) into the hair shaft are controlled by two separate genes. For the body coat to have a colour expressed, a dominant allele of the epistatic gene, E, must be present. For the coat to be black, the dominant coat colour allele B must also be present Dominant epistasis is the effect of a dominant allele of one gene masking the action of either allele of the other gene. An example of dominant epistasis is found for fruit colour in summer squash. There are three types of fruit colour found in this squash: white, yellow and green. White colour is controlled by the dominant gene W and yellow colour by the dominant gene G. White is dominant over both yellow and green. The green fruits are produced when the genotype is wwgg. A cross between plants having white and yellow fruits produces F1 with white fruits. A cross of F1 plants produces plants with white, yellow and green coloured fruits in F2 in a 12 : 3 : 1 ratio (Figure 16.21). This is because W is dominant to w and epistatic to alleles G and g. Hence it will mask the expression of G/g alleles. Thus, in F2, plants with WWGG and WWgg genotypes will produce white fruits; plants with wwGG and wwGg will produce yellow fruits and those with wwgg genotype will produce green fruits. So the normal dihybrid ratio 9 : 3 : 3 : 1 is modified to a 12 : 3 : 1 ratio in the F2 generation. Inheritance 2.4.4. Interpret and construct and genetic diagrams, including Punnett genetic diagrams squares, to explain and predict the results of test crosses. If you cross an individual showing the dominant characteristics in their phenotype with one showing the recessive characteristics, you can use the phenotypes of the offspring to work out the genotype of the unknown parent. If you cross an individual showing the dominant characteristics in their phenotype with one showing the recessive characteristics, you can use the phenotypes of the offspring to work out the genotype of the unknown parent. 2.5.1. Explain the relationship between genes, proteins and phenotype. with respect to the: Genes, proteins, TYR gene, tyrosinase and albinism and HBB gene, haemoglobin, and sickle cell anaemia phenotype F8 gene, factor VIII, and haemophilia HTT gene, huntingtin and Huntington’s disease Albinism Albinism is a rare inherited condition of humans (and other mammals) in which the individual has a block in the biochemical pathway by which the pigment melanin is formed. Albinos have white hair, very light coloured skin and pink eyes. Albinism shows a pattern of recessive monohybrid inheritance in humans. In the chart shown of a family with albino members, albinism occurs infrequently, skipping two generations altogether. Biochemical basis of albinism – the relationship between gene, enzyme and phenotype A mutation in the gene for the enzyme tyrosinase, in which glutamine is substituted for arginine at a particular locus, results in a defective gene that fails to code for the tyrosinase protein. As a consequence, active tyrosinase and the pigment melanin are absent from pigment-forming cells in the body. This has evident impacts on the phenotype. The mutated allele is recessive, so the albino people are homozygous for the mutant allele. The TYR gene and albinism Melanin is the dark pigment that gives fur, skin and the iris their colour. It is produced from an amino acid called tyrosine by a series of enzyme-controlled chemical reactions. » The TYR gene is the code for an enzyme called tyrosinase. » Tyrosinase is an enzyme that is needed in the production of melanin. » Tyrosinase is needed in the first stage of melanin production. It converts an amino acid into a substance called dopaquinone, which is eventually converted to melanin by another series of reactions. » There have been over 100 different mutations to the TYR gene identified in mammals. In most cases, this means that the enzyme tyrosinase does not function. If a mammal inherits two copies of a TYR gene that does not produce functional tyrosinase, it has a condition called albinism. Albinos have white hair or fur, pink-coloured irises and pale skin. Alleles for albinism are recessive – if there is one copy of a functional TYR gene, it can usually produce sufficient tyrosinase to produce melanin. TYR gene & albinism Humans with albinism lack the pigment melanin in their skin, hair and eyes This causes them to have very pale skin, very pale hair and pale blue or pink irises in the eyes There is a metabolic pathway for producing melanin: 1.The amino acid tyrosine is converted to DOPA by the enzyme tyrosinase 2.DOPA is converted to dopaquinone again by the enzyme tyrosinase 3.Dopaquinone is converted to melanin tyrosine → DOPA → dopaquinone → melanin A gene called TYR located on chromosome 11 codes for the enzyme tyrosinase There is a recessive allele for the gene TYR that causes a lack of enzyme tyrosinase or the presence of inactive tyrosinase Without the tyrosinase enzyme tyrosine can not be converted into melanin The HBB gene, haemoglobin and sickle-cell anaemia The HBB gene codes for one of the polypeptides in a haemoglobin molecule. In the genetic disease sickle-cell anaemia, the gene that codes for the β polypeptide has the base T where it should have the base A. This means that one triplet is different, so a different amino acid is used when the polypeptide chain is constructed on a ribosome. » These two different forms of the gene are different alleles. The normal allele is HbA and the sickle-cell allele is HbS. » The normal allele is as follows: – DNA base sequence: GTG CAC CTG ACT CCT GAG GAG AAG TCT – Amino acid sequence: Val–His–Leu–Thr–Pro–Glu–Glu–Lys–Ser– » The abnormal allele is as follows: – DNA base sequence: GTG CAC CTG ACT CCT GTG GAG AAG TCT – Amino acid sequence: Val–His–Leu–Thr–Pro–Val–Glu–Lys–Ser– » The abnormal β polypeptide has the amino acid valine where it should have the amino acid glutamic acid. These amino acids are on the outside of the haemoglobin molecule when it takes up its tertiary and quaternary shapes. » Glutamic acid is a hydrophilic amino acid. It interacts with water molecules, helping to make the haemoglobin molecule soluble. » Valine is a hydrophobic amino acid. It does not interact with water molecules, making the haemoglobin molecule less soluble. » When the abnormal haemoglobin is in an area of low oxygen concentration, the haemoglobin molecules stick to one another, forming a big chain of molecules that is not soluble and therefore forms long fibres. This pulls the red blood cells (inside which haemoglobin is found) out of shape, making them sickle-shaped instead of round. They are no longer able to move easily through the blood system and may get stuck in capillaries. This is painful and can be fatal. HBB gene & sickle cell anaemia Sickle cell anaemia is a condition that causes individuals to have frequent infections, episodes of pain and anaemia Humans with sickle cell anaemia have abnormal haemoglobin in their red blood cells β-globin is a polypeptide found in haemoglobin that is coded for by the gene HBB which i found on chromosome 11 There is an abnormal allele for the gene HBB which produces a slightly different amino acid sequence to the normal allele The change of a single base in the DNA of the abnormal allele results in an amino acid substitution The DNA base sequence GAG is replaced by GTG This means that CTC is replaced by CAC on the complementary DNA template strand, meaning that GAG is replaced by GUG in the resulting mRNA This change in amino acid sequence results in an abnormal β-globin polypeptide The amino acid Glu is replaced with Val The abnormal β-globin in haemoglobin affects the structure and shape of the red blood cells They are pulled into a half moon shape They are unable to transport oxygen around the body They stick to each other and clump together blocking capillaries A homozygous individual that has two abnormal alleles for the HBB gene produces only sickle cell haemoglobin They have sickle cell anaemia and suffer from the associated symptoms A heterozygous individual that has one normal allele and one abnormal allele for the HBB gene will produce some normal haemoglobin and some sickle cell haemoglobin They are a carrier of the allele They may have no symptoms The F8 gene, factor VIII and haemophilia Clotting factors are plasma proteins that are involved in the clotting of blood. » The F8 gene codes for factor VIII clotting protein, which is mainly made by liver cells. » Inactive factor VIII circulates in the blood bound to another clotting protein called von Willebrand factor. When a blood vessel is injured, factor VIII becomes active and separates from von Willebrand factor. The active factor VIII then initiates another series of reactions, leading to blood clotting. » Mutations in the F8 gene cause the production of an abnormal factor VIII protein, or a reduction in the amount of factor VIII produced. This is a condition called haemophilia A, the phenotype of which is an inability to clot blood, so bleeding can be extremely difficult to control. » The F8 gene is located on the X chromosome, so haemophilia is a sex-linked condition. » Many different mutations in the F8 gene have been identified. F8 gene & haemophilia Factor VIII is a coagulating agent that plays an essential role in blood clotting The gene F8 codes for the Factor VIII protein There are abnormal alleles of the F8 gene that result in: Production of abnormal forms of factor VIII Less production of normal factor VIII No production of factor VIII A lack of normal factor VIII prevents normal blood clotting and causes the condition haemophilia The F8 gene is located on the X chromosome This means F8 is a sex-linked gene Haemophilia is a sex-linked condition If males have an abnormal allele they will have the condition as they have only one copy of the gene Females can be heterozygous for the F8 gene and not suffer from the condition but act as a carrier The HTT gene, huntingtin and Huntington’s disease Most harmful genetic conditions tend to be caused by recessive alleles. An example of a genetic condition caused by a dominant allele is Huntington’s disease. » The HTT gene codes for a protein called huntingtin. » The role of huntingtin is not yet fully understood, although it is involved in the transport of vesicles and mitochondria in neurones. » The HTT gene has a repeated CAG triplet sequence. This triplet codes for the amino acid glutamine. Different alleles have different numbers of this triplet – anything between nine and 35 repeats – producing functional huntingtin protein. » Mutant alleles of the HTT gene, with more than 40 CAG triplets, produce huntingtin protein that causes damage to neurones in the brain. These effects are usually not seen until the person is between 35 and 44 years old, when they begin to develop problems with muscle coordination and cognitive difficulties. This condition is called Huntington’s disease. » Mutant alleles that have between 36 and 40 CAG repeats may cause Huntington’s disease in some cases. » Because Huntington’s disease is caused by a dominant allele, possession of only one copy of a mutant HTT gene will result in the disease. HTT gene & Huntington's disease Huntington’s disease is a genetic condition that develops as a person ages Usually a person with the disease will not show symptoms until they are 30 years old + An individual with the condition experiences neurological degeneration; they lose their ability to walk, talk and think The disease is ultimately fatal It has been found that individuals with Huntington's disease have abnormal alleles of the HTT gene The HTT gene codes for the protein huntingtin which is involved in neuronal development People that have a large number (>40) of repeated CAG triplets present in the nucleotide sequence of their HTT gene suffer from the disease The abnormal allele is dominant over the normal allele If an individual has one abnormal allele present they will suffer from the disease 2.6.1 Describe the differences Control between structural genes and of regulatory genes and the differences gene expression between repressible enzymes and inducible enzymes. L.O: Describe the differences between structural genes and regulatory genes and the differences between repressible enzymes and inducible enzymes. Gene Control The nucleus of every cell in the human body contains the same genes However not every gene is expressed in every cell. Not all of these genes are expressed all the time. There are several mechanisms that exist within cells to make sure the correct genes are expressed in the correct cell at the right time. They involve regulatory genes. L.O: Describe the differences between structural genes and regulatory genes and the differences between repressible enzymes and inducible enzymes. Structural & regulatory genes A structural gene codes for a protein that has a function within a cell. For example, the F8 gene codes for the protein Factor VIII involved in blood clotting. A regulatory gene codes for a protein that helps to control the expression of another gene. Structural and regulatory genes that work together are usually found close together. L.O: Describe the differences between structural genes and regulatory genes and the differences between repressible enzymes and inducible enzymes. Inducible & repressible enzymes Some genes code for proteins that form enzymes Some enzymes are required all the time and some are required only at specific times The expression of enzyme-producing genes can be controlled Inducible enzymes are only synthesized when their substrate is present The presence of the substrate induces the synthesis of of the enzyme by causing the transcription of the gene for the enzyme to start. Repressible enzymes are synthesized as normal until a repressor protein binds to an operator. The presence of the repressor protein represses the synthesis of the enzyme by causing the transcription of the gene for the enzyme to stop. Controlling when enzymes are synthesized can be beneficial for cells as it stops materials and energy being wasted For example, using materials and energy to synthesize an enzyme when its substrate is not present and it can’t carry out its function would be highly wasteful L.O: Describe the differences between structural genes and regulatory genes and the differences between repressible enzymes and inducible enzymes. L.O: Describe the differences between structural genes and regulatory genes and the differences between repressible enzymes and inducible enzymes. L.O: Describe the differences between structural genes and regulatory genes and the differences between repressible enzymes and inducible enzymes. 2.6.2 Describe genetic control of Control protein production in a prokaryote of using the lac operon (knowledge of gene expression the role of cAMP is not expected). One of the most studied genes is the one that codes for the production of the enzyme β-galactosidase (also known as lactase). This enzyme is used by some bacteria to hydrolyse lactose in the bacterium’s environment to glucose and galactose, which can then be absorbed and used as an energy source by the cell. A structural gene is one that codes for the production of a protein that is used by the cell. Some structural genes live up to their name by coding for proteins that become part of a structure in the cell, but many structural genes have other roles, such as coding for enzymes. The gene that codes for the production of β-galactosidase is an example of a structural gene. The expression of the lactase gene is controlled by other genes that lie close to it on the circular DNA (DNA molecule). These are called regulatory genes. L.O: Describe genetic control of protein production in a prokaryote using the lac operon Structural and regulatory genes that work together are generally found in a group, and this cluster of genes is called an operon. The operon that is responsible for the production of lactase in bacteria is called the lac operon. In the bacterium Escherichia coli, the number of molecules of β-galactosidase present in a cell varies according to the concentration of lactose in the medium in which the bacterium is growing. The quantity of the enzyme is altered by switching the transcription of the β-galactosidase gene on or off. L.O: Describe genetic control of protein production in a prokaryote using the lac operon The lac operon also contains other structural genes, besides the one that codes for β- galactosidase. There are three structural genes altogether: lacZ, coding for β-galactosidase lacY, coding for permease (which allows lactose to enter the cell) lacA, coding for transacetylase. Transcription of all of these genes is controlled by the same promoter, and they are all transcribed at the same time. L.O: Describe genetic control of protein production in a prokaryote using the lac operon L.O: Describe genetic control of protein production in a prokaryote using the lac operon The sequence of events when there is no lactose in the When lactose is present in the medium in which the medium in which the bacterium is growing is as bacterium is growing, the following processes occur. follows. Lactose is taken up by the bacterium. The regulatory gene codes for a protein called a Lactose binds to the repressor protein, distorting its repressor. shape and preventing it from binding to DNA at the The repressor binds to the operator region, close to operator site. the gene for β-galactosidase. Transcription is no longer inhibited and messenger Because the repressor is attached to the operator, RNA polymerase cannot bind to DNA at the promoter region. RNA is produced from the three structural genes. The genes have been switched on and are transcribed As a result, there is no transcription of the three together. The bacterium can now absorb and break structural genes. down lactose. The repressor protein has two binding sites. This repressor protein can bind to DNA at one site and to lactose at the other. When lactose binds to its site, the shape of the repressor protein changes so that the DNAbinding site is closed. L.O: Describe genetic control of protein production in a prokaryote using the lac operon This mechanism allows the bacterium to produce β-galactosidase, permease and transacetylase only when lactose is available in the surrounding medium and to produce them in equal amounts. It avoids the waste of energy and materials in producing enzymes for taking up and hydrolysing a sugar that the bacterium may never meet. However, the sugar can be hydrolysed when it is available. The enzyme β-galactosidase is an inducible enzyme. This means that it is synthesised only when its substrate is present. The presence of the substrate induces (causes) the transcription of the gene for the enzyme. The binding of the effector molecule (which in this case is lactose) to the repressor prevents the repressor from binding to the operator, the repressor is released and transcription proceeds. The production of other enzymes, called repressible enzymes, is controlled in a slightly different way. Here, the binding of the effector molecule to the repressor helps it to bind to the operator. So the repressor attaches to the operator region, which stops transcription. L.O: Describe genetic control of protein production in a prokaryote using the lac operon L.O: Describe genetic control of protein production in a prokaryote using the lac operon L.O: Describe genetic control of protein production in a prokaryote using the lac operon 2.6.3 State that transcription factors Control are proteins that bind to DNA and of are involved in the control of gene gene expression expression in eukaryotes by decreasing or increasing the rate of transcription. Eukaryotes do not have operons as prokaryotes do. Instead, the expression of genes in eukaryotes is controlled by transcription factors. A transcription factor is a protein that binds to DNA and affects whether or not a gene is transcribed. The role of transcription factors is to make sure that genes are expressed in the correct cell at the correct time and to the correct extent. In humans, for example, about 10% of genes are thought to code for proteins that act as transcription factors. L.O: State that transcription factors are proteins that bind to DNA and are involved in the control of gene expression in eukaryotes by decreasing or increasing the rate of transcription. Some transcription factors bind to the promoter region of a gene, either allowing or preventing its transcription. Their presence either increases or decreases the rate of transcription of a gene. For example, production of the enzyme amylase in germinating seeds depends on a transcription factor called PIF (phytochrome interacting protein) binding with the promoter region next to the gene that codes for amylase. » When no gibberellin is present, PIF is bound to a protein called a DELLA protein. This prevents PIF binding with the promoter for the amylase gene, so no amylase is made. » When gibberellin is present, it binds with a receptor and an enzyme. This activates the enzyme, which breaks down the DELLA protein. PIF is now free to bind to the promoter for the amylase enzyme, and amylase is produced. L.O: State that transcription factors are proteins that bind to DNA and are involved in the control of gene expression in eukaryotes by decreasing or increasing the rate of transcription. L.O: State that transcription factors are proteins that bind to DNA and are involved in the control of gene expression in eukaryotes by decreasing or increasing the rate of transcription. L.O: State that transcription factors are proteins that bind to DNA and are involved in the control of gene expression in eukaryotes by decreasing or increasing the rate of transcription.