فسلجه3).docx
Document Details
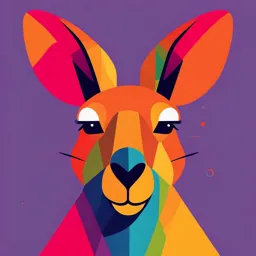
Uploaded by DecisiveHonor
Department of Anesthesia
Full Transcript
Cardiac Cycle The cardiac cycle refers to the sequence of events that occur during one heartbeat. The contraction phase of a cardiac cycle is known as systole (sis'-to-le); the relaxation phase is called diastole (di'-as-to-le). These phases are illustrated in figure 12.8. Note that the ventricles...
Cardiac Cycle The cardiac cycle refers to the sequence of events that occur during one heartbeat. The contraction phase of a cardiac cycle is known as systole (sis'-to-le); the relaxation phase is called diastole (di'-as-to-le). These phases are illustrated in figure 12.8. Note that the ventricles are relaxed when the atria contract, and the atria are relaxed when the ventricles contract. Systole increases blood pressure within a chamber, while diastole decreases blood pressure within a chamber. When both the atria and ventricles are relaxed between beats, blood flows passively into the atria from the large veins leading to the heart and then passively into the ventricles. Then, the atria contract (atrial systole), forcing more blood into the ventricles so that they are filled. Immediately thereafter, the ventricles contract. Ventricular systole produces high blood pressure within the ventricles, which causes both AV valves to close and both semilunar valves to open. Opening of the semilunar valves allows blood to move into the arteries leading from the heart. Ventricular diastole immediately follows and the decrease in ventricle pressure allows the AV valves to open. Simultaneously, the semilunar valves close because of the greater blood pressure within the arteries. The cardiac cycle is then repeated. Study these relationships in figure 12.8. Heart Sounds The sounds of the heartbeat are usually described as lubdup (pause) lub-dup, and so forth. These sounds are produced by the closing of the heart valves. The first sound results from the closing of the AV valves in the beginning of ventricular systole. The second sound results from the closing of the semilunar valves in the beginning of ventricular diastole. If any of the heart valves are defective and do not close properly, an additional sound, known as a heart murmur, may be heard. Pulmonary Aortic Pulmonary Aortic valve closed valve closed va|ve open valve open Atrial diastole Atrial systole Tricuspid Ventricular and mitral systole valves open Ventricular diastole (b) Tricuspid and mitral valves closed Figure 12.8 The Cardiac Cycle. (a) Blood flows from the atria into the ventricles during ventricular diastole, (b) Blood is pumped from the ventricles during ventricular systole. Heart Conduction System The heart is able to contract on its own because it con tains specialized cardiac muscle tissue that spontane ously forms impulses and transmits them to the myocardium to initiate contraction. This specialized tissue forms the conduction system of the heart, which consists of the sinoatrial node, atrioventricular node, AV bundle, bundle branches, and ventricular fibers. Observe the location of the conduction system and its parts in figure 12.9. The sinoatrial (si-no-a'-tre-al) node (SA node) is located in the right atrium at the junction of the superior vena cava. It is known as the pacemaker of the heart because it rhythmically forms electrical impulses to initiate each heartbeat. The impulses are transmitted to the myocardium of the atria, where they produce a simultaneous contraction of the atria. The flow of impulses causes contraction of the atria from superior to inferior, forcing blood into the ventricles. At the same time, the impulses are carried to the atrioventricular node (AV node), which is located in the right atrium near the junction with the interventricular septum. There is a brief time delay as the impulses pass slowly through the AV node, which allows time for the ventricles to fill with blood. From the AV node, the impulses pass along the AV bundle (bundle of His), a group of large fibers that divide into left and right bundle branches extending inferiorly to the interventricular septum and superior to the lateral walls of the ventricles. Interatrial septum Bundle branches Ventricular fibers AV Ventricular Interventricular bundle fibers septum Figure 12.9 The heart conduction system. Arrows indicate the flow of impulses from the SA node. fPlRJ Electrocardiogram The origination and transmission of impulses through the conduction system of the heart generate electrical currents that may be detected by electrodes placed on the body surface. An instrument called an electrocardiograph is used to transform the electrical currents picked up by the electrodes into a recording called an electrocardiogram (ECG or EKG). Figure 12.10 shows a normal ECG of five cardiac cycles and an enlargement of a normal ECG of one cardiac cycle. Note that an ECG consists of several deflections, or waves. These waves correlate with the flow of impulses during particular phases of the cardiac cycle. An electrocardiogram has three distinct waves: the P wave, QRS complex, and T wave. The P wave is a small wave. It is produced by the depolarization of the atria. The QRS complex is produced by the depolarization of the ventricles. The greater size of the QRS complex is due to the greater muscle mass of the ventricles. The last wave is the T wave, which is produced by the repolarization of the ventricular myocardium. The repolarization of the atria is not detected because it is masked by the stronger QRS complex. Regulation of Heart Function Cardiac output is the volume of blood pumped from each ventricle in one minute, and it is an important measure of heart function. It is determined by two factors: stroke volume and heart rate. Stroke volume (SV) is the volume of blood pumped from each ventricle per heartbeat. Multiplying this volume by the heart rate (HR), heartbeats per minute, yields the cardiac output (CO). CO = SV x HR At normal resting values of a stroke volume of 70 ml/beat and a heart rate of 72 beats/min, the cardiac output is 5,040 ml/min. This means that the total volume Figure 12.10 A normal ECG showing one cardiac cycle. of blood, 4 to 6 liters, passes through each ventricle of the heart each minute. Cardiac output increases with exercise because both stroke volume and heart rate increase. Heart function is regulated by factors both internal and external to the heart. For example, venous return, the amount of blood returning to the heart during diastole, is an internal factor that affects stroke volume. If venous return increases, more blood enters and is pumped from the ventricles, increasing the stroke volume and cardiac output. Heart rate is primarily controlled externally by the autonomic nervous system, although hormones and certain ions also affect it. Autonomic Regulation The cardiac control center consists of both sympathetic and parasympathetic components. Nerve impulses transmitted to the heart via sympathetic axons cause an increase in heart rate and contraction strength, while nerve impulses transmitted by parasympathetic axons cause a decrease in heart rate. The cardiac control center constantly adjusts the frequency of sympathetic and parasympathetic nerve impulses to produce a heart rate and a contraction strength that meets the changing needs of tissue cells (figure 12.11). Figure 12.11 The rate and strength of heart contractions are regulated by the antagonistic actions of sympathetic (colored blue) and parasympathetic (colored red) divisions of the autonomic nervous system. Sensory axons are colored green. Hi . When the heart is at rest, more parasympathetic nerve impulses than sympathetic nerve impulses are sent to the heart. As cellular needs for blood increase, a decrease in the frequency of parasympathetic nerve impulses and an increase in sympathetic nerve impulses cause heart rate to increase. Other Factors Affecting Heart Function Age, sex, physical condition, temperature, epinephrine, thyroxine, and the blood levels of calcium and potassium ions also affect the heart rate and contraction strength. strength. A high dose of K+ is often used in lethal injections, in which the abnormally high levels of blood K+ cause the heart to stop contracting. Abnormally low levels of blood K+ may cause potentially life-threatening abnormal heart rhythms. / Types of Blood Vessels Structure of Arteries and Veins Table 12.3 Comparison of Arteries, Capillaries, and Veins Type of Vessel Function Structure Arteries Carry blood from the heart to the capillaries Composed of tunica intima, tunica media, and tunica Control blood flow and blood pressure externa Contain more smooth muscle and elastic connective tissues than veins Capillaries Enable exchange of materials between blood and interstitial fluid Microscopic vessels composed of endothelium supported by areolar connective tissue Veins Return blood from capillaries to the heart Composed of tunica intima, tunica media, and tunica Serve as storage areas for blood externa Have thinner walls and larger lumens than arteries Large veins have venous valves. Areolar Artery Vein connective tissue Valve Endothelium Endothelium of tunica intima Areolar connective tissue of tunica intima Tunica media Tunica externa Capillary Figure 12.12 (a) The wall of an artery, (b) The wall of a vein, (c) The wall of a capillary. Blood Pressure The term blood pressure, the force of blood against the wall of the blood vessels, usually refers to arterial blood pressure in the systemic circuit-in the aorta and its branches. Arterial blood pressure is greatest during ventricular contraction (systole) as blood is pumped into the aorta and its branches. This pressure is called the systolic blood pressure, and it optimally averages 110 millimeters of mercury (mm Hg) when measured in the brachial artery. The lowest arterial pressure occurs during ventricular relaxation (diastole). This pressure is called the diastolic blood pressure, and it optimally averages 70 mm Hg (figure 12.15). The difference between the systolic and diastolic blood pressures is known as the pulse pressure (figure 12.15). The alternating increase and decrease in arterial blood pressure during ventricular systole and diastole causes a comparable expansion and contraction of the elastic arterial walls. This pulsating expansion of the arterial walls follows each ventricular contraction, and it may be detected as the pulse by placing the fingers on a superficial artery. Figure 12.17 identifies the name and location of superficial arteries where the pulse may be detected. Factors Affecting Blood Pressure Three major factors affect blood pressure: cardiac output, blood volume, and peripheral resistance. An increase in any of these factors causes an increase in blood pressure, while a decrease in any of these causes a decrease in blood pressure. Blood volume may be decreased by severe hemorrhage, vomiting, diarrhea, or reduced water intake. The decrease in blood volume causes a decrease in blood pressure. Many drugs used to treat hypertension (abnormally high blood pressure) act as diuretics, meaning they increase urine volume and as a result decrease blood volume. As soon as the lost fluid is replaced, blood pressure returns to normal. Conversely, if the body retains too much fluid, blood volume and blood pressure increase. A high-salt diet is a risk factor for hypertension because it causes the blood to retain more water as a result of osmosis, leading to an increase in blood volume. Peripheral resistance is the opposition to blood flow created by friction of blood against the walls of blood vessels. Increasing peripheral resistance will increase blood pressure, while decreasing peripheral resistance decreases blood pressure. Peripheral resistance is determined by vessel diameters, total vessel length, and blood viscosity. Arterioles play a critical role in controlling blood pressure by changing their diameters. As arterioles constrict, peripheral resistance increases and blood pressure increases accordingly. As arterioles dilate, peripheral resistance and blood pressure decrease. Peripheral resistance is directly proportional to the total length of the blood vessels in the body: the longer the total length of the vessels, the greater their resistance to flow. Obese people tend to have hypertension partly because their bodies contain more blood vessels to serve the extra adipose tissue. Viscosity is the resistance of a liquid to flow. For example, water has a low viscosity, while honey has a high viscosity. Blood viscosity is determined by the ratio of plasma to formed elements and plasma proteins. Increasing viscosity, or shifting the ratio in favor of the formed elements and plasma proteins, increases peripheral resistance and blood pressure. Both dehydration (loss of water from plasma) and polycythemia (elevated RBC count) can increase viscosity. Abnormally high levels of blood lipids and sugar are also risk factors for hypertension because they increase blood viscosity, in addition to promoting the formation of plaque on the vessel walls. Decreasing viscosity through over-hydration or certain types of anemia will decrease peripheral resistance and blood pressure. Control of Peripheral Resistance The sympathetic division of the ANS controls peripheral resistance primarily by regulating the diameter of blood vessels, especially arterioles. The integration center is the vasomotor center in the medulla oblon-gata. An increase in the frequency of sympathetic nerve impulses to the smooth muscle of blood vessels produces vasoconstriction, which increases resistance. The increase in resistance increases blood pressure and blood velocity. This response accelerates the rate of oxygen transport to cells and the removal of carbon dioxide from blood by the lungs. A decrease in sympathetic nerve impulse frequency results in vasodilation, which decreases resistance. The decrease in resistance decreases blood pressure and blood Fig. 12.14 capillary exchange Fig 12.17 arteries from which we can detect pulse