Flat Glass-Solar Education Guide PDF 2023
Document Details
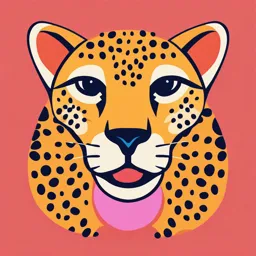
Uploaded by LovedDetroit
null
2023
Tags
Summary
This document is a `Flat Glass-Solar Education Guide`, published in 2023, providing an overview of solar energy, its interactions with glass, and various glazing types. It offers an introduction to window film technology and its applications in controlling comfort, glare, and furnishing deterioration.
Full Transcript
Flat Glass-Solar Education Guide 1 2023 Foreword The following material presented in this educational study guide is provided for the education of window film industry participants. Our hope is that IWFA members and non-members will use...
Flat Glass-Solar Education Guide 1 2023 Foreword The following material presented in this educational study guide is provided for the education of window film industry participants. Our hope is that IWFA members and non-members will use the information provided to promote window film professionally and competently. Additionally, accreditation tests are available through the IWFA education system. Passing grades on each test will give IWFA members additional accreditation references on the IWFA dealer locator. The information presented has been reviewed for technical accuracy by the IWFA Technical Committee. Therefore, we believe this guide presents a wide range of materials in a balanced and unbiased format. We not only welcome but encourage readers and users to continually offer suggestions for future edits. The Guide does not purport to state that any particular product should be used in any specific application. The user has the responsibility to ensure that any product selected and/or installed complies with all applicable laws, rules, regulations, standards, and other requirements. The IWFA does not design, develop, or manufacture any products, processes, or equipment referenced in this Guide and, accordingly, makes no guarantee, representation, or warranty expressed or implied, as to their fitness, merchantability, patent infringement, or any matter respecting their performance. The IWFA cannot guarantee and disclaims any responsibility for any specific result relating to the use of the Guide. We sincerely hope the use of this Guide in your business dealings will enhance your professional development and success. IWFA Board of Directors 2 Introduction The biggest problem in controlling comfort, glare, and furnishing deterioration in homes and offices is dealing with radiant energy from the sun. While walls and roofs absorb this energy, it literally pours through window and is absorbed by all it touches. The Guide will focus on describing the sun’s energy journey through the atmosphere, interaction with clear glass, variations in glass types and coatings, window glazing types and framing, window film constructions, and finally the measurement and impact of solar radiation on the interior of homes and buildings. The Sun – A Source of Radiant Electromagnetic Energy The sun is a tremendous source of energy. It is constantly sending its energy through space towards the earth in the form of electromagnetic radiation or energy waves. This transfer of heat from the sun to earth is called radiation. Throughout this Guide it will be important to remember that heat energy always flows from high temperature to lower temperatures. Freezers and coolers don’t “keep the cold in”; they prevent heat from entering and warming the interior contents. Earth’s atmosphere protects it from the sun’s energy reaching the surface. Changes in that atmosphere can change the rate at which that heat transfer occurs. Figure 1.1 3 Electromagnetic energy is expressed in units called wavelengths. A wavelength is the length of a full cycle in a repeating curve. As electromagnetic waves are impossible to see with normal vision it is helpful to use an example of something visual like the waves formed in a bowl of water in contact with a vibrating needle. The wavelength is the distance from the beginning of a positive phase through positive and negative phases to the beginning of the next positive phase. Figure 1.2 Individual waves are not visible within the electromagnetic spectrum although human senses and biology interact with these wavelengths in many ways. These waves are measured in nanometers. A nanometer (nm) equals one billionth of a meter or 0.0000000394 inches! Another common measure of electromagnetic energy is frequency, a measure of the number of wavelengths per second. Using the bowl and needle example, the frequency could be visualized by the number of waves hitting the side of the bowl per second. Figure 1.3 below, shows the electromagnetic spectrum from shorter, higher frequency wavelengths up to the longer, low frequency wavelengths. Figure 1.3 4 Measuring Light Energy Wavelengths Figure 1.4 shows the electromagnetic spectrum (EM), with nanometer measurements, starting at the low level of the scale with very short, high-energy wavelengths. Energy from the sun is only found in a portion of the EM spectrum and is referred to as the solar spectrum. Figure 1.4 Ultraviolet Included in the powerful, short wavelength band are the invisible and more energetic (higher frequency) ultraviolet rays. There are three types of ultraviolet rays: UV-C (100 – 290 nanometers), UV-B (290 -320 nanometers), and UV-A (320- 400 nanometers). The earth’s atmosphere and ozone layer filter out most UV-C and a percentage of the UV-B. Visible What is considered the visible band of the solar spectrum runs from roughly 380 nanometers to 780 nanometers. “Visible” is a subjective term as there are no globally agreed limits to the visible spectrum. CIE (International Commission on Illumination) defines the visible radiation as “any optical radiation capable of causing a visual sensation”. Age plays a significant role in a person’s ability to see. UV absorption increases in the human lens over time thus blocking more and more of the UV region. This is nature’s way of protecting the eye as excessive UV is a strong contributor to macular degeneration. Most spectral charts show the UV region overlapping with the visible region between 380-400 nm. Industry standard measurements will be reviewed later in this Guide. A similar situation occurs at the top range of the visible region. Most experts believe the range where humans start to lose the ability to perceive light is somewhere between 760 – 830 nm. Above this range are other invisible rays that we cannot see as light but can only feel as heat. These are called infrared rays. Infrared 5 Infrared is electromagnetic energy with wavelengths great than that of red visible light. Infrared is in the solar spectrum from 780 nanometers to greater than 1 millimeter. There are different ranges in the Infrared regions. Near- IR is from 780 – 2500 nm. Far-IR radiation is re- radiated from objects that have been heated by the sun or other heat sources. Far-IR is measured from 2500 nm to 40,000 nm. Beyond that point the amount of radiation from the sun is extremely low. Solar Heat: Visible and Invisible Light Electromagnetic energy from anywhere in the solar spectrum will heat a surface but the intensity and energy from the different wavelengths are not equal at all wavelengths. Roughly 44% of the sun’s radiant energy is received by the earth in the form of visible light. Invisible light in the form of infrared solar energy accounts for another 53%, with ultraviolet radiation making up the final 3%. Other references may show slight differences in these percentages due to different standard setting bodies setting different wavelength cut-off points for UV, Visible, and IR radiation. Figure 1.5 below illustrates the radiation intensity in each wavelength range throughout the solar spectrum. Figure 1.5 Other Forms of Heat Transfer While it is important to understand the various forms of the sun’s electromagnetic energy, it is also important to understand how heat transfer works. There are three forms of heat transfer: radiation (discussed in the previous sections), conduction, and convection. Conduction Conduction transfers heat within an object or between two bodies that are in contact. It is a point-by-point process of heat transfer. Conduction can occur in solids, liquids, or gases that are at rest. Consider a cup of coffee. Using a microwave oven heats the coffee with microwave radiation. Conduction is the transfer of heat from the cup to the tabletop when the cup sits in contact with the table. Conduction is also what warms the cup but also the air immediately adjacent to the cup. The warmth felt when hands are placed around the cup without touching it. 6 Figure 1.6 Convection Convection is the transfer of energy in a liquid or gas due to the motion of that fluid. The motion may be natural or forced. Natural convection: Using the coffee cup example once again, natural convection occurs when the coffee cup is in a room with a cooler temperature than the coffee. As the surrounding air warms through conduction, it expands pulling new cooler air in and creating a cycle in which cooler air is warmed, expands, and continues to pull in cooler air. The transfer of heat through this natural air movement process is natural convection. Forced convection: Providing an outside force which moves the gas or liquid faster than would occur naturally. In the coffee cup example, blowing over the surface of the cup is forced convection. Figure 1.7 With both conduction and convection, the larger the temperature differences between the warmer body and the cooler body the faster the heat transfer occurs. 7 Thermal Equilibrium Heat energy flows from a high temperature to a lower temperature. When these temperatures balance heat stops flowing, and the system is said to be in thermal equilibrium. In the case of the coffee cup, once the temperature of the coffee reaches the temperature of the room, heat transfer stops. But in a closed system where the input energy continues, heat transfer can easily change directions. A closed jar of cool water placed in the sun will absorb radiant energy from the sun. If the contact surface and outdoor temperature are warmer than the jar and liquid, then energy from radiation, conduction, and convection are all flowing towards the jar and the air around the jar. 8 Once the temperature of the liquid inside the jar and the jar are warmer than the outside temperature and the contact surface the heat transfer from conduction and convection will change directions while the radiant heat transfer from the sun’s rays will continue to heat the liquid to the point at which the radiant energy cannot heat the liquid further than the conduction and convection cool it due to temperature differences. 9 What Happens when Sunlight Strikes Glass When sunlight (incidental solar radiation) strikes glass, three things can happen: 1. The energy can be reflected away from the glass. 2. The energy can be absorbed by the glass. 3. The energy can pass through the glass. GLASS ABSORBED Figure 1.10 The performance definition for each of these events is expressed as a percentage, which will total 100%. Let’s see what happens when sunlight strikes clear 1/8” glass. Total Solar Reflectance = 8% Total Solar Absorptance = 9% Total Solar Transmittance = 83% 10 One of the easiest ways to remember this equation is using the acronym RAT. The sum of the reflectance (R), Absorption (A), and the Transmittance (T) must always equal 100%. If the values on a specification sheet do not equal 100% then the manufacturer should be contacted. One percentage point in either direction may just be a rounding issue. It the variation from 100% is more than the data should be considered suspect. 11 Let’s explore next what happens after the sunlight strikes the glass and heat transfer occurs. Heat Flow to the Frame by Conduction Inward Heat Flow By Convection and Radiation ABSORBED Outward Heat Flow By Convection and Radiation Figure 1.12 The solar energy is reflected, absorbed, or transmitted. The reflected energy does not enter the room and the transmitted energy goes through the glass without stopping. The absorbed energy flow is more complicated with absorbed energy flowing inward, outward, or both depending on the temperature difference from inside to outside. This heat transfer happens through convection and re-radiation. The absorbed energy can also flow to the frame through conduction. Heat Transfer of Absorbed Energy in Glass While transmittance and reflectance are fairly easy concepts to grasp, solar absorption is more complex. As seen in the previous Diagram, the absorbed energy is partially transferred through radiation. Absorbed solar energy is said to be “re-radiated” is this case. This refers to the fact that the transfer of energy occurs at different wavelengths than the source energy. While solar energy absorbed is from Ultraviolet, Visible, and Near IR, it radiates away from the glass as Far IR. In the glass jar example, the jar and its contents are heated by near IR from the sun but the increase in temperature underneath the jar is reradiated Far IR. 12 The following two figures illustrate the heat transfer of absorbed energy in an architectural setting where the outdoor temperature is cooler than the indoor temperature through conduction and convection. Re-radiation is a surface phenomenon. As all heat transfer is from heat to cold the radiation from the glass will occur at different speeds depending on the outside and inside temperatures. Figure 1.7 illustrates all the heat transfers while Figure 1.8 only illustrates convection. In addition to absorbed solar energy, heat transfer can occur with Far IR energy from other sources. Diagram 1.9 illustrates winter heat loss from an interior Far IR heat source. Diagram 1.95 illustrates summer heat gain from an exterior Far IR heat source. In both cases heat will also re-radiate at the glass in both directions depending on temperature differential. SUMMER HEAT GAIN CONDUCTION Reradiation Reradiation PATIO 13 Emissivity As noted above re-radiation happens at the surface of the glass. Different types of surfaces differ in their re-radiation and absorption capabilities. The emissivity of the surface of a material is its effectiveness in emitting energy as thermal radiation. Thermal radiation is electromagnetic radiation that may include both visible, near IR, and Far IR radiation. Emissivity measurements are reported as a comparison to a surface that would have an extremely high absorption rate and would re-radiate all the energy absorbed. Following this logic, the lower the emissivity value the better the surface would be at limiting re-radiation. Consider aluminum foil wrapped around a baked potato. Aluminum has a very low emissivity value. Holding your hand over a baked potato wrapped in foil will not feel very warm but grabbing it will burn your hand through conduction. In the chart below, polished aluminum has an emissivity of 0.05. Glass is not an inherently low emissivity surface with an emissivity of 0.84. A “perfect” emitter would have an emissivity of 1.00. It follows that glass will re-radiate a lot of heat and windows are one of the biggest sources of heat transfer in a building. Material Emissivity Gold, polished 0.02 (Poor absorber, good reflector) Silver, polished 0.02 Aluminum, polished 0.05 Glass, 1/8” 0.84 Paper 0.89 Wood 0.91 White Enamel 0.91 Flat Black Paint 0.96 (Good absorber, poor reflector) 14 Review of Solar Terms Below are short definitions of many of the terms introduced in this Section. Remember to keep in mind the differences between the individual wavelength ranges of the electromagnetic spectrum. Some definitions refer to specific ranges within the solar spectrum while others refer to the complete solar spectrum. In this section the glass used in the examples was clear, single pane, 1/8” glass. The next section will give a brief history of glass and then explains differences in glass types, thicknesses, and coatings. In addition, the section will explain window systems. Total Solar Reflectance (TST) The percent of incident solar radiation that is reflected by a glazing system. Total Solar Absorptance (TSA) The percent of incident solar radiation that is absorbed by a glazing system. Total Solar Transmittance (TST) The percent of incident solar radiation that is transmitted (passes directly through) a glazing system. Visible Light Transmission (VLT) The percent of visible light that is transmitted (passes through) a glazing system. Visible Light Reflectance (VLR) The percent of visible light that is reflected by a glazing system. Ultraviolet Transmittance (UVT) The percent of ultraviolet radiation that is transmitted through a glazing system. Many people prefer to report the percent of ultraviolet radiation that is prevented from passing through the glazing system for ease of customer understanding, but the actual measurement is UVT. Infrared Transmittance (IRT) The percentage of infrared radiation that passes through a glazing system. As with UVT, many people prefer to report a value that is the percent of IR that is prevented from passing through a glazing system, but this is more problematic in the case of IR since there is a high degree of absorption and the wavelength range is large and highly variable by wavelength in solar intensity. Calculations for a more accurate performance value will be discussed later in this guide. Section II: Glass and Glazing Systems 15 History of Glass Glass may be one of the oldest known man-made materials with examples of glass found at historic sites dating back to 7000 BC. By 3000 BC glass was being used on a regular basis in Egypt but primarily for decorative purposes. It was another 1500 years before the art of making glass into useable shapes was perfected. The New York Metropolitan Museum showcases a vase believed to have been made around 1490 BC. In simple terms, glass is liquid sand reformed into a transparent “solid”. The process requires heating the quartz sand, also known as silica sand, to temperatures above 3090 degrees Fahrenheit until it melts into a clear liquid. The idea for making glass from sand may have had its origins from the natural occurrence of fulgurites. Fulgurites are tubes or crusts of glass formed when lighting strikes sand high in silica content and fuses the silica into a shape that mimics the path the lightning bolt travels through the sand. Unlike the movies suggest, fulgurite doesn’t look like glass but more like a sand encrusted piece of driftwood. Interestingly, fulgurites tend to be hollow and these hollow tubes when exposed to bright light can be somewhat transparent. Given the high temperatures needed to create glass it is not surprising that glass took centuries to perfect. Glass blowing, which was believed to have originated in Phoenicia around 50 BC, greatly improved the possible uses for glass. Hollow objects such as glasses and urns are produced by “catching” a blob of molten glass on the end of a long hollow tube and then blowing air into the molten glass to form a bubble in the middle. The glass is rolled and manipulated at the same time to keep the molten glass from slumping to one side. The Venetians became masters at glass making, finding additives to give more flexibility in the manufacturing process and introduce color to the glass. Still, glass making remained more of an art form than a production process. The first “window” glass was made by catching a glob of glass, spinning it to increase the circumference, and then pressing it against a flat surface to make a circular sheet of glass. While the glass was roughly uniform in thickness, it was certainly flatter on one side than the other and the spinning produced concentric circles visible in the 16 glass and a dimple in the center where the glass was removed from the blowing tube. The circular glass was then cut into a square or rectangular shape to be used as a windowpane. The air bubbles, uneven texture, and general poor clarity made it difficult to see clearly through the glass, but it did provide light transmission. The French perfected this type of glass by grinding and polishing the glass to improve clarity and thickness. The best of these became known as “French Panes”, a term used to this day to describe small lites of glass even though they are no longer made with this process. Modern Glass Glass making and specifically glass blowing remained an art and the industry was controlled by a small group of craftsmen motivated to keep the production of glass small and the skill required to produce it in high demand. This all changed in 1916 when Michael J. Owens mechanized the production of glass containers and perfected the first machine for flat drawn window glass. In 1955, manufacturing took another leap forward when Pilkington introduced the float glass manufacturing method. Types of Glass Glass can be categorized by the amount of heat used in the manufacturing process, namely hot, hotter, hottest. At the low end of the scale is annealed glass, followed by heat-treated glass, and finally tempered glass. All three types of glass start as annealed glass, but heat-treated and tempered glass are subjected to subsequent processes which change their breakage characteristics. Annealed glass can be cut at a glass shop, but heat-strengthened and tempered glass must be heat treated at the size they will be used as they cannot be cut to size after heat treatment. Annealed Float Glass The most common window glass available on the market is commonly referred to as annealed float glass or simple annealed glass. Annealed float glass is manufactured in a process where molten glass is poured continuously onto a bed of molten tin. The molten glass tends to seek a level configuration as it floats on the surface of the molten tin. The thickness of the glass is relative to the rate at which the molten glass flows from the tank onto the tin. If the flow rate is slowed down, the glass is thicker. Because the melting point of the tin is much less than that for the glass, the glass solidifies as it cools on top of the tin. Once the glass solidifies, it is fed into an annealing oven where it is slowly cooled so that the residual stresses are minimized. This process results in the production of a glass product, which is very flat with nearly parallel surfaces. Since annealed glass has a minimum amount of residual surface compression, it is subject to easy breakage. Annealed glass is the most fragile of all manufactured glass. It is subject to 17 breakage from airborne flying objects, human impact, and thermal stress fracture as a result of temperature changes. When annealed glass breaks, it does so in many sharp, irregular-shaped pieces referred to as shards. Depending on the cause of the glass breakage these jagged pieces of glass can be propelled at high speeds and can cause serious bodily injuries and even death. Heat Treated Glass Heat treated glass is a type of glass that is the result of a heating and controlled cooling process in order to induce a change in structure within the glass leading to an increase in strength. The glass is heated to about 1150oF and then cooled. This type of glass has a strength factor about twice the strength of annealed glass. Heat strengthened glass tends to break in a similar way to regular annealed glass. Almost all original properties of the glass remain unchanged. The glass is more resistant to heat-induced stress, wind-loads and impacts by wind-borne debris and hail. Glass strength is defined by the degree of edge or surface compression. Surface compression is the end results of the heat strengthening process, where the outer state of the glass is locked in a state of high compression, and the middle is in a state of tension. Tempered Glass Tempered glass is a type of glass that is the result of heating and rapid cooling to induce a change in structure leading to an increase in strength. Single sheets of annealed glass are heated to temperatures around 1200oF. This is the temperature at which annealed glass begins to soften. The outer surfaces of the glass are then rapidly cooled. This creates high compression in the surfaces. This type of glass is about four times stronger than regular annealed glass. The change in structure has two main benefits. First, the glass is much stronger, and second when the glass is broken it breaks into small fragments as opposed to the large, sharp, shards created by annealed glass. This is a major benefit in areas that are at high risk of accidental human impact such as sliding doors or shop front doors and windows. There is another great benefit to tempered glass. It is very resistant to cracking from the thermal stress caused by solar absorption and temperature differentials from edge to center of glass. This phenomenon is known as thermal shock fracture, and usually occurs when the edge and center of the glass pane have a relatively high difference in temperature. Chemically Strengthened Glass 18 There is another type of glass produced, which is called chemically strengthened glass. This type of glass is produced when glass is submerged in a molten salt bath at temperatures below normal annealing. This results in an exchange of ions at the surface level of the glass. This is a complex process beyond the scope of this document. Chemically strengthened glass has similar compressive strength to heat treated glass. The product is not generally used for window glass but more commonly seen in industries where very thin, strong glass is needed. This glass breaks in a similar fashion to annealed glass. How to Identify Glass Types Most tempered glass products are identified through a clearly visible corner etching stating that the glass complies with safety glazing standards. The presence of this marking is intended to assure that the glass is fully tempered. If there is not corner etching, the most direct way to tell the difference between annealed and heat strengthened lass in the field is using two sheets of “polarized film”. One sheet should be positioned on each side of the glass pane and the character of the light that shines through the glass is examined. Annealed glass will exhibit a neutral appearance, while heat-strengthened glass will exhibit a mottled display of residual stress patterns. Glass Constructions For the purposes of this training guide, glass constructions can be divided into two major categories: Monolithic Laminated Monolithic Monolithic glass is the simplest glass construction type. It consists of a single flat piece of glass of constant thickness. Virtually all monolithic glass produced today throughout the world is produced using the float glass method. Monolithic glass can be annealed, heat-treated, or tempered. Additionally monolithic glass can be coated or combined with additional monolithic layers into various glazing and window systems. While most glass used today is 1/8” or 3mm minimal thickness, new manufacturing methods have been developed to produce thinner commercially viable glass. 19 Figure 2.1 Laminated Glass Laminated glass is produced using two or more monolithic layers of glass permanently bonded together using an “interlayer”. The most common interlayer is polyvinyl butyral (PVB) although polyurethane (PU) interlayers are also used. The glass used can be annealed, heat- strengthened, or tempered. Figure 2.2 Laminated glass is designed to be used in areas where increased strength, impact resistance, noise reduction, or flying glass may cause serious injury. Glass can also be laminated to multiple layers that are not glass. Glass laminated with plastic glazing such as polycarbonate or acrylic is used primarily for safety or security uses and is discussed in more detail in the Safety and Security Training Guide. Other Miscellaneous Glass Types There are several different glass constructions that may be encountered in the marketplace such as: wired, textured, and patterned glass. The manufacturing processes associated with these types of glass typically introduce surface and edge flaws, which make them more susceptible to glass breakage. Discussions about the various uses for these glass types is outside the scope of this document. 20 Non-Clear Glass Tinted Glass As glass is now manufactured primarily by the float glass method, very little glass is manufactured for the architectural market utilizing a full-bodied coloration process. In this process the color is mixed in with the glass at the time of manufacture, resulting in a glass that is colored consistently throughout its thickness. Most tinted architectural glass is created by applying the color as a surface coating during the cooling phase of the manufacturing process when the glass is still in a semi-molten state, or in a separate operation after the glass is manufactured. Tinted glass is used in many commercial buildings for solar heat control, privacy, and exterior aesthetics. When used alone, tinted glass has very little reflectivity and achieves solar heat control primarily through absorption. It is often heat strengthened to limit the glass breakage risk. Tinted glass may be hard to detect if the tinting is light. If the architectural specifications for are not available, placing a white piece of paper behind the glass and viewing from the other side can be helpful. Reflective Glass Coatings Glass that has metallic or metallic oxide coatings applied onto the surface is generally known as reflective coated glass. These coatings have a wide range of visible light transmissions and colors. Many different colors are available by combining the metallic layers with tinted glass layers to yield colors such as silver, gold, copper, grey, bronze, blue, and green. Additionally metallic and metallic oxide coatings can be applied in combination yielding high performance glass coatings. Two specific coatings are known as “spectrally selective” and Low emissivity or “Low E” for short. Spectrally selective coatings are produced using a combination of metal and metal oxide coatings and have the distinction of producing glazing products with a combination of high visible light transmission and low visible reflectivity but high solar reflectivity. These glazing products are especially desirable in homes where owners do not desire the low light transmission and high reflectivity often seen in commercial buildings with reflective glass. Low emissivity coatings are produced using either a metal oxide or a combination of metal and metal oxides. It comes in an earlier version which is referred to as standard Low E and a newer version referred to as high performance Low E. Standard Low E coatings have very high visible light transmission, but very low emittance and very low visible and solar reflectance. This glass is used most often in heating dominant climates (northern climates in the northern hemisphere). When used in the proper window construction they can be very beneficial in reducing winter heat loss from interior heat sources while also allowing winter heat gain from the sun (free daytime heating). 21 High Performance Low E coatings have high visible light transmission, low visible light reflectance, low emittance, but high solar reflectivity. This glass is used most often in cooling dominant climates (mid and southern climates in the northern hemisphere). When used in the proper window construction these glazing materials can be very beneficial in reducing summer heat gain. Dynamic Coatings Dynamic coatings are those that change solar performance in some manner based on an input. They are of high interest among researchers as they offer the best performance as they can change based on the outdoor conditions. There are three general types of dynamic coatings and several variations within those types. Thermochromic – Coatings that change solar performance properties with temperature. Electrochromic – Coatings that change solar performance properties with electric stimulus. Photochromic – Coatings that change solar performance properties with light stimulus. Many different glass types are available today with many different combinations of properties. The number of variations takes on even greater complexing as the type of window constructions are included. Glass Breakage No discussion of glass is complete without discussing how and why it breaks. Glass can break for any number of reasons associated with either pressures on the glass or direct impact to the surface from objects. They include: Human Impact – An adult or child running into or falling into a piece of glass. Forced entry – Breakage resulting from attempted illegal entry through glass. Windstorm – Breakage from flying objects or pressures associated with windstorms Earthquake – Breakage from the racking motion created during an earthquake Blast – Breakage resulting from the pressure wave of an explosion. Nickel-sulfide inclusions – Tempered glass breakage from a glass contaminant. Thermal breakage- Cracking associated with edge stresses caused by heat. The first five of these are covered in more detail in the Safety and Security Training Manual but nickel sulfide inclusions and thermal breakage can occur without a significant event and are issues that every student of this guide should understand. 22 Nickel Sulfide Inclusions Infrequently glass will contain very small particles of nickel sulfide produced from a nickel contaminant in the glass during the float process. A subsequent tempering process make the glass 4-5 times stronger than annealed glass. The combination of this glass tension and a rare nickel sulfide stone forming in the center tension zone of the tempered glass can lead to a spontaneous breakage later when the glass is exposed to varying temperatures after installation. Float glass manufacturers go to great lengths to eliminate nickel in their processes and so this has become a rare form of glass breakage. It is important to remember that this can only occur in tempered glass, and it is likely to be an edge defect or other impact that breaks tempered glass. Thermal Stress Fractures The most common cause of glass breakage not associated with an impact or pressure wave event is thermal stress fractures. Thermal stress fractures are the result of uneven temperature distribution across the glass surface. This leads to internally induced stress. Consider glass installed in the mountains or the desert. The glass temperature can drop significantly during the cold night-time hours but when the sun rises the center of the glass heats up rapidly while the glass under the frame remains cold. The resulting temperature variance may be sufficient to induce stress, which the glass cannot withstand, and it cracks. Factors that can exacerbate thermal stress can include type of glass, glass thickness, framing systems, surface or edge damage, heat absorption characteristics of the glass, edge bite, and unfavorable external shading on the glass or blockage of air flow due to close window coverings on the interior. It is possible to get some understanding of the cause of the breakage by looking at the breakage pattern on the surface of the glass. Breakage patterns which emanate from the corner of the glass may indicate a window out of square. Thermal stress fractures normally emanate from the edge of the glass, perpendicular to the edge at a 90-degree angle for the first ½ -1”, from there the crack may go in any direction. See Figure 2.3 below. For more about stress fractures, consider taking the Advanced Architectural course. 23 Figure 2.3 Glazing Systems A glazing system is comprised of the framing system, glazing materials, and the glass itself. Framing Systems The framing system serves to hold and minimize the edge deflection of the glass, keep water and air out of the building, and provide a method of cushioning as well as thermal isolation for the glass. In residential applications, most frames are wood, vinyl, or aluminum. In the case of commercial buildings, aluminum and steel are most often used. Aluminum is used extensively as it is a very diverse material that can be manufactured in a variety of shapes and is easily fabricated. The framing system should provide support to the glass and is obviously necessary to provide integrity to the facade of the building. Glazing Materials Gaskets, sealants and tapes are used to provide an effective seal, cushion the glass and provide thermal insulation between the glass and frame. In general, there are three common glazing materials. These are gaskets, sealants, and tapes. Gaskets can be made of solid or foam sections and are generally made of rubbery type materials which could include, vinyl, silicone, etc. They need to be resistant to the elements, but still be able to maintain elasticity and hardness properties. Depending on the system and design requirements, these gaskets can be keyed or wedge type. Gaskets are engineered in such a manner that they have an appropriate thickness, hardness in profile required to apply proper pressure on the glass and still take into consideration the likely glass tolerances, framing material and the overall gasket dimension. 24 Sealants may be used individually or may be combined with gaskets or tapes. Common sealants are silicones, polysulfides, polyurethanes, and other materials that can be applied with a sealant gun. Most of these materials can also be manipulated with tools to provide a designed shape. There are many factors that are taken into consideration when a decision is to be made on the type of sealant to be used. Some of these factors include the materials to be joined or sealed, environmental conditions that may prevail, thermal considerations such as expansion and contraction, as well as joint size. As the sealants have varying levels of performance and a multitude of different properties, careful consideration must be made to ensure that the appropriate sealant is used for the appropriate application. Tapes are frequently used in the design of window systems and will function in a similar manner to sealants and gaskets. These tapes normally have a rectangular cross section and can be provided in solid foam material. In some cases, they can have an adhesive on one or both faces. As the requirements of a framing system can be demanding, in many cases, tape is used as a backup to the sealant application. The tape can provide a temporary cushioning effect, while the sealant is curing or may provide a holding method prior to the application of a vinyl gasket. Window Construction Windows are part of a larger set of products described by the building industry as fenestration products. A fenestration is any opening in a building and includes windows, doors, skylights, tubular daylighting openings, etc. Windows come in many different configurations from the standard double hung found in many homes, through casements, and non-operable commercial windows. The many different configurations of window design are outside the scope of this document and the discussion will be restricted to showing the constructions using one, two, or three panes of glass. Single Pane Windows The most basic window design is a single pane of glass in a basic frame. This was once the only type of window found in almost every building around the world. These windows, while they may allow a lot of light and views, they are very energy inefficient. Something better was needed. Dual Pane Windows These windows as their name implies are built using two sheets of glass separated by an airspace of constant thickness. They are commonly referred to as insulated glass units or IGUs. Early units consisted of clear, annealed sheets of glass. Modern IGUs often use coated glass in different configurations to achieve varying energy performance standards. “Spacers” or edge seals are placed between the two sheets of glass to create the airspace. 25 Figure 2.4 The seal between the glass sheets is accomplished throughthe use of a variety of different types of materials and sealants. High performance IGUs use special technologies to minimize heat transfer through warm edge spaces, thermally improved sashes and frames, thermally broken framing, and special weatherstripping. In addition, a desiccant is incorporated into the edge seal technology to absorb water vapor that migrates across the seal. The intervening airspace reduces heat transfer by conduction and convection through the glass. In order to increase the insulating performance of the IGU, inert gases such as Argon and Krypton may be used to replace air between the panes of glass. Both Argon and Krypton are invisible, harmless, odorless, and heavier than air, which results in slower convective movement, few molecular collisions, and a reduction in heat transfer. Diagram 2.6 illustrates a dual seal IGU. One important note is how glass surface are numbered for users to identify and understand the placement of coatings. In the diagram below the faces of the glass are numbered 1-4 with the number one (1) face being the outside, number two (2) face being the inside surface of the outside pane, number three (3) being the air gap side of the inner pane, and finally the number four (4) face being the inside surface of the inner pane. Understanding the location of coatings in an IGU will be important in later sections of this guide as the location greatly impacts the solar performance and the heat transfer characteristics of a window. Figure 2.5 26 Triple Pane Windows These windows are built using three panes of glass. The third pane of glass further improves the performance of these windows by slowing the convection and conduction through the unit. Triple pane windows tend to be heavy and do not fit in a standard opening so are not very useful for retrofit applications. Newer versions use a very thin pane of glass in the middle and smaller air gaps to allow for retrofit in existing openings. IGU Seal Failure One of the most common IGU consumer complaints is “fogging”. Almost everyone has a seen a window that has turned very hazy, blocking the view and creating a very “dirty” looking window. An IGU seal failure occurs when the desiccant used to keep the gas or airspace dry between the two glass plates can no longer absorb migrating moisture or other vapors that invade the seal. This can happen because of the natural aging process of an IGU, or it can be greatly accelerated by a poor window design, which allows moisture to be trapped near the edges of the unit. Early IGUs used a single seal but most modern IGUs use a dual seal with one acting as the structural seal and the other as the moisture barrier. The result of a seal failure is the condensation of excess moisture on the inside glass surfaces of the unit. This eventually causes the IGU to have a hazy or fogged appearance. 27 Vacuum insulated glazing These windows are like dual pane IGUs but instead of sealants and air or inert gasses, the gap between the glass layers is a vacuum and the units are sealed to maintain that vacuum. VIGs have a higher performance level as there is almost no convection from one pane to the other. Newer versions have a very thin profile which allows for substitution into existing framing. With the high variety of glass types, coatings, glazing materials and window designs, there are endless options and configurations for architectural windows. The next section will describe the benefits, basic structures, manufacturing methods, solar performance properties, and the interaction with glass and window constructions for architectural Solar Control Window Films. Solar Control Window Film Introduction As glass coatings became prominent and energy became more costly, building owners and managers looked for ways to improve their existing commercial properties without the expense and disruption of replacement windows. Solar control window film fit that need. Dating back to the 1960’s reflective films made with metallized aluminum polyester coatings were offered for sale. Such early films were excellent at reflecting solar radiation back to the outside of the building and were especially popular in cooling dominant climates. As window film technology evolved additional benefits beyond solar control were discovered and promoted. Today the most widely marketed benefits for commercial buildings are solar heat control for occupant comfort, cost savings, fade protection, glare control, and exterior building aesthetic improvement. The cost of a window film installation is often justified and budgeted based on the payback in energy savings over a period. These cost savings can be calculated, and a discussion of those calculation methods are included in the Advanced Solar Control Guide. Not all building types are the same and the computer modeling software used for these calculations, called E-film, considers many factors. Big buildings often also experience hot and cold spots which can make the heating and cooling systems in a building conflict at times. Often the heat is trying to run on the east side of the building while the system is trying to cool the west side. Window film can modulate those effects making the whole system work more efficiently. Commercial buildings are often modernized by changing the look of the glass through the addition of window film, especially on clear single pane glass. 28 For residential consumers the benefits match common complaints including, rapid fade of furnishings, hot or cold spots and glare causing viewing issues with home electronics. While window film will often help mitigate the hot and cold spot issues in homes it is difficult to assign a cost savings benefit. Residential homes have a much small “window to wall” ratio meaning the surface area that window film could improve is a much smaller percentage than is found on large commercial buildings. Marketing of window film for homes often focuses on comfort from room to room or within a room rather than trying to predict an annual cost savings. While no product can prevent fading, window film does an excellent job of slowing down the fade process and helping to protect valuable furnishings and belongings. Glare protection is directly associated with the amount of light coming in the home and again, window film can do an excellent job of helping occupants see their favorite work or entertainment on their screen. Very few consumers want to change the appearance of their home from the outside and so most residential window films, unlike commercial products, are designed to be more neutral in color and less visibly reflective to not detract from the beauty and architecture of the home. Both markets may benefit from improved protection from shattered glass, but this benefit is covered in the Safety and Security Manual. The rest of this section will focus on the basic building blocks of window films, how those layers are manufactured, basic window film structures, and end with the measurements and industry standards used to describe window film performance. Basic Window Film Manufacturing Principles Window film is produced in what is termed a “conversion process”. This term applies to the process by which several different layers and raw materials are combined or “converted” into one cohesive final product. There are several raw materials used in the final structure and they may include: raw polyester film, dyed polyester, metal or metal oxide/nitride coated polyester, various performance coatings, and liner material. How these raw materials are combined or “converted” will determine the type of window film produced. No matter what the final combination, they all will require the addition of a scratch resistant coating and an installation adhesive. The five basic processes used in the production of window film are: Dyeing or coloring film Vacuum Deposition (electron beam, metallizing, sputtering) Laminating Coating Slitting 29 Manufacturers of window film will possess the ability to perform some, or all the processes shown above. To maintain the optical quality of the finished products, most of the processes will be performed in “cleanroom” environments. This simply means a sealed off area which has the incoming air filtered to remove impurities. These “rooms” are also kept at slight positive air pressure to prevent air contaminants from entering through openings. The personnel who work in clean room environments are required to wear special uniforms to prevent contamination. These processes are all very technical and must be tightly controlled to produce an acceptable product. While window film may look like a simple product that is far from the truth. Raw Materials Polyester Film Developed in the late 1920s and early 1930s, polyester film is a popular laminating substrate. Manufactured by melting polyester chips and then extruding and stretching the film in both the length and then width at high speeds, polyester film is a highly useful substrate. It is durable, tough, and highly flexible, absorbs little moisture, and has both high and low temperature resistances. It offers crystal clarity and can be pre-treated to accept different types of coatings such as adhesives. Polyester film can also be metallized and easily laminated to other layers of film. Polyester film can also be dyed, or metallized, by either vacuum coating or sputtering to produce an array of colored and spectrally selective films. Polyester film thickness is measured in the United States in “mils” which is.001 inches. In other parts of the world this same film is measured in microns with that same 1 mil film measuring 25 microns. Unless the product is going to be a safety film, standard window film widely uses 1 mil (25 micron) or ½ mil (12 micron) film. Polyester film serves as the backbone for the window film industry. Coatings and Coloration Coloration processes Color is imparted to the film in several ways. The color can be infused throughout the film either as part of coloring the PET chips before extrusion or deep dyed in an immersion process after the film is at its finished width and thickness. Additionally, the film can be surfaced coated and then the color infused into the polyester or surface coated and left on the surface as a standard coating. There are many positives and negatives to each of these processes which are outside the scope of this document. Ultraviolet UV Absorbers 30 Special ultraviolet (UV) absorbers are utilized to prevent the sun’s ultraviolet rays from breaking down the polyester film or adhesives that laminate the layers of polyester film together or bond the film to the glass. These UV absorbers can be present in either or both the adhesives, and/or be impregnated in the original polyester film. Absorbers allow window films to provide a significant degree of UV protection against fading to interior furnishings and fabrics and offer skin protection as well. These benefits are detailed later in this guide. Vacuum Deposition Metals and other metal oxides or nitrides are coated onto polyester film through processes that run in a vacuum (negative air pressure). Collectively these are known as vacuum deposition processes. There are two basic deposition process with some variations within those processes. Metallizing In simple terms, metallizing (or vapor deposition) is a process whereby a metal (almost exclusively aluminum) is applied as a coating onto clear film. Pure metal wire is continuously fed onto shallow electrically heated ‘boats”. Because the chamber containing the film and the boats has been pumped down to a very low pressure, the metal wire turns from solid to liquid and then gas at a temperature much lower than would be required at standard atmospheric pressure. This gaseous aluminum rises out of the boat much like steam out of a teapot and converts back to a solid when it hits cooled polyester film moving at a high rate of speed over the boats. The thickness of the coating is controlled by a shutter mechanism, the power to the boats, and the speed of the process. The aluminum layer deposited on the surface of the film has a very “open” porous structure. Diagram 3.8 below shows the basic elements of a vapor deposition chamber. A newer version of metallizing uses similar principles but uses an electronic beam to convert the metal to gas and can be used on metals with higher boiling points than aluminum. 31 Sputtering The basic sputtering process involves a large vacuum chamber and an inert (non-reactive) gas atmosphere as well as electrical energy. The electrical energy imparts a negative charge to the inert gas molecules. Because the atmospheric pressure is very low the negatively charged gas particles move freely and are attracted to the negative voltage underneath the material to be deposited. That material can be either in a solid flat plate or a cylindrical spinning roll both called targets. When the negatively charged particles strike the target, they dislodge metal atoms which fly at high speeds to strike the film moving over the target. The cloud of highly charged particles found between the target and the film is call the plasma. This process allows the composition of the coatings on the film to match the composition of the target material almost exactly. If a non-inert gas like oxygen is introduced to this process, it will react with the metal atoms and form oxides, which will have very different properties than the original metal. Because this process involves physical deposition of the target material it is often called a physical vapor deposition process (PVD). This allows metals with high melting points and alloys with varying melting metals to be deposited, something which would be impossible with standard vapor deposition. Scratch Resistant Coating (SRC) Manufacturers utilize numerous types of scratch resistant coatings applied to the exterior surface of the film to protect it from normal wear and tear and abuse by humans or by the natural environment. They will not protect the surface from gouges or abrasion from sharp objects or tools. Scratch resistant coatings are formulated to be either for exterior protection 32 or interior protection. Exterior coatings must withstand much harsher conditions than those formulated for interior coatings. Polyester film by itself is not scratch resistant. Window films are normally tested to ASTM D1044-94, the Test for Resistance of Transparent Plastics to Surface Abrasion. This is often referred to as the Taber Abrader Test, as this is the equipment used to perform this test. This device repeatedly abrades the surface of the film; and after a certain number of cycles, it measures the amount of haze (scratching) created by the abrader mechanism. The difference in haze before and after the test is known as the haze delta. Adhesives Window film manufacturers have developed and utilize a variety of patented adhesive formulations to adhere their films to the glass (installation adhesives) and to laminate one or more layers of polyester film together (laminating adhesives). Laminating Adhesives Laminating adhesives are typically used to bond two or more layers of film together. These layers may in turn be laminated to form a final product. Laminating adhesives can be pressure sensitive with a high degree of tack or may be a cured adhesive which makes a very permanent bond which would destroy the layers of film if they were pulled apart. The cured adhesive types tend to have a much lower coating thickness. Installation Adhesives Adhesives used to apply window film to the surface of glass fall into two main categories: Pressure Sensitive Adhesives (a.k.a. PS) Water Activated Adhesives (a.k.a. Dry Adhesives) Films utilizing either type of adhesives are installed in similar fashion, i.e., a soapy water or a proprietary solution is sprayed on both the glass surface and the film adhesive surface after the protective liner over the adhesive has been removed. The film is then positioned on the glass, cut to size, and squeegeed to remove excess water, etc. 33 The main difference between pressure sensitive and dry types of adhesives is how they bond with the glass. PS Adhesives form a “flat” mechanical bond with the surface of the glass based on pressure between film and glass. Water Activated Adhesives, on the other hand, form a chemical or molecular bond with glass. This chemical reaction makes for a very strong bond, offers excellent clarity, and generally has a greater longevity versus PS adhesives. However, should the film become damaged and need to be replaced, its removal may be more difficult. Performance Coatings IR nanoparticle coatings Many manufacturers have developed special performance coatings that impart solar properties. Some of the most common coatings combine infrared absorbing particles with an adhesive or UV cured coating. These coatings may be incorporated into the scratch coating, laminating adhesive, installation adhesive or may be coated as a separate layer. There are pros and cons to each option and discussions of those are outside the scope of this document. Please refer to the manufacturer for any questions concerning the placement of the coatings. There are also several different chemical structures for the IR nanoparticles with each having a different color, and a different degree of solar performance. All current nanoparticle solar coatings work predominantly through solar absorption and not solar reflectance. Release Liners The mounting adhesive of window films is protected by either silicone or non-silicone coated release liners. The liners are removed in the installation process. Since release liners are eventually discarded, the films used will vary in haze. To view window film samples with the highest degree of optical clarity, the release liner should be removed prior to inspection. Even then, slight irregularities on the adhesive surface may appear as visual flaws but they may disappear after bonding to glass has occurred. Basic Architectural Window Film Types and Structures There are four basic categories of architectural window films: 1. Clear 2. Nanoceramics - IR absorbing coated films 3. Reflective – Metallized films 4. Sputtered films 34 a. Neutral in color, low reflectivity metals b. Metal Nitride Ceramics c. Spectrally Selective Most window films are installed on the interior of the window but there are films designed to go specifically on the exterior of the window. Clear Film Most clear film products fall into the category of Safety Films. These films offer safety protection and some UV control to reduce fading. Safety films are generally considered to be 4- Mil and greater with thicker pressure sensitive adhesive layers to hold broken glass together after a glass breakage event. These films are considered non-reflective since they do not contain any metals to reflect solar radiation. (Note: Some safety films can exhibit color when one or more layers are dyed, or metallized, and are laminated together.) There are some thin clear UV films available that are used in specialized UV protection applications such as museums and art galleries. The diagram below shows a standard clear 4 mil safety film structure. Colored films Films that contain a coloration layer only are rarely used with architectural windows since the only heat control would be through absorption and is limited by the visible light transmission of the film. Occasionally tinted film is used in places where the only desired benefit is glare control or privacy. Very dark films with high absorptance are not recommended for many glass types and sun exposures due to the possibility of glass breakage from thermal stress. 35 Nanoceramic These films contain no metals and are considered non-reflective. Based on their VLT they can provide glare and fade control and reduce heat gain by solar absorption. The films come in a variety of colors achieved by a combination of the IR absorbing nanoparticle and the other dyed or colored films used in the structure. As these films do not contain metal, they are often less effective in terms of solar control because all heat control occurs as the result of absorptance, which is less efficient than reflectance. High absorbance can also lead to glass breakage. The diagram below shows a 70% visible light transmission window film where the IR performance coatings is part of the laminating adhesive. Most nanoceramic solar performance particles are not capable of producing a dark film using just the particles. The high visible light transmission of these films with relatively high solar absorption makes them good candidates for windows that require high visibility such as store fronts and some residential applications. Vacuum Coated Films Many films are manufactured using the previously described methods for depositing metals, oxides, and nitrides. These films have tremendous solar control properties because they can reflect significant amounts of solar radiation. They are traditionally identified as reflective, neutral, or sputtered films. There are new films that contain metal, metal oxides, metal nitrides or combinations but do not appear visually “reflective.” Reflective Films 36 These products are excellent solar control films with some dark VLT products capable of rejecting over 80% of all solar radiation. As the vacuum metallizing process can be tightly controlled, the thickness of the layer of aluminum layer can be manufactured to precise tolerances. The thicker the aluminum layer the lower the visible light transmission. In general, the lower the visible light transmission the higher the solar heat rejection and the higher the visible reflectance. The visible light transmissions usually range from a low of 15% to a high of 70%. Combining the aluminum substrate layer with a dyed film or coloration layer, instead of a clear layer, can produce various colored versions of this film (bronze, gray. etc.). They in turn may have various levels of light transmission and solar control properties, leading to a wide variety of reflective films. The diagram below shows a metallized film laminated to a colored film with a dry adhesive and liner without silicone. In this case the colored film is facing the glass which will give the film a colored appearance to the outside of the building. Other products put the dyed film to the scratch resistant coated side which reduces the interior reflectivity and is useful for buildings that are occupied at night. Sputtered Films A layer of sputtered metal is deposited on the film in a denser form than metallizing, and as such, most sputtered films will be slower drying unless the manufacturer goes through special steps to improve the porosity of the sputtered layer. There are three types of sputtered films: 37 Films featuring a metal or metal alloy, e.g. stainless steel, nickel-chromium, etc. Films featuring a metal nitride such as titanium nitride (ceramic). Films featuring a metal oxide in combination with a metal (spectrally selective). Sputtered films have excellent solar heat control properties like those that are produced by the metallizing process. Sputtering is a versatile process as several layers of different metals can be applied to a single piece of film (metal on metal layering) resulting in unique colors and higher levels of selective transmission. When comparing metallized vs sputtered products, metallized films will dry faster due to the more “open” crystalline structure produced by metallizing and will be less expensive due to the speed of the process. However, metallizing works best with a single metal since it relies solely on heating the metal until it reaches a gas state. It is difficult to metallize alloys since the metals that comprise the alloy melt and turn to gas at different temperatures. Sputtering is thus preferred for alloys or for depositing oxides or nitrides, but it has the disadvantage of slower drying due to a tighter crystalline structure and higher cost due to slower processing rates in manufacturing. Some manufacturers have special processes for improving the drying time of sputtered products. The effect of slower drying often results in a thin water layer collecting at the sputtered surface after installation which often shows up as “haze” to the consumer. This haze may take several days to clear and if a lot of water is left during installation it can pool into water pockets which can leave a permanent “water spot” even after drying. Sputtered products therefore take more care and the use of better squeegee techniques during installation. The diagram below illustrates a standard neutral sputtered film structure with a PS adhesive. 38 Some of the most interesting, sputtered films are those found in the “spectrally selective” category. These films have a sputtered “stack” of coatings as opposed to a single sputtered layer which gives the products interesting solar properties not possible with a standard sputtered layer. Exterior Films As mentioned earlier and shown in all the product structure diagrams, most window films are designed to be applied on the interior of the window. There are films that are designed for exterior installation. These films are used predominantly to change the aesthetics of a building where an interior film would not significantly change the look of the existing glass or because the glass is not accessible from the interior. Exterior film will have better solar performance as there is no glass layer to interfere with film properties. Additionally, placing the film on the exterior of a dual pane unit will generally mean lower glass breakage risk due to increased convection on the surface. Exterior films will normally have special scratch resistant coatings designed specifically for harsher exterior environments including, weather variables and higher UV-B exposure. Polyester film is not a natural exterior product. A reflective exterior film is shown in the diagram below. Note the position of the layers compared to an interior film. Window Film Performance Values and Measurements 39 There are many different combinations of technologies and materials that make up the window film architectural offering. It is important to understand the different performance values and how they are used to market and communicate the benefits of each product. In previous sections interaction of the sun with glass was explained using the reflectance, absorptance, and transmittance values for a single pane of glass. How the absorbed energy was transferred and how transmitted energy is converted to far infrared and re-radiated was also explained. It is not necessary to explain each of these values to a consumer or building manager. Calculated values which pull all this data together into a more easily communicated message are available. The most widely used in the glass, window, and window film architectural market is the Solar Heat Gain Coefficient (SHGC). Solar Heat Gain Coefficient SHGC measures how well a product blocks heat caused by sunlight. The SHGC is the fraction of incident solar radiation admitted through a window system, both directly transmitted and absorbed, then subsequently released inward. SHGC is expressed as a number between 0 and 1. The lower the SHGC, the less the solar heat transmitted. Shading Coefficient Less commonly today but still found in some documents the Shading Coefficient (SC) is the ration of solar heat gain passing through a glazing system compared to the solar heat gain that occurs under the same conditions for a clear, unshaded, window glass. The lower the number, the better the solar shading qualities of the glazing system. Shading Coefficient is only defined for the glazing portion of a window and does not consider the frame effects as a window system would do. For that reason, SHGC has become a more widely used value in the fenestration market. Total Solar Energy Rejection As with many product measurements, the most scientific measurement is often not the easiest to explain to a consumer. Many people have trouble with the concept that a lower number is a higher performing product. For that reason, the window film industry has used the Total Solar Energy Rejection (TSER) value to explain solar performance. TSER = 1- SHGC expressed as a percentage. The total solar energy minus the amount of solar energy transmitted. U-Value The U-Value (also referred to as the U-Factor) is a measurement of heat transfer due to outdoor/indoor temperature differences. It is used almost exclusively to describe the heat loss through a material. Technically the U Value represents the amount of heat passing through 40 one square foot of glass in one hour for every degree (F) temperature difference. The lower the U-Value the less heat transfers through the glazing. The previously described low emissivity value is a direct factor in achieving a low U-Value. R-Value Commonly referenced in the insulation industry, the R-Value, conversely, is a measurement that denotes a material’s ability to act as an insulator. The higher the R-Value, the less heat transfer. It is the reciprocal of the U-Value, expressed as R= 1/U-Value. British Thermal Units Most discussions of heat loss and gain in buildings use the British Thermal Unit (BTU) as the unit of measure. One BTU is the amount of heat required to raise the temperature of one pound of water by one degree (F). One ton of air-conditioning removes 12,000 BTUs per hour. For further discussion about BTUs, air-conditioning, and energy calculations please refer to the Advanced Solar Control Guide. Light to Solar Heat Gain Coefficient (LSG) LSG is the ratio of the visible light transmission to the solar heat transmission that passes through a window. It is calculated by dividing the VLT by the SHGC (VLT/SHGC). The higher the number the better it indicates how much of the transmitted solar energy is visible light, versus heat. This value is a good indicator of the trade-off in energy usage between power used to provide light in a building and the power needed to heat or cool that same building. The more natural light a glazing allows, while at the same time optimizing the energy needed for heating or cooling, the more efficient the overall building. Much of this is dependent on the climate and orientation of the building. While reducing solar radiation through windows during the summer is desirable in many cooling dominated locations, that same reduction in “free” winter heat from the sun (often called passive solar heating) is less desirable in heating dominated locations. Any LSG value over 1.00 is considered “spectrally selective”, a term applied to coatings or films which preferentially screen the sun’s energy in a way that delivers higher visible light and less solar heat gain. A previously used term, Luminous Efficacy is calculated by dividing the VLT by the Shading Coefficient. It is important to review specification sheets and understand that there is a wide range of performance values between products with the same visible light transmission. Glare Reduction Glare is a fascinating and complicated subject. Simply put, glare is the loss of visual performance when the intensity of the light in the field of vision is greater than the eyes’ ability to adapt. The wavelength of the light and other factors contributes to a person’s ability to deal with light. While not an all-inclusive measurement, comparing the VLT of a product to the 41 visible light transmission of clear glass does give a relative number by which to compare products. It may be just as easy to simply compare the VLT of two products and surmise that the darker product will give better glare protection than the lighter product. With the rapidly advancing research into blue light and other factors of glare this may be too simplistic an approach for light sensitive customers. For additional information consider studying the Advanced Architectural Guide or the Automotive Guide. Industry Standards Manufacturers generally provide dealers and distributors with individual sample sheets, or sample books of their various window film offerings. These film sample sheets provide performance specifications for the respective film type. Performance values can also be found on most manufacturers’ web sites. IWFA guidelines recommend the use of the National Fenestration Rating Council (NFRC) procedures for measurement, calculation, and reporting of these performance values. The initial measured data is usually based on 1/8-inch clear monolithic, annealed glass of a particular type. Values for other glass types may also be shown on data sheets in addition to the 1/8-inch glass but the glass type and thickness applicable for each published value should always be included in the data sheets. NFRC works in cooperation with ASTM (American Standard Test Methods) and ANSI (American National Standards Institute) to make their standards the same wherever possible. Also mentioned on some data sheets will be standards from ASHRAE (American Society of Heating, Refrigeration and Air Conditioning Engineers) which set constants for test methods around wind speed, etc. Calculations and data in areas outside the US may be particular to the region or use the ISO (International Standards Organization) test methods. It is important to note that the “solar spectrum” used in the various standards are not equal. There are at least three different “standard” solar spectrums used globally. Seasonal Differences in Sunlight In addition to differences in solar spectrums used by different standards-setting organizations, it is important to understand that sunlight will vary depending on time of year and each climate zones. In the Northern Hemisphere, the sun rises higher above the horizon in the summer than the winter. This creates seasonal differences in the directions from which sunlight is brightest on windows. In the summer the highest exposure is in the east and the West but in the winter the southern exposure is the highest as the sun rises in the southeast and sets in the southwest. The exact opposite is true in the Southern hemisphere and the differences are much smaller down to becoming non-existent at the equator. All performance values are measured with the 42 sun at 90 degrees to the window. You can imagine the complexity of calculating year around performance and energy savings with differences in building orientation and geography. Energy analysis programs take these effects into account, but specification sheets will be reported with the sun perpendicular to the glass. One might question why not measure the performance at the height of the sun’s intensity at noon, but the sun for the most part is on top of the building and there is less sunlight hitting the windows. The performance values will look better, but it is simply because there is less radiant energy hitting the windows. Peak load for utility companies in the northern hemisphere is almost always at mid to late afternoon. Matching Measurements to Benefits Commercial Today the most widely marketed benefits for commercial buildings are solar heat control for occupant comfort, cost savings, fade protection, glare control, and exterior building aesthetic improvement. 1. Occupant comfort and cost savings are mostly driven by SHGC, especially in cooling dominated climates. Consult with your manufacturer for a possible energy analysis for large buildings. 2. Fade protection is a function of UV protection, lower visible light, and to some extend by lowering the near IR directly transmitted through the glass as well. Read the additional information at the end of this section about Fade and UV. 3. Glare control is almost exclusively a function of visible light although the color of the light (wavelength dependent) can play a role with blue light being a bigger issue. 4. Exterior building aesthetics are a combination of color, reflectivity, and glass type. These decisions should be made with full glass size samples to properly judge the look from outside the building. Make sure to view the samples at different times of the day and in different weather conditions. Residential For residential consumers benefits match common complaints including rapid fade of furnishings; hot or cold spots; privacy; and glare causing viewing issues with home electronics. 1. Rapid fading of furnishing is similar to the commercial space, but often residential furnishings utilize fabrics and finishes that are more delicate than used in commercial spaces. See discussion below. 2. Hot and cold spots in a room are often associated with furniture placement in conjunction with the windows. Lowering the overall need for constant air cooling will help alleviate some of these issues. You may consider low emissivity options if the problem is feeling cold next to a window in the winter. 43 3. Privacy in homes is often an issue of lighting. Make sure to understand the exact time of day the residents expect privacy and other issues. While highly reflective films can provide significant privacy during the day, it virtually disappears once there is more light in the home than outside, such as at night. Explaining that phenomenon is important to avoiding complaints later. 4. Glare control is often challenging in a residential setting as many homeowners do not want to lower the incoming light. It is important to help them understand that the room is probably receiving more light than the occupants need and possibly causing eye fatigue and strain. Chose something with a medium light transmission in a neutral shade with low reflectivity to minimize the feeling of a big change in lighting. Ultraviolet Radiation Control Health considerations As we learned from the earlier discussion about the sun and its electromagnetic radiation, invisible ultraviolet (UV) radiation represents only about 3% of the energy being transmitted in normal sunlight. However, these are very powerful and more energetic (higher frequency) rays. There are three types of ultraviolet rays: UV-C (100 to 290 nanometers), UV-B (290 to 320 nanometers) and UV-A (320 to 380 nanometers). The earth’s atmosphere and ozone layer filter out most UV-C and a percentage of UV-B rays. UV-B causes sunburn, and prolonged exposure to it over many years has been linked to skin cancer, particularly basal and squamous cell. Glass absorbs heavily in the UVB range and screens most of those wavelengths. UV-A is now thought to cause 90% of skin aging and has been linked to melanoma since the longer wavelength of the UV-A rays penetrate deeper into the skin. “Broad-spectrum” sunscreens were developed to screen UV-B and UV-A. Early sunscreens only screened in the UV-B and allowed people to stay in the sun longer without experiencing a sunburn, thus allowing more UV-A skin damage. According to the Skin Cancer Foundation, there are about 106,000 new cases of non-invasive and 101,000 cases of invasive melanoma carcinoma and about 3.6 million basal cell and 1.8 million squamous cell skin cancers diagnosed annually in the US. There are roughly 7000 melanoma skin cancer deaths per year. Window film is designed to absorb UV-A radiation so while glass may protect from a sunburn, the addition of window film can be a significant improvement in the blocking of aging and cancer-causing UV radiation. Fading considerations Fading is a complex issue because each material has a different propensity to degrade from exposure to both normal visible sunlight and ultraviolet radiation, in addition to a host of other factors. For example, wood is extremely vulnerable to fading. Different types of hardwood floors have varying tolerance levels to fading from exposure to sunlight. Similarly, papers, inks, natural plant dyes, and natural fibers are more susceptible to fading than synthetics. While the 44 exact percentages of impact vary from item to item it can be generalized that at least five factors contribute to fading. 1. Ultraviolet radiation 2. Visible light both from the sun and artificial sources 3. Humidity and moisture 4. Dye fastness 5. Chemical vapors in the air Ultraviolet radiation is considered the harshest of the factors although the percentages will differ based on the material. Going into a museum with priceless artifacts is a good lesson in understanding fading and deterioration. The light will be extremely dim, generally it is cool, with a highly controlled humidity. Not seen is that all the lighting has extra UV radiation protection. Some exhibits require the visitor to push a button which illuminates the objects for a short period of viewing time only. Dealers are encouraged to exercise caution in this area and not oversell the products’ potential benefits in “preventing” fading. Again, no film or glazing product will totally prevent or stop fading. Window Film Application Considerations for Different Types of Glazing and Windows Wired Glass Solar control window films should not be applied to the interior surface of any exterior facing wired glass. The wire contained within the glass absorbs heat and if film is installed on the interior surface, the heat reflected out of the film greatly increases the heat absorption of the wire, leading to a higher rate of expansion. This can lead to glass breakage. Wired glass is a fire-rated safety glazing and is designed to keep the glass in place longer during a fire event. It is not an impact resistant film and has come under scrutiny in recent years as a human impact hazard as the wires can cause serious damage if a head or limb penetrates the wired glass. Patterned or Textured Glass Patterned or textured glass surfaces will not allow window film adhesive to form an adequate bond to the glass surface. In most cases, this type of glass is installed with the smooth side facing the exterior of the building in which case the use of exterior film is recommended. Plastic Glazing 45 Window film should not be applied to an acrylic or polycarbonate window unless the window film specifically designed for that purpose. These plastic glazing’s have the potential to out-gas. Out-gassing is the release of chemical components or moisture from the plastic glazing leading to bubbles between the plastic glazing and the window film. Additionally, the thermal expansion of these glazing types is significantly higher than glass but not the same as window film. This leads to expansion and contraction differences over the course of the day/night cycle often causing the film to delaminate. Also these “plastic” type glazing materials ar often soft and attempts to remove failed film will scratch them and ruin the surfaces. Glass Thickness and Size It should be noted again that the thickness of glass does increase its absorption, and subsequently adds stress. The amount of stress induced will depend on the glass type, thickness, and the type of film selected. Often thick glass is extremely expensive to purchase and to install. The profit to be made by the installation of window film in these cases rarely balances the risk. Extreme caution should be used when recommending or installing film to large panes of glass. The pressure required to squeegee the moisture out may result in breaking the glass as the area of the glass compared to the edge bite is very large. Skylights The application of window film to skylights is much more restricted versus vertical glazing systems. Installing the film on the inside often requires lifts and other expensive equipment along with the installation issues of hanging film overhead. Exterior film can also be problematic as most skylights are parallel to the roof or at a slight angle meaning rain, snow, etc. tend to pool and sit on the film unlike in a vertical application. Additionally, skylights are often annealed laminated glass which has special glass breakage considerations. Always consult the film manufacturer for special instructions and rules around the installation of film on skylights. Louvered Windows Because of the nature of the design of louvered windows, any film applied to them is likely to be exposed to the elements. Furthermore, louvered windows expose an edge of the glass and film to exterior weather which can lead to film issues along the edge. For this reason, it is recommended that exterior films be used on these windows. Glass Breakage considerations with Film All glass types except tempered glass are susceptible to thermal glass breakage if the wrong film is installed. Windows made with insulated glazing units (IGUs) require special consideration when considering film installation. These window types are more susceptible to thermal glass breakage due to a complex list of considerations but certainly can be filmed very effectively if 46 certain film selection rules are followed. Each manufacture will have information available on which films can be installed on different window types. The information required to compare film and glass compatibility will include film type and performance values (especially absorption); glass type; glass thickness; glazing type (single, dual, or triple pane units); glass coating or tinting; and orientation in the window; gas fill (if applicable); climate; altitude; and uneven shading. In general, highly-absorbing automotive type films should be avoided on architectural applications. To learn more about this complex subject please consider the Advanced Architectural Training Module. 47