Methods of Antibody Production Against Plant Viruses (Arabic PDF)
Document Details
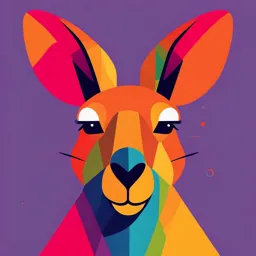
Uploaded by PatientMiracle3865
Tags
Summary
This document describes the process of producing antibodies against plant viruses. It includes steps like virus cultivation, purification, animal injection, antibody extraction, and applications in diagnostic tests, The study also covers some basic techniques in molecular biology like PCR.
Full Transcript
مراحل تولید آنتی بادی چندهمسانه ای علیه یک ویروس گیاهی مثال فرضیTMV : الف -خالص سازی پیکره های TMVطی مراحل ذیل: -1ت...
مراحل تولید آنتی بادی چندهمسانه ای علیه یک ویروس گیاهی مثال فرضیTMV : الف -خالص سازی پیکره های TMVطی مراحل ذیل: -1تکثیر ویروس روی گیاهان توتون (مایه زنی تعداد زیادی از گیاهان با ویروس) -2برداشت برگ های گیاهان آلوده ،عصاره گیری در بافر -3حذف تمام اورگانل های سلول گیاهی (شامل دیواره و غشای سلولی ،هسته ،میتوکندری ،پالست ها و ... -4تهیه آموده خالص ویروس (فقط پیکره های TMVدر تعداد بسیار زیاد). ب -تزریق آموده خالص به خرگوش در 5مرحله به فواصل یک هفته ای.در واقع پیکره های TMVنقش آنتی ژن ایفا می کند.بدن خرگوش علیه این آنتی ژن واکنش دفاعی نشان داده و تولید آنتی بادی های اختصاصی TMVخواهد کرد. ج -خونگیری از خرگوش د -حذف لخته خون (سلول های خون) و جدا کردن سرم (به این سرم که حاوی آنتی بادی های اختصاصی TMV نیز می باشد ،آنتی سرم گفته می شود) و -استخراج مولکول های آنتی بادی از آنتی سرم ه -استفاده از آنتی بادی استحصال شده در آزمون های سرولوژیکی Methods for detection antibody-virus combination 1- Double-diffusion assay (Ouchterlony method) Immunodiffussion reaction in gel IgG IgG- Enzyme conjugate (IgG-E) Enzyme: Alkaline phosphatase Substrate: p-nitrophenyl phosphate 2- enzyme linked immunosorbent assay (ELISA) 3A- Direct 3B- Indirect lateral flow immune assay (LFIA) PRIMARY PRIMARY SECONDARY ANTIBODIES CONJUGATE CONJUGATE TMV-Y Y-E CMV-Y Y-E ToMV-Y Y-E PVY-Y Y-E Y-E PVX-Y Y-E PLRV-Y Y-E LMV-Y Y-E 3. Immuno-Tissue Printing (tissue printing immunoassay, TPIA) Tissue printing has several advantages: 1. It gives detailed information on the tissue distribution of a virus; 2. Increased sensitivity. No dilution of the virus (virus has limited tissue distribution) due to extraction; 3. The technique is easily applicable to field sampling tissue blots can be made in the field and there is no need to collect leaf samples for sap extraction in the laboratory. 4- Dot blot immunoassays 2- methods depending on properties of viral NA 1- The type and molecular sizes of the virion associated nucleic acids dsRNA is a relatively good antigen. dsRNA-specific antibodies like J2 recognize structural features common to most dsRNA species. 2.The polymerase chain reaction (PCR) Polymerase chain reaction, PCR PCR a DNA photocopier History: 1971: Khorana et al., just an approach for replicating of duplex DNA using 2 primers 1983: Mullis invented PCR 1988: Saiki et al., published first paper about using Taq DNA polymerase 1993: Mullis (inventor of PCR) wins a Nobel prize PCR involves DNA replication but in the test tube DNA replication in cells need to a lot of components to replicate DNA PCR uses only basic components to replicates short fragments PCR is controlled by heating and cooling Advances in PCR: 1- thermal cycler (before this machine PCR was performed using three water bathes) 2- replacement of Klenow polymerase with thermostable DNA polymerase DNA polymerase I in bacteria, does nick translation comprises: Klenow fragment, with synthetic activity, the best activity at 37˚C, resulted in nontarget DNA products because of mispriming PCR steps: 1- denaturaion at 94˚ C 2- annealing at 40- 72˚ C 3- extension at 72˚ C For 25- 40 cycles The kinetics of PCR PCR has 3 distinct phases, 1- E, early cycles, a large number of primers copies and limited number of target sites 2- M, mid cycles, with exponential accumulation of product fragment 3- L, late cycles, amplification is suboptimal due to limiting reagents (usually DNA polym.) Basic PCR protocol A- denaturaion at 94˚ C for 5 min B- denaturaion at 94˚ C for 1 min C- annealing at 55˚ C for 1 min (repeat B-D 30 cycles) D- extension at 72˚ C for 1 min E- Final extension at 72˚ C for 10 min F- below 10˚ C forever PCR reagents: 1- PCR buffer comprises of Tris-HCl, whose pH varies between 6.8- 8.3 with temperature. Taq DNA polymerase has a higher fidelity at lower pH that occur at higher temp. KCl, assist primer/template annealing Mg2+, Taq DNA polymerase is dependent to Mg 2- primers (forward and reverse) 3- template DNA 4- dNTP (Deoxyribonucleotide triphosphate) 5- thermostable DNA polymerase 5´ Primers 3´ 3´ end of primer is very important in specificity 5´ end of primer is less important template Primer dimer Tm of primer: temperature at which half the primer annealed to target Degenerate primer DNA polymerases for PCR DNA-dependent DNA polymerases RNA-dependent DNA polymerases (RT) DNA polymerase catalyzes synthesis of DNA in 5´ → 3´ Proofreading activity: 3´→ 5´ exonuclease activity, which increase the accuracy (fidelity) 1- Klenow fragment is not thermostable, has two enzyme activities, 5´ → 3´ DNA synthesis 3´→ 5´ exonuclease activity Problems: should be added at each 37˚C DNA synthesis large amount of klenow is needed low temperature 37˚C DNA synthesis led to amplification of nontarget regions 2- Thermostable DNA polymerases Taq DNA polymerase from Thermus aquaticus, optimum temp. is 72-75 ˚C RT-PCR (reverse transcription-polymerase chain reaction) Including: 1- total RNA isolation of tissue 2- cDNA synthesis 3- PCR The seven stages of a virus infection cycle. 1 TMV Life cycle The uncoating process depends on the chemical bonds that stabilise the virus particles The early stages of uncoating do not appear to depend on preexisting or induced enzymes. The process is not host specific, at least in the early stages. the alkali treatment destabilizes the 5′-end of the rod sufficiently to allow a ribosome to attach to the 5′ leader sequence and then to move down the RNA, displacing CP subunits as it moves by a process termed cotranslational disassembly. The ribosome-partially-stripped-rod complexes wsas called “striposomes”. Ribosome meets start codon, translates first two proteins (126K ,183 K) while uncoating continues. 126 K ( MET-Hel) & 183 K ( RdRp) use viral RNA as template to make full length complementary negative strand RNA. Replicase might perform this task in a 3′→5′ direction in synthesizing the (−)-strand replication intermediate: coreplicational disassembly from the 3′-end. Thus, TMV is uncoated in a bidirectional manner 5’ cap Host Rb 5’ 4 GENOME COMPOSITION AND ORGANIZATION A. General Properties of Plant Viral Genomes - Kind of NA - Number of genome pieces (components) - Terminal structures - nt sequence - ORF (less than 7- 10 kDa, usually not been considered) - Regulatory signals - mRNAs B- Coding regions - initiation codon (AUG, AUU, CUG) - stop codon UAA>UAG>UGA C- Non-coding regions - End-group structures - 3´ and 5´ non-coding regions (NCR) - Intergenic regions Tobamovirus, type member: Tobacco mosaic virus methyl transferase motif, the helicase motif the RdRp motif I2 sg RNA CP sg RNA The function of viral coded proteins 1- structural 2- Functional Enzymes Protease (polyprotein producing viruses) Enzymes involved in nucleic acid synthesis Non-enzymatic role in RNA synthesis (VPg as primer in RNA synthesis) Coat protein of AMV: initiation of infection by the viral RNA by controlling switching between translation and priming (−)-strand synthesis. Facilitate viral movement and transmission Proteins recognizing host cells (in bacterial and animal viruses, circulative plant viruses) Proteins interacting with host defense systems 1) Polymerases that catalyze transcription of RNA from an RNA template have the general name RNA-dependent RNA polymerase (RdRp). 2) The enzyme complex that makes copies of an entire RNA genome and the sg mRNAs is called a replicase. 3) If an RdRp is found as a functional part of the virus particle as in the Rhabdoviridae and Reoviridae it is often called a transcriptase. 4) The enzyme coded by members of the Caulimoviridae, which copies a full-length viral RNA into genomic DNA, is called an RNA-dependent DNA polymerase or reverse transcriptase (RT). 5) In the Geminiviridae the viral gene product(s) associate(s) with the host DNA- dependent DNA polymerase. Economy in the Use of Genomic Nucleic Acids packed ORFs Overlapped ORFs Read-through of leaky termination codons muti-funtional proteins Functional introns in geminiviruses and mRNA splicing, may be a feature common in viruses with a DNA genome Regulatory sequence may overlap with coding sequences. e.g. signals for subgenomic RNA synthesis in TMV In the 5 ´ and 3 ´ noncoding sequences of the ssRNA viruses, may be involved in more than one function. For example, 5 ´ noncoding sequences a ribosome recognition site and complementary sequence for a replicase recognition site in the 3 ´ region of the (–) strand. ss DNA by host DdRp ds DNA by host DdRp FIGURE 6.2 Routing of viral genome expression through mRNA. Route I: transcription of dsDNA by the host DdRp route II is transcription of ssDNA to give a dsDNA template for I (e.g., geminiviruses) route III is transcription of dsRNA, usually by virus-coded RdRp (e.g., reoviruses) route IV is replication of (+)ss RNA via a (−)-strand template by virus-coded route V is transcription of (−)-strand RNA viral genome by virus-coded RdRp (e.g., tospoviruses); route VI is reverse transcription of the RNA stage of retro- and pararetroviruses leading to a dsDNA 12 template for mRNA transcription (3) production of mRNAs ss DNA by host DdRp ds DNA by host DdRp Retroviruses cycle FIGURE 6.2 Routing of viral genome expression through mRNA. Route I: transcription of dsDNA by the host DdRp route II is transcription of ssDNA to give a dsDNA template for I (e.g., geminiviruses) route III is transcription of dsRNA, usually by virus-coded RdRp (e.g., reoviruses) route IV is replication of (+)ss RNA via a (−)-strand template by virus-coded route V is transcription of (−)-strand RNA viral genome by virus-coded RdRp (e.g., tospoviruses); route VI is reverse transcription of the RNA stage of retro- and pararetroviruses leading to a dsDNA 13 template for mRNA transcription EXPRESSION OF VIRAL GENOME 14 Strategies: 1- subgenomic RNAs In: Tobamovirus, Cucumovirus, … 15 2- Polyprotein strategy In: Potyvirus, Bymovirus 16 3- Multi-partite genomes, Bromoviridae, Comoviridae are multi-component and Reoviridae, all segments are encapsidated in one particle 4. Internal Initiation translation can be initiated at a site, termed the IRES or ribosome landing pad that is not the 5′ AUG start codon. Comoviridae, Luteoviridae, Potyviridae, and Tombusviridae and the genus Tobamovirus 17 18 19 5- leaky scanning 20 leaky scaning strategy B- overlapping ORFs: ORF 3,4 PVX 6- Read-through Type I (generally UAG CAA UYA) is found in tobamovirus replicase Type II [generally UGA CGG or UGA CUA together with a 3′ structure is found in tobravirus, pecluvirus, furovirus, and pomovirus replicase Type III (generally UAG G together with a compact pseudoknot) is found possibly in luteoviruses and tombusviruses. 22 23 6- frame shift 24 For a –1 frameshift three features are needed: A- heptanucleotide sequence, termed the “slippery” or “shifty” sequence at the frameshift site B- strongly structured region downstream of the frameshift site, e.g. hairpin or psudoknot C- and a spacer of four to nine nucleotides between the slippery sequence and the structured region. The slippery sequence comprises two homopolymeric triplets of the type XXX YYY Z (X = A, G, U; Y = A, U; Z = A, C, U). Upon reaching this heptanucleotide sequence, the two ribosome- bound tRNAs that are in one reading frame (X.XXY.YYZ) shift by one nucleotide in the 5´direction (XXX.YYY.Z), retaining two out of the three base-paired nucleotides with the viral RNA. This mechanism was deduced for retroviruses, but the evidence points to a similar mechanism in plant RNA viruses. 25 26 CaMV Splicing In Caulimoviridae Geminiviridae Translation control 5´ cap without poly A tail, like TMV poly A tail without 5´ cap, like Potyviruses 5´ untranslated regions (UTR), like 67 nt of 5´ end of TMV genome Cap snatching: All negative-strand RNA viruses with segmented genomes use this mechanism. During this process a nucleotide sequence between 10 and 20 nt in size is cleaved from the 5’ end of host mRNAs by an endonuclease activity encompassed within the viral RdRp. The capped leader obtained is subsequently used to prime transcription on the viral genome, which ultimately leads to the synthesis of capped, translatable viral mRNAs. e.g. Tospoviruses, Tenuiviruses 28 29 Viral genomes differ from cellular mRNAs in two major respects: 1. The genomes of many RNA viruses lack a 5′ cap and a 3′ poly (A) tail. 2. ORFs downstream of the 5′ ORF normally remain untranslated; so effectively these ORFs are “closed.” Translation of Plant Virus Genomes 1. Cap-Independent Translation The mRNAs of many plant viruses, e.g., those in the Potyviridae, Comoviridae, Tombusviridae, and Luteoviridae families and the Sobemovirus and Umbravirus genera, lack a m7GpppG 5′ cap structure, are very efficiently translated by two basic mechanisms, sequences, and sequence structures that capture or assemble the host translation complex (CITES), and sequences that provide a “landing pad” for ribosomes (IRES). a. CITEs Cap-independent translation elements (CITEs) are found mainly in the 3′ UTRs of several viruses. b. Internal Ribosome Entry Sites (IRES) Another way to overcome the lack of a 5′ cap and also to express non-5′ ORFs is for translation to be initiated at a site, termed an internal ribosome entry site or ribosome landing pad that is not the 5′ AUG start codon. 30 Advantages of Cap-Independent Translation Cap-independent translation involves the same basic mechanism of closed loop initiation as with cap-dependent translation. Advantages of cap-independent translation: It enables the virus to compete with the host mRNAs for the translational machinery by using alternative ways to assemble and position the translational initiation complex. This can be done in a measured way so that the host is not damaged too much which would compromise the virus–host interaction. It avoids the need to encode capping enzymes. Thus, capped, (+)-strand plant viruses encode their own capping enzymes. It enables translational control of expression of viral genes 2. Expressing Potentially Closed ORFs By different strategies 31 Mechanistic models of 3′ CITE activity. the 3′ CITE interacts with the 5′-UTR via RNA–RNA base pairing (i), while bound to eIF4F (ii). This facilitates recruitment of the 43S ribosome subunit (iii), which enters at the 5′-end (iv) and scans (v), disrupting the RNA–RNA interaction (vi). When the 43S subunit reaches the start codon it is joined by the 32 60S subunit to form the 80S initiation complex (vii) and translation begins (viii). The seven stages of a virus infection cycle. 1 TMV Life cycle The uncoating process depends on the chemical bonds that stabilise the virus particles The early stages of uncoating do not appear to depend on preexisting or induced enzymes. The process is not host specific, at least in the early stages. the alkali treatment destabilizes the 5′-end of the rod sufficiently to allow a ribosome to attach to the 5′ leader sequence and then to move down the RNA, displacing CP subunits as it moves by a process termed cotranslational disassembly. The ribosome-partially-stripped-rod complexes wsas called “striposomes”. Ribosome meets start codon, translates first two proteins (126K ,183 K) while uncoating continues. 126 K ( MET-Hel) & 183 K ( RdRp) use viral RNA as template to make full length complementary negative strand RNA. Replicase might perform this task in a 3′→5′ direction in synthesizing the (−)-strand replication intermediate: coreplicational disassembly from the 3′-end. Thus, TMV is uncoated in a bidirectional manner 5’ cap Host Rb 5’ 4 GENOME COMPOSITION AND ORGANIZATION A. General Properties of Plant Viral Genomes - Kind of NA - Number of genome pieces (components) - Terminal structures - nt sequence - ORF (less than 7- 10 kDa, usually not been considered) - Regulatory signals - mRNAs B- Coding regions - initiation codon (AUG, AUU, CUG) - stop codon UAA>UAG>UGA C- Non-coding regions - End-group structures - 3´ and 5´ non-coding regions (NCR) - Intergenic regions Tobamovirus, type member: Tobacco mosaic virus methyl transferase motif, the helicase motif the RdRp motif I2 sg RNA CP sg RNA The function of viral coded proteins 1- structural 2- Functional Enzymes Protease (polyprotein producing viruses) Enzymes involved in nucleic acid synthesis Non-enzymatic role in RNA synthesis (VPg as primer in RNA synthesis) Coat protein of AMV: initiation of infection by the viral RNA by controlling switching between translation and priming (−)-strand synthesis. Facilitate viral movement and transmission Proteins recognizing host cells (in bacterial and animal viruses, circulative plant viruses) Proteins interacting with host defense systems 1) Polymerases that catalyze transcription of RNA from an RNA template have the general name RNA-dependent RNA polymerase (RdRp). 2) The enzyme complex that makes copies of an entire RNA genome and the sg mRNAs is called a replicase. 3) If an RdRp is found as a functional part of the virus particle as in the Rhabdoviridae and Reoviridae it is often called a transcriptase. 4) The enzyme coded by members of the Caulimoviridae, which copies a full-length viral RNA into genomic DNA, is called an RNA-dependent DNA polymerase or reverse transcriptase (RT). 5) In the Geminiviridae the viral gene product(s) associate(s) with the host DNA- dependent DNA polymerase. Economy in the Use of Genomic Nucleic Acids packed ORFs Overlapped ORFs Read-through of leaky termination codons muti-funtional proteins Functional introns in geminiviruses and mRNA splicing, may be a feature common in viruses with a DNA genome Regulatory sequence may overlap with coding sequences. e.g. signals for subgenomic RNA synthesis in TMV In the 5 ´ and 3 ´ noncoding sequences of the ssRNA viruses, may be involved in more than one function. For example, 5 ´ noncoding sequences a ribosome recognition site and complementary sequence for a replicase recognition site in the 3 ´ region of the (–) strand. ss DNA by host DdRp ds DNA by host DdRp FIGURE 6.2 Routing of viral genome expression through mRNA. Route I: transcription of dsDNA by the host DdRp route II is transcription of ssDNA to give a dsDNA template for I (e.g., geminiviruses) route III is transcription of dsRNA, usually by virus-coded RdRp (e.g., reoviruses) route IV is replication of (+)ss RNA via a (−)-strand template by virus-coded route V is transcription of (−)-strand RNA viral genome by virus-coded RdRp (e.g., tospoviruses); route VI is reverse transcription of the RNA stage of retro- and pararetroviruses leading to a dsDNA 12 template for mRNA transcription (3) production of mRNAs ss DNA by host DdRp ds DNA by host DdRp Retroviruses cycle FIGURE 6.2 Routing of viral genome expression through mRNA. Route I: transcription of dsDNA by the host DdRp route II is transcription of ssDNA to give a dsDNA template for I (e.g., geminiviruses) route III is transcription of dsRNA, usually by virus-coded RdRp (e.g., reoviruses) route IV is replication of (+)ss RNA via a (−)-strand template by virus-coded route V is transcription of (−)-strand RNA viral genome by virus-coded RdRp (e.g., tospoviruses); route VI is reverse transcription of the RNA stage of retro- and pararetroviruses leading to a dsDNA 13 template for mRNA transcription EXPRESSION OF VIRAL GENOME 14 Strategies: 1- subgenomic RNAs In: Tobamovirus, Cucumovirus, … 15 Bromoviridae, Cucumovirus 16 2- Polyprotein strategy In: Potyvirus, Bymovirus 17 3- Multi-partite genomes, Bromoviridae, Comoviridae are multi-component and Reoviridae, all segments are encapsidated in one particle 4. Internal Initiation translation can be initiated at a site, termed the IRES or ribosome landing pad that is not the 5′ AUG start codon. Comoviridae, Luteoviridae, Potyviridae, and Tombusviridae and the genus Tobamovirus 18 19 20 5- leaky scanning 21 6- leaky scanning strategy For example: overlapping ORFs: ORF 3,4 of PVX 7- Read-through generally UAG/ UGA followed by a 3′ structure (i.e. pseudoknot) 23 8- frame shift 24 For a –1 frameshift three features are needed: A- heptanucleotide sequence, termed the “slippery” or “shifty” sequence at the frameshift site B- strongly structured region downstream of the frameshift site, e.g. hairpin or psudoknot C- and a spacer of four to nine nucleotides between the slippery sequence and the structured region. The slippery sequence comprises two homopolymeric triplets of the type XXX YYY Z (X = A, G, U; Y = A, U; Z = A, C, U). Upon reaching this heptanucleotide sequence, the two ribosome- bound tRNAs that are in one reading frame (X.XXY.YYZ) shift by one nucleotide in the 5´direction (XXX.YYY.Z), retaining two out of the three base-paired nucleotides with the viral RNA. This mechanism was deduced for retroviruses, but the evidence points to a similar mechanism in plant RNA viruses. 25 26 CaMV 9- Splicing In Caulimoviridae Geminiviridae Translation control 5´ cap without poly A tail, like TMV poly A tail without 5´ cap, like Potyviruses 5´ untranslated regions (UTR), like 67 nt of 5´ end of TMV genome Cap snatching: All negative-strand RNA viruses with segmented genomes use this mechanism. During this process a nucleotide sequence between 10 and 20 nt in size is cleaved from the 5’ end of host mRNAs by an endonuclease activity encompassed within the viral RdRp. The capped leader obtained is subsequently used to prime transcription on the viral genome, which ultimately leads to the synthesis of capped, translatable viral mRNAs. e.g. Tospoviruses, Tenuiviruses 28 Cap snatching 29 Tobamovirus, type member: Tobacco mosaic virus methyl transferase motif, the helicase motif the RdRp motif I2 sg RNA CP sg RNA Potyvirus Type Member (PVY) Genomic Organization The genome of TEV (9,704 nt) is translated into a single polyprotein, which is subsequently processed into at least eight, and probably ten, products: P1 protein 35 kDa Proteinase HC-Pro 52 kDa helper component (insect transmission), cytoplasmic amorphous inclusion protein, Proteinase P3 protein 50 kDa May be proteinase cofactor 6K1 6.0 kDa unknown function CI 71 kDa Cytoplasmic pinwheel inclusion protein involved in RNA replication 6K2 6 kDa unknown function NIa 21 kDa VPg, Genome-linked protein 27 kDa Nuclear Inclusion proteinase NIb 58 kDa Nuclear Inclusion polymerase (RdRP) CP 30 kDa coat protein P3-PIPO pretty interesting potyvirus ORF, cell-to-cell movement P1N-PISPO Pretty Interesting Sweet potato Potyviral ORF, RNA Silencing Suppressor Potexvirus, type member: Potato virus X Genes are translated by means of subgenomic RNAs, with a total of 5 open reading frames: ORF1 166 kDa Polymerase ORF2 25 kDa ORFs 2-4 (Triple gene block) translated from different ORF3 12 kDa reading frames and all involved in cell-to-cell movement, TGB1 is also a suppressor of RNA silencing ORF4 8 kDa ORF5 25 kDa Coat protein Rhabdoviridae, Nucleorhabdovirus, Potato yellow dwarf virus Monopartite, linear, single-stranded, negative sense RNA, 11000-15000 nucleotides long. 1 N 54 kDa Coat protein (nucleocapsid) 2 P 38 kDa Phosphoprotein 3 3,Sc4, 4b 37 kDa Movement protein 4 M 32 kDa Matrix protein 5 G 70 kDa Glycoprotein 6 L 241 kDa Polymerase The complex then transcribes the mRNA for the N protein, which is capped during synthesis by the polymerase. At the end of the N gene, and of all genes, is the sequence 5'-AGUUUUUUU-3‘ (element I) which signals termination and polyadenylation of the mRNA. This intergenic sequence also comprises a short untranscribed sequence (element II) and the start site for transcription of the next mRNA (element III) 6 Tospoviridae, Orthotospovirus type member: Tomato spotted wilt virus Genome Linear, tripartite ssRNA: L (c.8900 nt), M (c.4800 nt) and S (c.2900 nt). Genomic nucleic acid is negative sense (RNA-L), or ambisense (RNA-M and RNA-S). Nucleotide sequences of 3'-termini are complementary to similar regions on the 5' end, thus forming a panhandle Translation of the RNA is believed to produce the following products: ORF Segment complementary Size Putative function 1 RNA-L complementary 330 kDa RNA polymerase 2 RNA-M viral 34 kDa NSM cell-to-cell movement 3 RNA-M complementary 127 kDa precursor of glycoproteins G1 (75 kDa) and G2 (46 kDa) 4 RNA-S viral 52 kDa NSS suppressor of RNA silencing 5 RNA-S complementary 29 kDa N, nucleoprotein Ambisense Expression Strategy (Nucleoprotein) In Orthotospovirus (unknown) (movement} (RNA polymerase) 8 Reoviridae; Phytoreovirus; Rice dwarf virus Linear, double stranded RNA in 12 segments, have all their multipartite genome segments in one particle. Each virion contains a single copy of the entire genome. The 3' terminus has neither a poly(A) tract nor a tRNA-like structure. Conserved nucleotide sequences occur at the 3'-terminus and at the 5'-terminus of each gene segment. The products encoded are as follows: FAMILY: NANOVIRIDAE Genus: Nanovirus genomes segmented into 6 to 8 segments of ∼1 kb each. Each segment is encapsidated as a single copy into individual icosahedral virus particles 18 to 20 nm in diameter. DNA-R, Master replication initiation protein for all genomic DNAs (M-rep) DNA-S, coat protein DNA-C, cell cycle regulation (Clink) DNA-M, movement protein DNA-N, nuclear shuttle protein DNA-U1, DNA-U2, and DNA-U4, unknown. Caulimoviridae; Caulimovirus; Cauliflower mosaic virus Genome Monopartite, open circular, double stranded DNA of 7800-8200 base pairs. Genome has discontinuities in both strands: one in the transcribed (alpha- or minus-) strand and one to three in the non-transcribed strand. Type Member Genomic Organization There are believed to be 7 open reading frames: 1 38 kDa Movement protein 2 18 kDa aphid transmission 3 15 kDa virion-associated protein 4 57 kDa Coat protein (precursor of 42 kDa protein subunit of the virion shell) 5 79 kDa replication polyprotein processed to give an aspartate protease, reverse transcriptase (RT), and RNase H 6 58 kDa Translational enhancer, suppressor of RNA silencing. 7 11 kDa Unknown function VII Geminiviridae; Begomovirus; Bean golden yellow mosaic virus Genome Usually bipartite, closed circular, single stranded DNA. Both DNAs are required for full systemic infection. Genus Genomic Organization ORFs occur on both genome segments (if present) and in both the virion (+) and complementary (-) senses. Component A has 6 open reading frames, 2 in the virion sense and 4 in the complementary sense. Component B always has two open reading frames, one each in the virion and complementary sense, encoding products involved in virus spread within plants. AC4: possibly determining symptom expression Nuclear shuttle protein transcriptional activator protein (TrAP) Replication enhancer 13 Virus Replication Replicative form (RF) Semiconservative RF conservative RF 14 15 The begomovirus life cycle The caulimovirus life cycle Transmission of plant viruses 1-mechanical 2-by seed Cryptovirus Comovirus Sobemovirus Potyvirus Nepovirus Ilarvirus 3- by pollen Nepovirus Ilarvirus Sobemovirus 4- by vegetative propagation 5- by grafting 6- by dodder 2- Seed transmission Seed transmission is known to occur for about 25% of the known viruses. A- seed-borne: viral inoculum located outside the embryo Tobamoviruses: Cucumber green mottle mosaic virus (CGMMV) and Tomato mosaic virus (ToMV) Sobemovirus: Southern bean mosaic virus (SBMV) B- seed-transmitted: viral inoculum located inside the embryo Bean common mosaic virus, Bean yellow mosaic virus, Lettuce mosaic virus viruses can infect the developing embryo by 2 ways B-1- Direct embryo invasion, i.e. after fertilization. B- 2- indirect invasion, i.e. by infection of the gametes before fertilization Percentage of seed transmission depends on: 1- virus 2- host 3- Time of mother plant infection Indirect Direct 3- by pollen TRANSMISSION BY INVERTEBRATES A. Arthropoda – 1. Insecta – 2- Arachnida Tetranychidae Eriophyidae B- Nematoda Insecta: over 80 % of plant viruses are dependent upon insect vectors for their spread. 549 Acquisition phase Acquisition feed Latent period Inoculation feed Retention period (retention of virus after moulting) Figure 5. Schematic showing how the retention and movement of plant viruses leads to classification of transmission mode, Whitfield et al. 2015 , (Figure 1). In this representation, the classification is made in terms of: A stylet retention (elsewhere described as non-persistent), B foregut retention (semi- persistent), and C circulative movement (including both persistent- circulative and persistent- propagative https://doi.org/10.3390/pla nts9121768 Non-persistent Alfamovirus, Caulimovirus (by M. persicae), Cucumovirus, Fabavirus, Macluravirus and Potyvirus. Fasting has positive effects on transmission DAG (aspartic acid-alanine-glycine) PKT (proline-lysine-threonine) KITC (lysine- isoleucine-threonine -cysteine) Model of the ingestion-salivation mechanism of non-circulative, non-persistent transmission. Gray S M , and Banerjee N Microbiol. Mol. Biol. Rev. 1999;63:128-148 Semi-persistent transmission Caulimovirus, Closterovirus Fig. 11.10 (see Plate 11.1} Model of the sequential acquisition of CaMV by aphids from infected cells. (A) electron-lucent inclusion bodies (eliB) and electron- dense inclusion bodies (ediB). (B) (B) After salivation (C) While the liberated P3 is ingested, the released P2, perhaps together with a few virus:P3 complexes from the eliB, attaches to the aphid stylet cuticle. The aphid is now 'P2 loaded', and thus transmission competent, and ready to acquire more P3:virions (solid circles) from either the same cell or (D) on following feeding on other cells from the same or different host plants. Virion, CP eliB (P2) ediB (P3) CaMV Bimodal transmission a virus could apparently be transmitted both non-persistently and semi-persistently by the same aphid species. CaMV Persistent transmission Non-propagative Nanovirus, Umbravirus, Luteovirus Propagative Rhabdoviridae (coated pit) (apical plasmalemma) (coated vesicles) (Receptosom) (basal plasmalemma) receptor-mediated endocytosis as a mechanism regulating vector-specific luteovirus acquisition. Based on this model, luteoviruses recognized at the gut cell apical plasmalemma (APL) bind to the membrane (1) initiating virus invagination (2) into coated pits (CoP) Coated pits bud off the APL as virus-containing coated vesicles (CV), which transport the virus (3) to larger uncoated vesicles, called receptosomes (RS) (4). Tubular vesicles containing linear aggregates of virus, form on the receptosomes (5) and transport the virus to the basal plasmalemma (BPL). Tubular vesicles fuse with the BPL (6). Luteoviruses can then diffuse through the gut basal lamina (BL) and into the hemocoel (7). Eventually, receptosomes (endosomes) mature into lysosomes (L), and any virus particles remaining in the lysosome are probably degraded. MT, microtubules. Effects of Bacterial Symbionts on Plant Virus Circulation in Insect Bodies FIGURE 1. Schematic diagram. Finally, virions enter into salivary glands to be horizontally transmitted to healthy plants or transfer into the reproductive system, thus being vertically transmitted to offspring. (B,C) In horizontal transmission, Rickettsia in the midgut lumen can benefit geminivirus acquisition, retention, and transmission in the insect (B). Here, it should be noticed that GroEL-begomovirus/- luteovirus interaction is essential for the stability of the virus in hemolymph, otherwise the insect immune system may recognize and degrade these foreign invaders (C). (D) During the process of vertical transmission, Reovirus virions migrate to the ovaries and enter th eggs by hitchhiking on the envelopes of Sulcia and Nasuia, and they benefit from the vertical transmission routes taken by the two obligate bacterial symbiont partners in females. Figure 7. Schematic representation of the attract and deter host plant phenotype, Carr et al. 2020 , (Figure 1). In some non-persistently transmitted viruses, an infected plant gives off Volatile Organic Compounds which attract the vector (in this representation an aphid) to land and probe epidermal cells. However, virus infection may result in plant chemicals which deter the vector from settling and feeding, with the vector now potentially having acquired virus from the initial probing moving on to potentially inoculate a healthy plant. https://doi.org/10.3390/plants9121768 Plant–virus–vector interactions in non-persistent, semi-persistent, and persistent transmission Fig. 3. Molecular mechanisms of plant-virus-vector interactions in persistent transmission A. βC1 protein of TYLCCNV interacts with host plant MYC2 proteins to suppress terpene synthesis, thereby increasing the preference of its vector, B. tabaci; B. NSs proteins in TSWV interact with host plant MYC2 proteins to suppress the biosynthesis of plant volatile monoterpenes, thereby increasing the preference of its vector, F. occidentalis; C. C2 proteins in TYLCV interact with plant ubiquitin to inhibit JA defense responses, and the reduced JA levels result in increased neophytadiene concentrations, thereby inducing the preference and performance of B. tabaci. https://doi.org/10.1016/j.hpj.2021.04.006 Figure 12.17- Two possible routes for plant rhabdovirus movement in a leafhopper vector. Virions are acquired from plant cells through the food canal inside the stylets and enter the lumen of the filter chamber and midgut. The virus then replicates in the midgut epithelial cells and later invades the principal salivary glands moving through the hemolymph (brown arrow) and/or the nervous system (blue arrows). Whiteflies (Aleyrodidae) 1- circulative: Begomovirus by Bemisia tabaci 2- semipersistent: Clestroviridae, Crinivirus by Bemisia and Trialeuroides Potyviridae, Ipomovirus by Bemisia tabaci 3- Nonpersistent Betaflexiviridae, Carlavirus by Bemisia tabaci Thrips (Thysanoptera) Orthotospovirus circulative, propagative Machlomovirus, MCMV, is transmitted in a semipersistent manner Ilarvirus, Carmovirus, Sobemovirus by pollen via action of pollinating insect eg. thrips Mealybugs (Coccoidea and Pseudococcoidea ) semipersistent 1- Cauliomoviridae, Badnavirus 2- Closteroviridae, Closterovirus 3- Betaflexiviridae, Vitivirus Beetles Comovirus Bromovirus Tymovirus Sebemovirus Mites Eriophyidae, (Subclass Acari) includes eleven species of mites that serve as vectors of several plant viruse ) Potyviridae, Tritimovirus Wheat streak mosaic virus (WSMV) by Aceria tosichella (WSMV replicates in mites) Persistent, circulative Potyviridae, Rymovirus Ryegrass mosaic virus by Abacarus hystrix Emaravirus Fig mosaic virus by Aceria ficus Alphaflexiviridae, Allexivirus Shallot virus X by Aceria tulipae Betaflexiviridae, Trichovirus, Peach mosaic virus by Eriophyes Cilevirus, Citrus leprosis virus C by Tenuipalpidae, circulative non-propagative Chromista Plasmodiophoridae Polymyxa betae, in vivo virus-vector relationship Polymyxa graminis Spongospora subterranean Polymyxa and Spongospora, transmit rod-shaped or filamentous viruses Benyvirus, Bymovirus, Furovirus and Varicosavirus Fungi Chytridiomycota Olpidium transmit viruses with isometric particles, in vitro virus-vector relationship Tombusviridae and two Olpidium species Olpidium brassicae Tomato bushy stunt virus Nematodes Nepovirus by Xiphinema and Longidorus Tobravirus by Trichodorus and Paratrichidorus Localization of viruses within nematode vectors پایان این درس viruses can infect the developing embryo by 2 ways 1- Direct embryo invasion, i.e. after fertilization. 2- indirect invasion, i.e. by infection of the gametes before fertilization Percentage of seed transmission depends on: 1- virus 2- viral determinants 3- host 4- Time of mother plant infection 5- age of infected seed seed-transmissible viruses properties: 1. (a) Most viruses are readily sap-transmissible, indicating an ability to invade parenchymatous tissue. (b) Viruses transmitted by certain types of vectors are more often seed-transmitted than those transmitted by other types of vectors 3. The earlier the mother plant becomes infected, the higher the level of seed transmission 6. The distribution of virus-infected seeds in infected mother plants are completely random suspensor middle cell; suspensor columnar cells embryonic sheath endosperm cytoplasm micropyle pocket micropyle E, embryo; Ec, endosperm cytoplasm; ES, embryonic sheath; F, funiculus; M, micropyle; MP, micropyle pocket; Sm, suspensor middle cell; Sc, suspensor columnar cells; T, testa; V, main vascular strand; Vp, peripheral vascular strands the virus uses the embryonic suspensor as the route for the direct invasion of the embryo. Important features: Virus isolate or strain host cultivar labium mandibles and maxillary