Enzyme_catalysis_M2P_202425_revised.pptx
Document Details
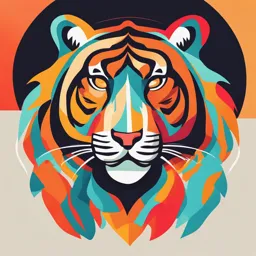
Uploaded by ConvincingFluorite
Tags
Full Transcript
BIOCHEMISTRY OF ENZYMES AND CATALYSIS M2P Fall 2024 Wendy S. Innis, Ph.D. [email protected] Session Objectives Explain how an enzyme functions as a catalyst in lowering the activation energy of reactions Define...
BIOCHEMISTRY OF ENZYMES AND CATALYSIS M2P Fall 2024 Wendy S. Innis, Ph.D. [email protected] Session Objectives Explain how an enzyme functions as a catalyst in lowering the activation energy of reactions Define and describe catalytic power, specificity, affinity, regulation, turnover number, isozymes and zymogen/proenzyme Define the term transition state and propose a role for the transition state in lowering activation energy. Explain basic catalytic mechanisms, including metal ion, covalent and acid-base catalysis Review the induced fit model of enzyme catalysis. Identify factors that inactivate enzyme activity. Compare and contrast reversible and irreversible enzyme inhibitors Describe the major enzyme classifications including oxidoreductases, transferases, hydrolases, lyases, isomerases, and ligases. Enzymes have active sites substrates bind and are held in proper proximity and orientation for reaction to occur different types of inhibitors alter enzyme activity Big Picture Small amount of enzyme can catalyze large amount of substrate (S) to product (P) For the simplest reaction catalyzed by an enzyme [E]), the observed reaction rate is described by Michaelis-Mention equation (Enzyme kinetics session) What is a catalyst? 3 ways to increase rate of rxn in any chemical reaction: Increasing temp (adding energy in form of heat) Temp regulation important for organisms—only thrive within relatively narrow temp ranges Increasing conc of reacting substrate—increases probability of reactant collisions, and in correct orientation Adding a catalyst—participates in rxn but not consumed or changed Enzymes are catalysts for chemical rxns in living systems Most enzymes are proteins Act under mild conditions Rate enhancements of 108 to 1012 are typical (cat rate/uncat rate) Have rxn specificity for reactants and products Based on functional groups in active site Enzymes differ from nonbiological catalysts in that activities are regulated—allows response to changing conditions; follow genetically determined developmental programs Reaction coordinate diagrams-- Catalyst provides a reaction pathway with a lower activation energy barrier—interacts with reactants to create and stabilize a transition state E lowers height of activation energy barrier (ΔG) by lowering the energy of transition state Theory of catalysis Enzymes increase the rate of a reaction by decreasing the activation energy Enzymes DO NOT get consumed in the reaction affect the thermodynamic stability of substrate or product affect the free energy change of the reaction (∆G) Catalytic power (rxn rate) Enzyme–substrate complex forms in the active catalytic site The rate of anhydride formation from esters and carboxylates shows a strong dependence on proximity of two reactive groups. The rate increases even more if the translational and rotational motion of reacting bonds is restricted. Enzyme-substrate complex Induced-fit model active site changes conformation (shape) when substrate binds enzyme requires protein flexibility facilitated by small negative ∆G of folded state relative to unfolded state Requires close interaction between E and S typically used to describe substrate- binding behavior Transition state complex Energy necessary to form the transition-state complex Energy barrier known as activation energy (Ea) Enzyme binds the transition state more tightly than the natural substrate; TS form is stabilized S is strained toward structures of product TSstabilization is accomplished through the close complementarity in shape and charge between active site and transition state Exhibitenzyme affinities 102-106 times greater than with only corresponding natural substrates ILLUSTRATIO N OF TRANSITION STATE STABILIZATIO N CONCEPT Active site microenvironment promotes catalysis Efficient catalysis depends on proximity and Acetoacetate orientation—E brings reacting groups into close proximity to increase the frequency of collisions and decarboxylase optimal orientation Charged/polar amino acid side chains near reactive functional group can alter pKa or reactivity Functional groups can be significantly more reactive within a hydrophobic microenvironment compared to their behavior in a polar solvent Solvent molecules excluded and functional groups desolvated Acetoacetate decarboxylase: pKa value of lys 115 in active site is unusually low—due to environment created by other residues in the site Lys115 is positioned in a hydrophobic pocket that lowers its pKa to 5.9 (normally ≈ 10.5!!) Enzyme specificity Enzyme specificity for S results from 3D arrangement of specific amino acid Active site of residues that form binding site chymotrypsin All of these enz catalyze proteolytic rxns with similar mechanisms, but have different S specificities for aa residue on N-term side of scissile peptide bond Chymotrypsin: aromatic or bulky nonpolar side chain Trypsin: Lys or Arg Elastase: smaller & uncharged side chains Neg- Hydrophobi Small, charged c pocket hydrophobic pocket pocket Zymogens Chymotrypsinogen and trypsinogen are proenzymes (zymogens) Requires biochemical change – proteolysis (covalent modification) for activation Sometimes autoactivation (e.g., Factor XII in clotting cascade) Table 8.1 Enzymes employ FUNCTION OF AMINO ACID ENZYME EXAMPLE different catalytic Covalent intermediates Glyceraldehyde 3-phosphate strategies Cysteine–SH Acid-base catalysis dehydrogenase Acetylcholinesterase, Serine–OH chymotrypsin Covalent catalysis Lysine–NH2 Aldolase Electrostatic catalysis Histidine–NH Phosphoglucomutase Metal ion catalysis Acid–base catalysis Catalysis by approximation => Histidine–NH Chymotrypsin proximity and orientation Aspartate–COOH Pepsin Cofactor catalysis Stabilization of anion formed during the reaction Peptide backbone–NH Chymotrypsin Arginine–NH Carboxypeptidase A Serine–OH Alcohol dehydrogenase Acid-base catalysis Functional group on the enzyme either donates a proton (acid catalysis) or accepts a proton (general base catalysis) during the reaction Side chains can be reactive in protonated Histidine and unprotonated generally form participates in acid-base enzyme catalysis Chymotrypsin combines catalytic strategies General Acid/ base catalysis Ser provides nucleophile Covalent catalysis His base catalyst Asp stabilizes protonated His Chymotrypsin catalysis His extracts H+ Catalytic triad Ser attacks carbonyl Peptide bond breaks Substrate His acid catalyst Covalent intermediate His base catalyst Enzyme regenerated! Lysozyme cleaves glycosidic bonds in peptidoglycan, which is Covalent catalysis the major component of gram- positive bacterial cell wall. Substrate is covalently linked during the reaction to active site of the enzyme. A nucleophile at the active site displaces a leaving group on the substrate H2O 2 Electrostatic catalysis Notalways listed separately since involved in many other aspects of catalytic mechanisms Examples: Providinglower dielectric constant of the environment of the active site (hydrophobic environment) Altering pKa values of specific functional groups Stabilizing a particular conformation of critical groups in active site by electrostatic interactions Stabilizing (binding) a charged intermediate or transition state by providing an oppositely charged enzyme group nearby Rxn: CO2 and H2O converted to Carbonic Metal ion catalysis bicarbonate and protons anhydrase Zn2+ coordinates with a water molecule and by further polarizing the H-O bond increases Enzyme requires metal ion as the acidity of the cofactor (metalloenzyme) bound water Metals provide catalytic and structural functions Coordinated via interactions with amino acid side chains Serve as electrophiles Mediate oxidation-reduction rxns or promote reactivity of other groups in E active site through stabilize electrostatic effects developing anions Factors that affect enzyme activity pH AA side chains in the active site may act as weak acids and bases with critical functions that depend on a specific ionization state. Temperature [Substrate] Conformation Enzyme inhibitors Compounds that decrease the rate of an enzymatic reaction Reversible inhibitors not covalently bound to the enzyme and can dissociate at a significant rate... more in enzyme kinetics session Irreversible inhibitors form irreversible covalent bonds in active site Covalent inhibition when a tight covalent bond forms in active site Transition state analogs mimic transition state and bind more tightly than substrates Suicide inhibition when enzyme converts the inhibitor into a reactive form Examples of these 3 types of irreversible inhibitors found on next slide Irreversible inhibition Transition state analog Covalent Suicide Covalently binds active site Covalent inhibition—rxn typical of nerve gases (organophosphates), like sarin—binds to hydroxyl of ser in active site of enzyme Acetylcholine is a neurotransmitter, failure to break it down by acetylcholinesterase results in muscle paralysis, respiratory failure, increased respiratory secretions, seizures, coma and death Suicide inhibitor—allopurinol is med that decreases production of urate; accumulation of urate (in high conc will form needle-shaped crystals in joints) in gout Compare these panels: B shows normal pathway, notice reactive part marked in green. Interestingly, the E utilitzes molybdenum (Mo) as a cofactor. See the complex that would form between the oxidase enzyme and the xanthine substrate Now look at panel C—a similar part of allopurinol is in green; once the allopurinol substrate binds to the enzyme site, that bond is irreversible, and the enz is left inactive Transition state analog: penicillin is a good inhibitor of this peptidase, as it binds with the –OH on a ser residue to create an analog of the transition state— which is not released by E, so left inactive Heavy metal toxicity Carbonic anhydrases are especially affected by heavy React w/ sulfhydryl groups in active site metals – catalyze reversible Mimic rxn of CO2 and H2O and replace normal metal ions Lead mimics calcium and is part one of its toxicity mechanisms While you’ve seen how metals participate as cofactors from the lecture on “Cofactors and Coenzymes”, also have to account for the toxic effects of high levels of some metals (such as heavy metals). Many heavy metals (thinking of mercury, lead, chromium, cadium, and arsenic) bind to sulfhydryls (from cys) in the E active site, rendering the active site “inactive” We’ve looked at carbonic anhydrase ( very common metalloenzyme) already, but now pay especial attention to the participation of Zn+2 in the reaction sequence where CO2 is hydrated to produce bicarbonate and H+: Zn+2 plays an essential role; but if it is replaced by other metals, the rxn sequence will not proceed Metal ions similar to Zn+2 can be substituted in lab experiments (Co+2, Ni+2, and Cu+2—all are divalent transition-metal ions), and drastic changes are seen in carbonic acid anhydrase activity On the next slide are examples of metals that are toxic are high conc, organs which experience toxicity, and the causes of the toxicity at a molecular level No need to memorize these—note the elements involved and the potential for significant damage The next two slides show a problem which will require understanding of the previous material and articulate the terms which can be applied to elucidate of the reaction catalyzed by carbonic anhydrase Here is a reaction catalyzed by carbonic anhydrase, an enzyme present in red blood cells. The active site of the enzyme shown below, facilitates the generation of OH-. The three histidine residues on the left bind an important Zn2+ ion. Describe the different ways this Zn2+ ion catalyzes the reaction mechanism. Ways in which the Zn2+ ion catalyzes the reaction mechanism: Proximity and orientation effects: The Zn2+ ion positions the water molecule correctly oriented in the active site of the enzyme in close proximity of the histidine residue, which increases both the collision frequency of the reactants and the probability that reactants collide in the right orientation. Increasing electrophilicity: The Zn2+ ion places positive charge next to the oxygen of H2O, which makes the hydrogen atom a better electrophile. Stabilizing transition state: The positive charge of the Zn2+ ion also stabilizes the formation of the negative charge on the oxygen of H2O and thus stabilizes the transition state, lowering the activation energy. The final slide shows how enzymes can be classified into group based on the type of reaction that is catalyzed. You need to know each of these enzyme classes You need to know/understand the description of activity performed by each class You should be able to examine a simple reaction, look at the changes being made in substrates to form products, and identify which enzyme class is involved The great thing is the the names used for enzymes will reflect the type of reaction that is catalyzed—see examples provided International Classification of Enzymes Enzyme class Activity Oxidoreductase Catalyze oxidation-reduction reactions s Transferases Catalyze transfer of a functional group from one molecule to another Hydrolases Catalyze hydrolysis (cleavage) of bonds by adding water in the form of OH and H to atoms forming the bond Lyases Catalyze cleavage of bonds by means other than hydrolysis or oxidation; usually require carbonyl Isomerases Catalyze rearrangement of existing atoms of a molecule Ligases Catalyze synthesis of bonds in reactions coupled with cleavage of high energy phosphate bond