Protein-Based Diagnostics PDF
Document Details
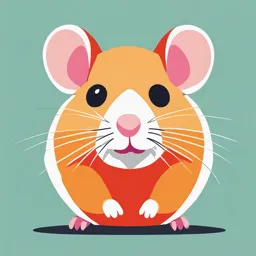
Uploaded by MagicBixbite
Tags
Related
- Engineering Protein-Based Therapeutics PDF
- Pharmacology Lecture 2024 PDF
- Beliefs Underlying Older Adults' Intention to Consume Plant-Based Protein Foods PDF
- Cardiac Amyloidosis Imaging, Part 1: Amyloidosis Etiology and Image Acquisition PDF
- Bio Inorganic Ferritin, Hemoglobin, and Hemerythrin PDF
- Cirrhotic Liver Disease & Ascites PDF
Summary
This document provides a comprehensive overview of protein-based diagnostics, covering topics such as antibody technology, enzyme-linked immunosorbent assays (ELISAs), and chromatography techniques. It also details the practical application of these technologies in various diagnostics, including the detection of biomarkers for disease.
Full Transcript
PROPRIETARY. DO NOT SHARE. Transcript: How Diagnostic Works Protein-Based Diagnostics Section 1: Defining Protein-Based Diagnostics Welcome Welcome to our course on Protein-Based Diagnostics. This course will be broken into 6 sections, and each of these sections will discuss a different technology...
PROPRIETARY. DO NOT SHARE. Transcript: How Diagnostic Works Protein-Based Diagnostics Section 1: Defining Protein-Based Diagnostics Welcome Welcome to our course on Protein-Based Diagnostics. This course will be broken into 6 sections, and each of these sections will discuss a different technology involving protein-based diagnostics. Section 1: Defining Protein-Based Diagnostics Objectives At the end of the course, you should be able to: ● Explain what an antibody is. ● Describe an enzyme-linked immunosorbent assay, also known as an ELISA. ● Describe the adaptation of an ELISA to high throughput techniques, including multiplexed bead assays. ● Describe lateral flow. ● Describe column chromatography. ● Explain how column chromatography can be used in diagnostic applications. Protein-Based Diagnostics: Biomarkers Protein-based diagnostics detect the presence or absence of a protein, and sometimes how much of that protein is present. So, we're looking for proteins as biomarkers. We frequently use antibodies to detect our protein biomarkers. Remember, a biomarker is a measurable characteristic that we find in a fluid, usually blood, but it could be urine or saliva, that reflects the severity or presence of a disease state. Protein-Based Diagnostics: Examples Frequently, we detect a protein as our biomarker. So, our example here is the C-reactive protein, CRP, as an indicator of inflammation. PSA, the prostate-specific antigen is the detection of a tumor-specific protein, or our HER-2 detection of a tumor-specific protein if we do a biopsy. Again, most of these proteins are detected using antibodies. 1 Copyright 2024 Biotech Primer, Inc. PROPRIETARY. DO NOT SHARE. Section 2: Antibody Technology What are Antibodies? Antibodies are also proteins made by the B cells of your immune system. Antibodies are proteins that recognize and bind or stick to other proteins. Antibodies have this characteristic Y-shape. If you look at this antibody, you'll see that it is composed of four units, two smaller units, and two longer units. And so, the small units are called the light chains, the longer units are called the heavy chains. The antibody is symmetrical. So, if you split it down the middle, it would be identical on each side. Variable Region Layer At the top of the antibody, we have what is known as the variable region. The variable region is the part of the antibody that recognizes and binds to other proteins. The variable region is the action part of the antibody. Constant Region Layer At the bottom, we have what's called the constant region. The constant region is part of the antibody that remains constant for every single antibody within the species, which allows a person's immune system to recognize that we do have an antibody protein present. What Do Antibodies Recognize? Different antibodies can recognize different proteins that have different variable regions. The variable region, again, changes from one antibody to the next because it is the variable region that allows an antibody to recognize another protein. The constant region remains unchanged between antibodies. Human Antibodies Humans have the same constant region for every antibody, but each of our antibodies will have different variable regions. For example, one antibody may have a variable region that will recognize this year's flu. We will make another antibody, having the same constant region, but a different variable region that will allow us to recognize next year's flu, the West Nile virus, HIV, or whatever else we may be exposed to. 2 Copyright 2024 Biotech Primer, Inc. PROPRIETARY. DO NOT SHARE. Antibodies are Specific Antibodies, again, are proteins, but they are highly specific for their target, which is usually another protein. So again, an antibody is a protein that recognizes another protein, and not only recognizes it, but it also binds or sticks to that other protein. Why are Antibodies Useful as Detection Reagents? With a little bit of chemistry, we can then turn our antibody into a quantitative detection reagent. We do that usually by adding a dye molecule or some other marker molecule to the constant region of the antibody that allows us to visualize it. Antibodies are relatively stable, meaning they don't denature, they don't unfold, and they retain their structure and function for relatively long periods. It is easy to produce antibodies on a large scale, especially for research or diagnostic purposes. There are lots of different things that we can do with antibodies as you'll see in the next sets of slides. Antibodies are Specific to a Protein Epitope We want to emphasize again the importance of how specific antibodies are, click on each tab to learn more. Layer 1 Antibodies recognize a specific protein. For each type of protein, we have a specific antibody. So, for each year's flu virus, for example, we make a new antibody to recognize that flu virus. Layer 2 The part of a protein that the antibody recognizes is known as an epitope. An epitope is just a very small piece of a protein, but it has a unique feature or features that allow it to be recognized by the antibody. This unique feature of feature is a specific amino acid sequence. So, the variable region of the antibody recognizes a specific amino acid sequence on a protein. The sequence of amino acids that the antibody recognizes on a protein is known as the epitope. Different proteins have different epitopes. 3 Copyright 2024 Biotech Primer, Inc. PROPRIETARY. DO NOT SHARE. Layer 3 The epitope changes, for example, each year for the flu virus. The antibody recognizes and binds to that epitope, and you notice that the second protein we have here doesn't have that epitope, and therefore, the antibody will not bind to it. Different antibodies recognize different epitopes on the same antigen. What we're trying to say here is that a protein such as a flu-virus protein or a protein on a cancer cell may have several different epitopes. Remember that a protein can be made up of hundreds to a couple thousand amino acids. Different Antibodies Recognize Different Epitopes on the Same Antigen Therefore, it makes sense that there may be more than one unique amino acid sequence on a protein. Each of those unique amino acid sequences makes up a different epitope. We could have epitope one, epitope three, and epitope four on a protein, and then antibody one would recognize epitope one, antibody two would recognize epitope two, and so forth, but antibody two would not recognize epitope one. There is one antibody for one specific epitope. How can we apply this? One of the earliest applications of using antibodies diagnostically - that is, to detect a particular protein - was in ELISA. This is still used quite frequently. 4 Copyright 2024 Biotech Primer, Inc. PROPRIETARY. DO NOT SHARE. Section 3: Enzyme-Linked Immunosorbent Assays (ELISA) Technology Enzyme-Linked Immunosorbent Assay (ELISA) ELISA stands for enzyme-linked immunosorbent assay. ELISAs are quantitative when done correctly and can tell us how much of a particular protein is present. Usually, this is a very small amount, picograms, femtograms, nanograms, so a very small amount of protein. ELISAs are performed in multi-well plates. These are plastic plates with lots of little wells in them. In each well, we put our antibodies, our antigen, and so forth. The antigen is just another term for the protein that the antibody will recognize. On the antigens, there are the epitopes, the specific part of the antigen that an antibody recognizes. Enzyme-Linked Immunosorbent Assay (ELISA) Steps When we start, we're looking here at a single well in a multi-well plate and just want to see what happens in this well. Click each step on the tabs to learn more. Layer 1 We start by binding the antibody to the bottom of the well, and you can buy plates where the well is specially designed chemically so the antibody will attach to it. This is a nonspecific interaction. Any protein will stick to the well, but we're attaching an antibody to this plate. Layer 2 We then add a patient’s blood sample, which will likely contain multiple antigens, designated here by different colors. Only the purple antigen binds to the antibody. The other antigens do not bind because they do not have the epitope that the antibody recognizes. So, the antibody has captured the antigen that we're interested in. Layer 3 At this point, though there is no label, there's no way to visualize that any of this has occurred. So, what we need to do is add something that allows us to detect this interaction between the antigen and the antibody. So, we add in a second antibody, which has a label, or a dye attached to it. In the meantime, we rinse away all the other molecules that are not antigens. 5 Copyright 2024 Biotech Primer, Inc. PROPRIETARY. DO NOT SHARE. We now add in our secondary antibody. This binds to a different epitope, usually, on the antigen, and the second antibody has a fluorescent dye or some other marker on it that allows us to visualize this. Layer 4 In this case, we've attached an enzyme. Remember, an enzyme is a protein that carries out a chemical reaction. So, we have chemically attached an enzyme to the antibody. This is called an enzyme antibody conjugate. We conjugated the enzyme to the antibody, then we add in a substrate, a chemical that the enzyme acts on, and the substrate changes color. ELISA Results The whole process here is we have our first antibody, which we've attached to the well. The first antibody captures the antigen. The second antibody then binds to the captured antigen and generates a color. The more captured antigen we have, the darker the color. That's what's reflected in the plate here on that right side of the screen. Click on the multi-well plate to learn about the differences we see in color for each well. Layer 6 The wells with the darker color have more antigens because they have bound more second antibody which has the label on it so we can see it. The wells that are colorless or virtually colorless have little or no antigen in them, so no color is generated. There's no secondary antibody binding to the antigen, and even when we add the substrate because there's no secondary antibody, there's nothing to make the color. So that's how an ELISA works. So again, the more intense the color, the more antigen is present. Instead of just looking at the color intensity with the eye, there are machines called plate readers that quantify or assign a numerical value to the intensity of the color in each well. And then you can get a printout of that, and you can analyze it. From that information, you can calculate the absolute amount of antigen that's present. Multiplexed ELISA Now in a multiplexed ELISA, instead of detecting one antigen at a time, we're going to detect multiple antigens at the same time. You can see this saves a lot of time. Instead of doing each 6 Copyright 2024 Biotech Primer, Inc. PROPRIETARY. DO NOT SHARE. thing individually, we're going to do it all at once. We're going to attach the antibody to a bead. So instead of attaching our antibody to the well, we're going to attach it to a bead. The way it's been designed, the multiplex assays can generate beads that have 100 different colors to them. By color, we mean the wavelength of light. So, each bead will interact with a different wavelength of light, which we can generate by a laser beam. We then attach an antibody to these beads to each color. So, we're going to have groups of colors. Initially, in one vial, we're going to have these pink beads, and in another vial, we'll have the blue beads. The pink beads get an antibody that recognizes one epitope. The blue beads have a different antibody attached to them that recognizes a different epitope. We can do this 100 times with 100 different antibodies, which allows us to recognize 100 different antigens simultaneously. So, we can then use multi-well plates. The next slide will show us different types of multi-well plates. These are multiples of each other. 96, 384, 1536 Well Plates We started with 96 well plates, then 384, then 1536 wells. You notice that each plate is the same size and therefore has a footprint. These fit basically in the palm of your hand, just maybe a little bit bigger depending on your hand size. But they're all the same size because each plate can fit into a plate reader, which can be purchased. If you buy a higher quality plate reader, it is configured so that it can analyze the different plate types with different numbers of wells. You program into the plate reader that it is reading a 96 well plate, 384 well plates, or 1536 well plate. But in each of those, in each well of that 96-well plate, we can put 100 different beads, which means that we can then analyze 100 different samples or 100 different antigens. The same thing can be done in each well 1536 well plate, the only thing that will change is the total volume of liquid in each well. 96, 384, 1536 Well Plates Volumes As we increase the number of wells, the size of the wells decreases, so there's less volume to deal with. That becomes a little bit problematic as we move into the 1536 well plate. It becomes a little bit more problematic because the smaller the well, the less volume we have. We must 7 Copyright 2024 Biotech Primer, Inc. PROPRIETARY. DO NOT SHARE. begin to worry about evaporation issues. As the liquid evaporates, that changes the concentration, and the beads won't be suspended in the liquid. Therefore, their ability to interact with the antigen may also be altered. So, we just need to keep the chamber that this occurs in well-humidified so that it minimizes evaporation. Again, these are specially made plates that allow us to pass light through them but also allow the reactions to occur properly within the plate. To learn about how this process has now become automated click on the “Look Closer” button, otherwise, click next to continue. Look Closer: Rapid Multiplexed Analyzers This process is now becoming automated because it is very difficult to accurately pipette into a 1536 well plate a specific volume manually. So, you now purchase robots that will pick up the samples, add them to the plate, and the robot adds the same amount of liquid into each well of the plate. They can even pick up the different samples and so forth. Therefore, essentially, all you need is a human being to be able to monitor the process, make sure the plates don't get jammed, make sure all the liquids are filled and in the proper location, and the machine will add all the liquids to the wells. These machines are large, but they save a lot of time. And they make fewer mistakes in theory than a human does. 8 Copyright 2024 Biotech Primer, Inc. PROPRIETARY. DO NOT SHARE. Section 4: Bead Immunoassay Technology Let's look at an individual bead then in terms of our multiplexed assay. Click on each tab to learn more. Layer 1 We have some sort of inert material, and this inert material is filled with a dye that can be excited by a laser to give us a particular color at a particular wavelength of light. Layer 2 Attached to that bead is an antibody, which is specific for a particular epitope on an antigen. This is our capture antibody. This is the antibody that was on the bottom of the well on the ELISA plate. We've now simply attached it to a bead. Layer 3 When we add in the antigen, the antigen binds to the antibody and captures it on the bead, it's now attached to the antibody and, by default, to the bead. The color of the bead tells us what epitope the antibody is specific for; that is, we know that if it's a blue bead, it's going to capture epitope number one. Layer 4 We still at this point just know that we have a blue bead, but we don't know if we've captured the antigen or not, we will only capture that antigen if it's present in the sample. So, we need to add our second antibody, which has our detection label on it as well. Once again, we've now completed a sandwich. Layer 5 And then we add in our substrate, and we get our color formation. We said we can do this with up to 100 different beads. What changes is the color of the bead, and then the color of the bead is going to tell us what antibody we have attached to it. 9 Copyright 2024 Biotech Primer, Inc. PROPRIETARY. DO NOT SHARE. Multiplexed Bead Assays Here we have simply two beads, a pink bead, and a blue bead, the capture antibodies are slightly different colors. These will capture different epitopes, epitope one on one protein, epitope two on a different protein. These get captured by the beads. These beads can be placed in the same well so that we can measure both proteins in the same well. Click each number chronologically to learn more. Layer 1 We’ve captured two different antigens, but we still can’t demonstrate they are captured, so we add the second antibody, which will bind to the antigen. This now has the substrate on it that gives us the color. Layer 2 Here's the tricky part. The color of the bead tells us which antibody is attached to it and that tells us which antigen we've captured. The second antibody, they all have the same substrate on them, so they give us the same color. That tells us how much of each antigen we have present. So that's what we call the analyte signal. The nice part about this is we can detect multiple samples, multiple different antigens in a very small volume. Therefore, we don't need to acquire as much fluid from the patient. Layer 3 There is a second signal as well called the discriminator signal. This signal tells us whether we're epitope one or epitope two on the antibody. We can read each bead individually by essentially using a method called flow cytometry, which sorts the beads based on color. Cell Sorter “Reads” Beads In a flow cytometric device, we have a very narrow tube, which only allows one bead at a time to pass through the tube. You see that on the right side of the screen. The tube is so narrow that only a single bead can drop through the tube. As it drops through the tube, it's illuminated by a laser, and the wavelength of light, that is the color, is detected on the other side. The bead is then counted so we know how many colors of the bead are in the sample. 10 Copyright 2024 Biotech Primer, Inc. PROPRIETARY. DO NOT SHARE. Section 5: Lateral Flow Assay Technology Home Pregnancy Test Let's look at some real-life examples of our immunoassays. We’ll start with a home pregnancy test. We're using a single antibody to detect a single protein. The home pregnancy test is a sandwich of ELISA on a strip and we're measuring the beta subunit of human chorionic gonadotropin or hCG. This is a protein that's produced in pregnant women, and it appears in their urine. We call this a lateral flow immunochromatographic assay because this is flowing in one direction. Lateral Flow Immunochromatographic Assay There are a couple of details that we need to discuss before we can illustrate the test. Click each number chronologically to learn more. Layer 1 First, we have our hCG, the protein that we're measuring. On that protein, remember, there are epitopes, and we've made antibodies to detect the epitopes on this protein. These are anti-hCG antibodies. Notice that we have two different antibodies here to capture the hCG. On one of these antibodies, we've conjugated or attached an enzyme. Enzymes, again, carry out chemical reactions and the enzyme will interact with the dye molecule to create a color that we can observe. We then need to be able to detect this process. So, we have a second antibody to detect the antibody that has the dye molecule on it. These antibodies were originally made in mice, but this is all packaged into the home pregnancy test kit. Layer 2 The way this works is if you think you're pregnant, you obtain a urine sample, you dip the test strip in the urine sample, and by capillary action, just like a Bounty paper towel sucking up a liquid spill, the urine moves up the test strip. Within the urine is our hCG protein, which is then recognized by the initial antibody, which is in the lower part of the strip. This antibody captures the hCG to form an antibody-hCG complex. 11 Copyright 2024 Biotech Primer, Inc. PROPRIETARY. DO NOT SHARE. Layer 3 After it's captured, this antibody complex then continues up this test step, carrying the hCG and the antibody. Our second antibody then recognizes the initial antibody and the hCG complex, and then we have our chemical reaction where it reacts with a dye molecule. If hCG is present, this turns red. Layer 4 But we also want to just make sure that this works. Therefore, we have what's called positive control. We also have dye molecules and capture antibodies up there, up at a second strip, a second panel, which will always turn color no matter what. This is called control. This just ensures that the antibody is working properly and that we're able to detect color. Pregnancy Test Assay Results If you have a positive test, you'll see both panels appear, the color change will appear on both panels. But if you have a negative test, only the control zone will change color. This shows us that indeed, this test is working. If there was no color appearing in a control zone, then it tells us that your test kit is defective and that the test is inconclusive because we don't know if it worked or not. So, we always like to include what's called a positive control, which is a sample that we have created that gives us a positive result just to know if the test is working correctly. In the positive test, we have the actual patient sample reacting, and we have a positive control that we have artificially included to see if things are working or not. In the negative test, the patient sample did not have hCG, so the bottom panel didn’t change color, but the top panel changed red, indicating that everything is working properly. In some cases, we may need to achieve some measure of separation of proteins to detect them, that is we have mixtures of proteins, mixtures of biomarkers present, and one protein may mask our ability to detect another protein. If this is the case then we first need to separate our proteins, which we will discuss on the next screen. 12 Copyright 2024 Biotech Primer, Inc. PROPRIETARY. DO NOT SHARE. Section 6: Chromatography Technology Protein Chromatography If we have a mixture of proteins, then we need to separate our proteins from one another. The process that we frequently use to separate one protein from another is called protein chromatography. In protein chromatography, we separate proteins from one another based on their physio-chemical characteristics, and all that means is their physical and chemical characteristics. And really, all that means is their physical and chemical characteristics. The physical and chemical characteristics of a protein are things like charge: does the protein have a positive or negative charge? structure, meaning what shape the protein has, and based on that shape, what will the protein bind to. And finally, size. Are the proteins large, small, or intermediate size? Click the button to learn more about the types of chromatography. Chromatography Types We name the type of chromatography according to its physio-chemical characteristic. If it's based on charge, we call it ion exchange chromatography or IEC because ions are charged particles. If it's based on structure or the ability to recognize another molecule, we say that the protein has an affinity for another molecule. We call this affinity chromatography. If it’s based on size, we call it size exclusion because larger proteins get excluded from the chromatography matrix and pass right through. Protein Chromatography Steps Let's look at the basic steps in column chromatography. Click each number chronologically to learn more. Layer 1 Let’s start with a column. A column is just a cylinder that's capped at both ends. Inside the pipe, we put the chromatography matrix. The matrix is going to be based on what we're using to separate our proteins. So, if it's an ion exchange matrix, the matrix will either be positively charged, or it will be negatively charged. It will be one or the other. If it's affinity chromatography, 13 Copyright 2024 Biotech Primer, Inc. PROPRIETARY. DO NOT SHARE. the matrix will have a particular molecule attached to it that the protein we want to capture recognizes. If it's size exclusion, it will have beads with holes or pores in it. Layer 2 We then load the protein mixture onto the column. The column matrix is sometimes called the stationary phase because it just sits there in the column. It's stationary, it doesn't move. Our protein mixture is loaded in and is called the mobile phase. This is a liquid, some type of buffer usually, and the liquid moves over the column. As it moves over the column, it carries our protein mixture with it. Depending on the characteristics of the proteins, they will interact with the column differently, some will move through quickly, some will move through at an intermediate speed, and some will move through slowly. So over time, the molecules will separate. Layer 3 In this example, if we're talking about ion exchange, the green protein that's left behind is positively charged and is sticking to a negatively charged matrix. The other proteins that move through quickly either had the same negative charge as the matrix or had no charge at all. Therefore, they were not retained. As the proteins move through the column and come out at the bottom of it, we call this elution. The proteins are eluted. Sometimes we need to change the composition of the mobile phase, we need to change the buffer to achieve elution, to make the proteins come off the column. We can do this by either changing the pH or more frequently, changing the concentration of sodium chloride in the buffer. This change causes the protein to come off the column and elute. Layer 4 We detect the elution using a detector. Frequently, we use what's called, a UV or ultraviolet detector because proteins absorb light at 280 nanometers. That's simply a wavelength of light in the ultraviolet region of the spectrum of light. As the proteins elute from the column, they pass through to the detector, and light of wavelength 280 nanometers shines on the protein. 14 Copyright 2024 Biotech Primer, Inc. PROPRIETARY. DO NOT SHARE. Layer 5 If there is, in fact, a protein present, it's detected and it shows up as what we call a peak, the absorbance value increases every time a protein comes off. Here we see two peaks, which means two proteins have come off. The size of the peak, and the height of the peak, correspond to how much protein is present. So, the higher the peak, the more protein is present. This can be quantitated. If you look at these peaks and use your imagination a little bit, you'll see that the peaks resemble a triangle. We can calculate the area under the peak, the area of a triangle as one-half to base times the height. The greater the area under the peak, the more material is present. That allows us then to also quantitate how much protein came off each column. The time it takes the protein to come off is another parameter known as retention time and taken together with the size of the peak, this is called an elution profile. If we do things correctly, for the same type of sample on the same proteins, we should always get the same elusion profile. Layer 6 We can collect each eluent and each protein in different fractions. We use a different tube for each protein. Every time we see a peak, we use a new tube to collect each protein. Ion Exchange Chromatography Now we're going to examine each of the different types of chromatography. We'll start with ion exchange chromatography. Click each number chronologically to learn more. Layer 1 Here, our chromatography matrix is either positively charged or negatively charged. The beads are one charge or the other, not both, but either positive or negatively charged. We call this anion exchange or cation exchange. Anion just means negatively charged; cation means positively charged. 15 Copyright 2024 Biotech Primer, Inc. PROPRIETARY. DO NOT SHARE. Layer 2 The matrix has a charge to it. Proteins that have the same charge as the matrix are repelled, positive repels positive, and negative repels negative. Proteins that are positively charged stick to the matrix. Proteins that have no charge or are neutrally charged pass through the column. We've color-coded our proteins here according to their charge. Here, we have a positively charged matrix. If the chromatography matrix is positively charged, what will stick to it? Let’s find out. Layer 3 Let’s load our protein onto the matrix then our mixture of proteins, the neutral charge has no charge, so it won't stick to it, it moves right through. The positively charged protein is repelled by the positively charged matrix. It also passes right through, but our negatively charged protein is retained because we have this plus-minus interaction. So, we've achieved a separation here. Layer 4 Positive and neutrals flow right through the eluate immediately. The negatively charged proteins are retained. To take the protein off the column, add sodium chloride. The sodium chloride dissolves into positively charged sodium ions and negatively charged chloride ions; the negative chloride will displace the negative protein at the positive binding site on the column. If we add a high enough concentration of sodium chloride, it will out-compete or it will win, if you will, and displace our protein of the column. And so now it can be eluted. Therefore, we have achieved our separation. Affinity Chromatography In affinity chromatography, we're separating based on a particular shape of the protein, that is the ability of the protein to recognize and bind a specific molecule. Click each number chronologically to learn more. Layer 1 Our matrix in this case is going to have attached to a molecule that can be recognized by the protein we want to capture. If you look at our illustration here, we see that some of the proteins have a unique sort of star-shaped to them, and we've also attached that star to our affinity matrix. Layer 2 16 Copyright 2024 Biotech Primer, Inc. PROPRIETARY. DO NOT SHARE. As we load our protein sample here, any protein without the star shape will pass right through the column. Any proteins that have that structure will have an affinity for the ligand, for the molecule that's attached to our matrix, and it will bind. So, once again, proteins without that shape pass right through the elute immediately, our other proteins that do have the affinity for our molecule that's attached to the matrix, stick, and bind to it. Layer 3 To elute, we just add a free ligand, and the free ligand displaces, and competes with the ligand on the column, and now, our protein is displaced. Once again, we've achieved a separation. Frequently, people say that affinity chromatography can achieve a one-step purification. That's not always true, but a lot of times it is. This makes it very efficient, but because we're essentially designing a unique column for each different protein that we want to separate, this can be very expensive. The trade-off is we have an initial upfront cost, but the efficiency of separation, and the fact that we may only need to take a single step, in some cases makes up for that initial cost. Size Exclusion Chromatography In size exclusion chromatography, we are separating proteins based on size. Click each number chronologically to learn more. Layer 1 In this case, the chromatography matrix has few pores or channels in it. What happens is the smaller proteins fit inside the pore or that channel, so they must move around in there, and that slows them down, whereas the larger proteins cannot fit in the pore - they are excluded. They go outside the pores, and they just run in between the beads and elute immediately. For intermediate-size proteins, some just barely fit in the pore and are retained, and some just pass right through. 17 Copyright 2024 Biotech Primer, Inc. PROPRIETARY. DO NOT SHARE. Layer 2 The large proteins elute or pass through the column almost instantly. The smaller proteins get trapped inside the channels and they are retained to take longer to go through. So over time, we get a separation. So here a large protein just passed right over that column because it couldn't fit into the channel. The small protein enters the pores, and it takes a while to move through the channel before it comes out. The intermediate size proteins, some just barely fit in the pore, and they're retained, and some pass right through. We call this a resolution issue. Resolution refers to how easily the protein separates from one another; how cleanly they separate from one another. In this case, the green protein would be incompletely resolved because some of it would elute with our large protein and some of it would elute with our smaller protein. What you would see when you saw the elution profile is the peaks may be blurred together. So, this is a way to tell how effective your chromatographic separation or your resolution is. Layer 3 You need to design a chromatographic process that gives you a sharp resolution that separates one protein from another. Chromatography: Monitoring Diabetes What are the practical uses of protein chromatography? It turns out there's a particular protein, HbA1c, which is a biomarker for diabetes. Measuring levels of glycosylated hemoglobin, HbA1c is a key method used in diabetes diagnosis and monitoring. We can separate HbA1c from other blood proteins using ion exchange column chromatography. It turns out that our glycosylated hemoglobin is more negatively charged than normal unglycosylated hemoglobin. If we put the two proteins, HbA1c, and normal nonglycosylated hemoglobin, on an ion exchange column, they will elute, they will separate from one another, and elute at different times. To learn how this test can be done using an automated system click the “look closer” button, otherwise click next to continue. 18 Copyright 2024 Biotech Primer, Inc. PROPRIETARY. DO NOT SHARE. Look Closer: Automated A1C Test You can now do this process using an automated device where you just pop in your ion exchange column and load your sample on. You can see on the left side of our device, there are buffers to carry out the elution, the UV detector is in there, and it will detect the glycosylated from the unglycosylated hemoglobin based on the retention time, that is how long it takes the protein to elute from the column. And so, by looking at that, you can see if you have HbA1c or not. How Diagnostics Work: Protein-Based Diagnostics Summary In summary, in this course, we learned that: ● Antibody-based diagnostics rely on the specificity of antibody-antigen interactions to detect the presence of a specific protein associated with a disease or condition. ● Enzyme-linked immunosorbent assays (ELISA) allow clinicians to visualize the interaction by using a secondary antibody with a fluorescent label. ● ELISAs can be adapted for high throughput or home use. ● Column chromatography is a technique used to separate proteins based on their differing chemical or physical properties. ● Column chromatography can be used to detect variants of a protein associated with a disease. 19 Copyright 2024 Biotech Primer, Inc.