Engineering Protein-Based Therapeutics PDF
Document Details
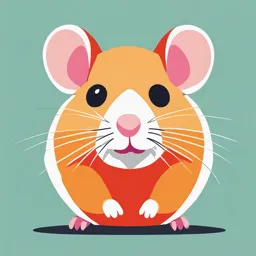
Uploaded by LucrativeYtterbium
Sasha B. Ebrahimi and Devleena Samanta
Tags
Related
- Protein-Based Diagnostics PDF
- Proteins PDF - Biochemistry Notes
- Beliefs Underlying Older Adults' Intention to Consume Plant-Based Protein Foods PDF
- Cardiac Amyloidosis Imaging, Part 1: Amyloidosis Etiology and Image Acquisition PDF
- Lectures 13-17 Study Guides PDF
- Bio Inorganic Ferritin, Hemoglobin, and Hemerythrin PDF
Summary
This review article explores the strategies used in the engineering of protein-based therapeutics. It highlights the importance of chemical and structural modification in creating effective medications. The article also underscores the role of protein-based therapeutics in modern medicine, their advantages over traditional small-molecule drugs, and current challenges.
Full Transcript
Review article https://doi.org/10.1038/s41467-023-38039-x Engineering protein-based therapeutics through structural and chemical design Received: 3 November 2022 Sasha B. Ebrahimi 1 & Devleena Samanta 2 Accepted: 5 April 2023 1234567890():,; 1234567890():,; Check for updates Protein-based therapeuti...
Review article https://doi.org/10.1038/s41467-023-38039-x Engineering protein-based therapeutics through structural and chemical design Received: 3 November 2022 Sasha B. Ebrahimi 1 & Devleena Samanta 2 Accepted: 5 April 2023 1234567890():,; 1234567890():,; Check for updates Protein-based therapeutics have led to new paradigms in disease treatment. Projected to be half of the top ten selling drugs in 2023, proteins have emerged as rivaling and, in some cases, superior alternatives to historically used small molecule-based medicines. This review chronicles both well-established and emerging design strategies that have enabled this paradigm shift by transforming protein-based structures that are often prone to denaturation, degradation, and aggregation in vitro and in vivo into highly effective therapeutics. In particular, we discuss strategies for creating structures with increased affinity and targetability, enhanced in vivo stability and pharmacokinetics, improved cell permeability, and reduced amounts of undesired immunogenicity. In 1982, Humulin became the first FDA-approved, recombinant, protein-based therapeutic1. Today, protein-based drugs constitute a market approaching ~$400 billion with hundreds of candidates approved and in clinical trials2. Here, we chronicle the chemical design strategies that have transformed the field of medicine from one that has been historically dominated by small molecule pharmaceuticals to one where proteins have emerged as comparable or superior rivals. Proteins represent the most versatile class of biomolecules, acting as catalysts, signaling molecules, molecular and ion transporters, scaffolds for maintaining cellular and tissue integrity, receptors, and more3. Therefore, as medicines, proteins can be used to serve any of these roles (Box 1) and offer several advantages over small molecule drugs. Proteins are less likely to cause side effects by interfering with normal biological processes because they have evolved to play highly specific roles. Typically, protein therapeutics show high potency and can also execute more complex functions owing to their intricate three-dimensional structures. Over the last ~150 years, fundamental advances have been made towards harnessing the power of proteins to create new medicines (Fig. 1). The development of an antibody-based treatment for diphtheria in 1891 was a major milestone and was awarded the first Nobel Prize in medicine4. The antibody was extracted from the serum of horses that had been challenged with an attenuated form of diphtheria-causing bacteria. Two decades later, the extraction of insulin from porcine pancreas for the treatment of diabetes mellitus marked another significant advance—the use of an exogenous protein to treat an endogenous deficiency5,6. However, two tons of pig pancreas were required to produce eight ounces of protein7. Assuming 72 million patients (a close approximation for the number of diabetics in the world requiring insulin) and the need for 0.02 ounces of insulin per year for the average patient, this would necessitate ~150 million pigs/ yr, making it challenging to produce the drug at appropriate scale8,9. Moreover, the use of proteins extracted from animals could elicit an immune response in patients and lead to potential exposure to animal diseases. A breakthrough moment came in 1982 when recombinant DNA technology was used to produce insulin in a bacterial host (E. Coli)10. The successful use of recombinant DNA technology helped to circumvent challenges with both scale-up and immunogenicity of animal-derived proteins. Considering that, in principle, one could express any protein for which its associated gene is known, a viable competitor to the workhorse of medicine at the time – small molecules – had truly emerged. Still, there were intrinsic challenges that needed to be solved due to the inherent susceptibility of proteins to aggregation, degradation, denaturation, and concomitant loss of activity11. Moreover, untimely clearance from the body, non-specific distribution, immunogenicity, and toxicity also posed relevant concerns12. Advances in rational design and ability to deliberately introduce chemical and structural modifications have driven a paradigm shift in how these properties can be tuned13–15. In the following sections, we delineate the key considerations that drive the chemical design of protein-based therapeutics and describe the various design strategies that have led to 1 Drug Product Development—Steriles, GlaxoSmithKline, Collegeville, PA 19426, USA. 2Department of Chemistry, The University of Texas at Austin, Austin, TX e-mail: [email protected]; [email protected] 78712, USA. Nature Communications | (2023)14:2411 1 Review article https://doi.org/10.1038/s41467-023-38039-x BOX 1 Common classes of protein-based therapeutics Antibodies Antibodies are produced by B lymphocyte cells of the immune system in response to foreign objects, such as invading pathogens. They function by binding to specific molecules on the pathogen’s surface (antigens) and inactivating the invader. As therapeutics, antibodies are predominantly used to bind target antigens which can then block specific signaling pathways (e.g. nivolmab) or induce cell death (e.g. binutuzumab). Antibodies can also serve as vehicles for the targeted delivery of potent cytotoxic drugs to diseased cells (e.g. antibody-drug conjugates such as trastuzumab emtansine). Monoclonal antibodies (mAbs), generated using clones of a unique lymphocyte cell, constitute the most prevalent type of antibody-based therapeutics. Structurally, antibodies are Y-shaped, comprising two antigen-binding fragments (Fab) and a crystallizable fragment (Fc). Enzymes Enzymes play a key role in the human body by catalyzing a wide variety of biochemical reactions. As therapeutics, they can be delivered to replace a deficient or absent enzyme in so called enzyme replacement therapy. In other cases, they can be used as agents to catalyze the degradation, cleavage, or chemical modification of therapeutically relevant targets. Examples of enzyme-based drugs include PEG-asparaginase, sacrosidase, pegvaliase, and laronidase. Coagulation factors Coagulation factors are naturally occurring proteins that play an essential role in the blood clotting process. Consequently, as therapeutics, these proteins can be plasma-derived or produced recombinantly and administered to patients with a deficiency in native levels. Coagulation factor-based medicines are used for various bleeding-related indications, including hemophilia A and B (e.g. eptacog alfa). Protein hormones Protein hormones constitute a class of molecules that are secreted by endocrine glands. They act as chemical messengers by binding to receptors which then triggers a signaling cascade and leads to a physiological response. Insulin represents a classic example. After a meal, insulin facilitates glucose removal from the bloodstream by binding to its cell-surface receptor. This initiates an intracellular cascade that results in translocation of glucose transporters to the surface and subsequent uptake of glucose into cells. Other therapeutically-relevant protein hormones include erythropoietin and gonadotropin. Cytokines Cytokines comprise a broad group of proteins (encompassing molecules such as interleukins, interferons, and colony-stimulating factors) that mediate cell-to-cell communication during immune responses. This contrasts with protein hormones, which largely regulate the endocrine system (vide supra). Therapeutic cytokines can be used as immunomodulatory agents for a variety of indications, including multiple sclerosis (interferon β−1b) and hairy cell leukemia (interferon α−2b). ~350 approved drug products (Supplementary Tables S1–S3). We end with an outlook on areas that need further development to realize the full potential of these molecules. We particularly focus on deliberate structural and chemical modifications that are made directly to the protein structure as opposed to strategies such as directed evolution and protein encapsulation, or those focusing on tuning protein formulations. We also exclude vaccines. Key considerations driving the chemical design of protein-based therapeutics To function as effective therapeutics, proteins must have certain characteristics (Fig. 2). These desirable attributes vary from protein to protein based on the application of interest. A key consideration is the stability of the proteins both under storage conditions and in vivo. Many proteins are susceptible to aggregation, degradation (e.g., via deamidation/oxidation in vitro, through proteases in vivo, etc.), and denaturation which can significantly reduce efficacy16,17. Some proteins are sensitive to moderate changes in temperature, which is an added concern for their transport and storage in different locations11. Moreover, the residues at the surface of certain proteins can display favorable interactions with container surfaces, resulting in adsorption and reducing the concentration of the active ingredient available for therapeutic action18. Therefore, several structural modifications (e.g., site-specific mutations19 and PEGylation20) are aimed at improving the solubility and stability of proteins. Protein-based therapeutics must also exhibit appropriate pharmacokinetics and pharmacodynamics for optimal function. For example, a protein that is rapidly cleared from the body is not suitable for applications that require sustained action16. On the other hand, a protein that circulates for a very long time may cause side effects. Nature Communications | (2023)14:2411 Similarly, protein drugs must not interfere with the body’s natural functions or elicit unwanted immune responses. To address these challenges, various strategies including PEGylation20, glycosylation21, lipidation22, and protein fusion3 have been developed. Another important consideration in the development of proteinbased medicines is targetability. The ability to target drugs to specific tissues (e.g., cancer cells) or organs (e.g., brain) is highly desirable both from the standpoint of lowering therapeutic dose as well as reducing side effects. For example, antibody-drug conjugates can be used to direct the drug to cells that express a specific receptor as the antigen. Many protein-based structures are prone to sequestration in the liver, kidney, and spleen23. Strategies wherein targeting moieties are incorporated to promote distribution outside of these organs are an active area of investigation24. Recently, it has been shown that proteins covalently conjugated to multiple copies of the transferrin aptamer show preferential accumulation in the brain relative to native proteins25. The majority of current protein-based pharmaceuticals have extracellular targets. The relatively large sizes compared to smallmolecule drugs and heterogeneous surface charges render most proteins impermeable to the cell-membrane. Intracellular delivery is highly sought after as the ability to intercept deleterious intracellular processes can lead to highly effective medicines. Emerging chemical strategies such as appending cell-penetrating peptides26, supercharging27, and dense DNA grafting28 have shown promising results in this regard. While chemical modifications to protein structures can impart several advantageous properties, they can also alter the activity or potency of the drugs20. Typically, high activity or potency is a desirable trait as it can lower the therapeutic dose necessary. The central 2 Review article https://doi.org/10.1038/s41467-023-38039-x 1990 FDA approval of first PEGylated protein, pegademase bovine 1991 FDA approval of first enzyme replacement therapy, alglucerase 1997 FDA approval of first humanized mAb, daclizumab 1922 insulin purified from bovine and porcine pancreas and used as a life-saving daily injection for patients with Type I diabetes 1890s 1920s FDA approval of first mAb for cancer treatment, rituximab 2013 FDA approval of first glycoengineered protein, obinutuzumab 2014 FDA approval of first bispecific antibody, blinatumomab FDA approval of first albumin fusion protein therapeutic, albiglutide 1998 2019 FDA approval of first protein fusion / Fc fusion, etanercept First de novo protein therapeutic, NL-201 1980s 1990s 2000s 2010s 1891 1982 2000 discovery and use of diphtheria antitoxin FDA approval of first recombinant protein therapeutic, insulin (Humulin) FDA approval of first ADC, gemtuzumab ozogamicin 1986 FDA approval of first sitespecifically PEGylated protein, pegfilgrastim 2020s 2021 FDA approval of 100th unique monoclonal antibody 2002 FDA approval of first mAb, muromonab-CD3 2005 FDA approval of first lipidated protein therapeutic, insulin detemir Fig. 1 | A timeline of significant advances in the development of protein-based therapeutics. challenge in designing protein-based therapeutics is to choose a strategy that leads to the gain of a certain function without causing the loss of another. Table 1 summarizes the ability of established and emerging chemical design strategies (Fig. 3) to achieve this balance. Established chemical design strategies Site-specific mutagenesis. The use of site-specific mutagenesis to introduce amino acid point mutations has been a widely employed method to confer enhanced properties to protein-based therapeutics29. A classic example of this has been in the development of insulin variants with different kinetics of action. (Supplementary Table S1). For instance, the substitution of asparagine by glycine at amino acid 21 of the α chain and the addition of 2 arginines to the β chain gives rise to insulin glargine, a long-acting variant with duration of action up to 24 h30. These amino acid modifications Nature Communications | (2023)14:2411 increase the isolectric point (pI) of the structure towards physiological pH, resulting in precipitation upon injection and therefore a decrease in absorption rate29. In other cases, substitutions can be made that decrease self-association and increase the rate of absorption30. Insulin glulisine, for example, has a modified amino acid sequence wherein β chain asparagine (position 3) and lysine (position 29) are exchanged with lysine and glutamic acid, respectively31. The subsequent decrease in pI from 5.5 (native insulin) to 5.1 promotes increased solubility with less propensity for hexamer formation, leading to fastacting effect. Targeted mutations can also be used for tuning protein stability in solution, resulting in more robust formulations less prone to unwanted aggregation. A well-established modification involves the substitution of free cysteines with other amino acids such as serine to prevent the formation of non-native disulfide bonds or oxidation of 3 Review article https://doi.org/10.1038/s41467-023-38039-x Stability Desirable Pharmacokinetics [Drug] in blood Faster-acting Longer-acting Targetability Time Denaturation Degradation Aggregation Intracellular delivery Desirable Pharmacodynamics Response High potency Potency difference Low potency Drug dose No side effect No unwanted immune response Fig. 2 | Desirable characteristics of protein-based therapeutics. These include high stability (top left), ability to enter cells (bottom left), and appropriate pharmacokinetics (top right) and pharmacodynamics (bottom right). cysteine residues leading to thiol adducts or cysteic acid32. This strategy has been employed in various FDA-approved protein therapeutics, including aldesleukin (Proleukin), interferon β1b (Betaseron), and pegfilgrastim (Neulasta). To explore a more general strategy, Trout et al. developed spatial aggregation propensity (SAP), a molecular dynamics-based simulation technique for identifying key regions in proteins that drive aggregation33. Based on this information, they were able to make specific mutations that enhanced stability compared to the native protein. Validated point mutations are also commonly used to control IgGbased antibody behavior in vivo. For instance, circulation half-life can be tuned by introducing substitutions into the Fc region that change the nature of binding interactions with the neonatal Fc receptor (FcRn), a receptor present in the endosomes of a variety of cell types. Specifically, promoting binding at endosomal pH (